Abstract
Bio-refinery processes require use of the most suitable lignocellulosic biomass for enzymatic saccharification and microbial fermentation. Glucose yield from biomass solid fractions obtained after dilute sulfuric acid (1%) pretreatment (at 180 °C) was investigated using 14, 8, and 16 varieties of rice, wheat, and sorghum, respectively. Biomass solid fractions of each crop showed similar cellulose content. However, glucose yield after enzymatic hydrolysis (cellulase loading at 6.6 filter paper unit/g-biomass) was different among the varieties of each crop, indicating genotypic differences for rice, wheat, and sorghum. Nuclear magnetic resonance method revealed that the high residual level of lignin aromatic regions decreased glucose yield from solid fraction of sorghum.
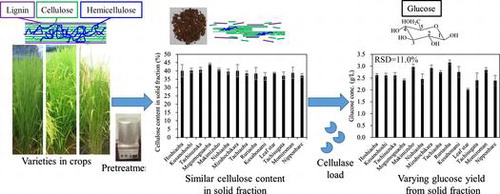
The use of lignocellulosic biomass to produce biofuels will help decrease our dependence on fossil fuel resources and reduce net CO2 emissions. Inedible biomass has recently drawn attention for this purpose as it does not compete directly with global food production, in contrast with edible biomasses such as maize and barley grains. Lignocellulosic biomass is mainly composed of cell wall components such as cellulose, hemicellulose, and lignin.Citation1) These polysaccharides must be hydrolyzed to fermentable sugars to be utilized by microorganisms.Citation2) However, cellulose and hemicellulose comprise densely packed layers of lignin, and cellulose forms a highly crystalline structure. Consequently, both polysaccharides are resistant to enzymatic hydrolysisCitation3) and thus require pretreatment prior to enzymatic hydrolysis to efficiently provide fermentable sugars. The pretreated lignocellulosic biomass is separated into solid and liquid fractions. The solid fraction consists mainly of cellulose and lignin and is used as a sugar source for fermentation.Citation4)
A high sugar yield from the solid fraction is critical for obtaining high concentrations of bio-products during subsequent microbial fermentation and for decreasing purification cost. Thus, plant varieties that release high amounts of glucose should be used in bio-refinery processes. Previous studies reported variation in sugar yields between plant species (wheat straw vs. corn stover, and wheat straw vs. water hyacinth).Citation5,6) In addition, transgenic approaches that change the lignin composition of the plant cell wall result in different enzymatic hydrolysis efficiencies.Citation7) Moreover, the biomass composition of different varieties of the same plant species differ,Citation8) suggesting that the yield of sugar obtained from the solid fraction after pretreatment and enzymatic hydrolysis will differ between varieties of a crop species such as rice, wheat, or sorghum.
Dilute acid pretreatment is a generally inexpensive and convenient process, and is effective for a broad range of lignocellulosic biomass types (herbaceous, softwood, and hardwood).Citation9,10) The temperature used during pretreatment affects subsequent enzymatic hydrolysis efficiency.Citation11) Thus, the effect of pretreatment temperature, and the enzymatic load following pretreatment, was investigated to quantify the glucose yield from the solid fraction residue from 14 rice (Oryza sativa L.), 8 wheat (Triticum aestivum), and 16 sorghum (Sorghum bicolor) varieties in order to clarify genotypic differences affecting sugar yield. In addition, the factor affecting different sugar yield was in depth investigated by a solution state two-dimensional (2D) 1H-13C hetero-nuclear single quantum coherence (HSQC) nuclear magnetic resonance (NMR) method to resolve lignin components. The results described herein will help identify suitable varieties of rice, wheat, and sorghum for microbial conversion to bio-based products.
Materials and methods
Plant materials
The 14 rice straw varieties examined in this study were grown in 2012 in an experimental field located at the Food Resources Education and Research Center, Kobe University (Kasai City, Hyogo Prefecture, Japan). The wheat varieties were grown in 2012–2013 in a greenhouse at Kobe University (Kobe City, Hyogo Prefecture, Japan). Sorghum varieties were grown in 2013 at Togo Field of the Science and Education Center of Nagoya University (Togo, Aichi, Japan). The sorghum whole plants were harvested at 45 to 50 days after flowering and allowed to dry in the field for 3 days. The grains were removed and the straw was powdered using a blender (WB-1; TGK, Tokyo, Japan) fitted with a 2-mm screen.
Sugar analysis
Sugar content was analyzed by mixing each sample (1.5 μL) with 1.5 μL of 0.1% (w/w) Ribitol, followed by drying in a vacuum concentrator (7810010; Labconco, Kansas City, MO, USA). The dried residue was dissolved in 100 μL of 20 mg/mL methoxyamine hydrochloride in pyridine and incubated at 30 °C for 90 min, then 50 μL of N-methyl-N-trimethylsilyltrifluoroacetamide was added and the sample was incubated at 37 °C for 30 min. A 10-μL aliquot of this sample solution was subjected to gas chromatography-mass spectrometry (GC-MS) on a GC-MS-2010 Plus system (Shimadzu, Kyoto, Japan) using the following conditions: column, Agilent CP-Sil 8CB-MS (30 m × 0.25 mm); carrier gas, helium; injection temperature, 230 °C; oven temperature, 80 °C at t = 0 to 2 min, then to 330 °C at 15 °C/min.
Dilute acid pretreatment
Dilute acid pretreatment was performed using an HHE-19G-U laboratory-scale thermostirrer (Koike Precision Instruments, Kanagawa, Japan). The reactor had a total volume of 100 mL and was equipped with an electric heater and magnetic agitator. The optimal temperature and agitation speed for pretreatment were determined according to a previous report.Citation12) Biomass powder (6 g) was suspended in 80 mL of 1% (v/v) sulfuric acid and incubated at 130 or 180 °C for 45 min with agitation at 200 rpm. The resulting liquid hydrolysate and solid fraction were separated by filtration. The solid fraction was washed with deionized water, neutralized to pH 7.0, and then dried. The weight of the residue was determined using an XS105DU electronic balance (Mettler Toledo, Greifensee, Switzerland).
Enzymatic saccharification
Enzymatic saccharification of the solid fraction (10% dry weight) was performed by adding 0.3 M citrate buffer (pH 4.8) and cellulase (Cellic CTec2, Novozyme, Bagsvaerd, Denmark) at a concentration of 6.6 or 66 filter paper unit (FPU)/g-dry biomass. Tetracycline (40 μg/mL) and cycloheximide (30 μg/mL) were added to prevent the growth of potential microbial contaminants. The reaction mixture was incubated at 50 °C for 72 h in a PPS-2000 ChemiStation (Tokyo Rikakikai, Tokyo, Japan) with agitation at 120 rpm. Enzymatic saccharification was stopped by rapid chilling on ice, followed by centrifugation at 21,880 × g for 10 min at 4 °C. The sugars in the supernatant were analyzed as described above.
2D NMR spectroscopy
Solubilized lignocelluloses were prepared in a manner similar to previous studies [Citation13]. Briefly, dried cell-wall material was further ground in a Pulverisette 5 ball mill (Fritsch GmbH, Idar-Oberstein, Germany) to yield 300 mg of stock powder. The ball-milled powder (30 mg) was mixed with 600 μL of DMSO-d6:pyridine-d5 (4:1), heated at 50 °C for 30 min in a Thermomixer Comfort (Eppendorf AG, Hamburg, Germany), then centrifuged at 20,380×g for 5 min. The supernatant was transferred to 5-mm ϕ NMR tubes and subjected to NMR analysis. NMR spectra were recorded on an AvanceIII HD-600 instrument (Bruker, Billerica, MA, USA) equipped with a 5-mm TXI-cryo probe operated at 600 MHz for 1H and 125 MHz for 13C analysis. The temperature of all NMR samples was maintained at 313 K. Chemical shifts were referenced to the methyl group of DMSO-d6 at 13C = 40.03 ppm and 1H = 2.582 ppm. Solution-state 2D 1H-13C HSQC-NMR spectra were collected using echo/antiecho gradient selection (the ‘hsqcetgp’ pulse program in the Bruker library). Peak intensity of each regions of interest (ROI) was calculated using tetramethylsilane as internal standard. ROI were identified based on previously assigned chemical shifts.Citation13,14
Results and discussion
Similar cellulose contents in solid fraction among varieties of each crop
Glucose is generated from cellulose in the solid fraction and thus the cellulose (glucan) content of the solid fractions obtained from rice, wheat, and sorghum were analyzed (Fig. ) following pretreatment of these lignocellulosic biomasses at 180 °C using dilute acid. The solid fraction yields were 49.4 ± 9.1%, 56.8 ± 9.1%, and 46.2 ± 9.1%, for rice, wheat, and sorghum, respectively. The cellulose content of the solid fractions did not differ significantly between varieties of a single species. Nonetheless, we investigated whether the amount of sugar obtained from the solid fraction differs among varieties.
Pretreatment and saccharification conditions to differentiate glucose yield of rice solid fractions
The pretreatment temperature and the amount of enzyme loading are important factors to affect the glucose yield that can be obtained from solid fraction. Thus, two pretreatment temperatures (130 or 180 °C) and enzymatic loads (6.6 or 66 FPU) were tested on straw from 14 rice varieties (a total of four conditions) (Fig. ). Relative standard deviation (RSD) was used as an indicator of varietal difference in glucose concentration obtained after enzymatic hydrolysis of the solid fraction. The RSD value was highest (RSD = 11.0%) when straw from 14 rice varieties was pretreated at 180 °C followed by enzymatic hydrolysis using 6.6 FPU of enzyme. The highest glucose level was obtained from the rice variety Ruriaoba and the lowest level was obtained from the variety Leaf Star. Interestingly, the significantly different glucose levels between Ruriaoba and Leaf Star resulted despite the lack of significant difference in cellulose content in solid fraction between these two rice varieties (Fig. ). A pretreatment temperature of 180 °C was previously often used for dilute acid pretreatmentCitation12,15) and 10 FPU of cellulase was used for bio-ethanol production from the solid fraction of rice using engineered Saccharomyces cerevisiae displaying cell-surface cellulase.Citation16) Therefore, pretreatment at 180 °C followed by enzymatic hydrolysis using 6.6 FPU cellulase was previously used to prepare lignocellulosic biomasses for microbial fermentation.Citation16) The present results suggested the importance of choosing a suitable rice variety to obtain higher amounts of bio-products from glucose.
Fig. 2. Effect of pretreatment temperature and cellulase load on glucose yield from solid fractions (10% wt/vol) obtained from 14 rice varieties. (A) 130 °C, 66 FPU/g-biomass, (B) 180 °C, 66 FPU/g-biomass, (C) 130 °C, 6.6 FPU/g-biomass, and (D) 180 °C, 6.6 FPU/g-biomass. The mean ± SD of triplicate measurements is shown.
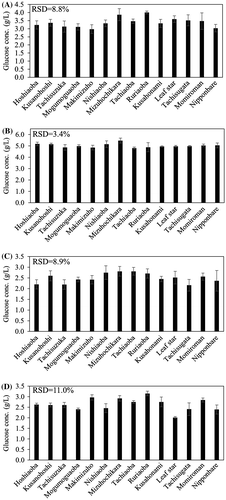
Varying glucose yield from solid fractions of wheat and sorghum
The above conditions (pretreatment temperature of 180 °C and an enzymatic load of 6.6 FPU) were used to obtain glucose from the solid fractions obtained from straw from various wheat and sorghum varieties (Fig. ). Consistent with the results obtained using different rice varieties, wheat and sorghum similarly showed varietal differences in glucose yield from solid fractions (RSD = 12.1 and 21.6%, respectively). In wheat, the highest glucose yield was obtained from the Mironovskaya 808 and the lowest from the Timstein solid fractions, despite the lack of significant difference in cellulose content between the two solid fractions. Similarly, the highest glucose content was obtained from the bmr-6 sorghum and the lowest from the Icr-38 solid fractions, again despite the lack of significant difference between their cellulose contents. Thus, the glucose yield is independent of the cellulose content of a rice, wheat, or sorghum solid fraction.
2D-NMR analysis of solid fractions
We investigated this interesting dichotomy further. The average ratios of the glucose yield to the maximum glucose yield were 47.2, 43.3, and 34.6% in rice, wheat, and sorghum, respectively, indicating that enzymatic hydrolysis of the solid fraction was being prevented, probably by lignin.Citation17) Lignin binds to cellulase and inhibits enzymatic hydrolysis.Citation18) We hypothesized that the lignin structure of the solid fraction affects enzymatic hydrolysis efficiency. Solid fractions from the rice, wheat, and sorghum varieties providing the highest and lowest glucose yields were analyzed by 2D-NMR to resolve the lignin components in detail.Citation13) The spectra related to lignin aromatic regions and methoxyl were lower in bmr-6 than in Icr-38 sorghum (Fig. and Supplementary Table 1). The spectra related to lignin aliphatic linkages were low in both bmr-6 and Icr-38 sorghum. This result is in line with the previous research showing that most of lignin linkages are cleaved by dilute acid pretreatment.Citation15) Our NMR data suggested that, whereas the lignin levels substantially differ, composition and structure of lignin remained in bmr-6 and Icr-38 solid fractions were overall similar. Thus, the different enzymatic hydrolysis efficiencies observed for the sorghum solid fraction could be attributed mainly to the differences in their residual lignin levels. Low lignin content in bmr-6 solid fraction was reasonable as previous study reported that non-pretreated sorghum mutant bmr-6 also contained lower level of lignin than wild type sorghum.Citation19) In contrast, no clear difference was observed in the 2D NMR results of solid fractions from different rice and wheat varieties (Supplementary Fig. 1), suggesting that another factor, such as the crystallinity of the cellulose, is affecting enzymatic hydrolysis.
Fig. 4. 2D-NMR analysis of the solid fractions obtained after pretreatment of two sorghum varieties, bmr-6 (blue) and Icr-38 (green). The peak intensities of the lignin components are shown. Chemical shifts and references for the assignments are shown in Supplementary Table 1.
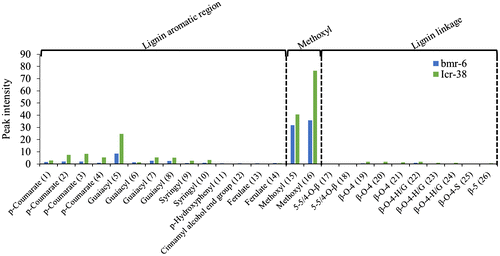
It is important to select a lignocellulose biomass variety that produces a high amount of glucose from the solid fraction after dilute acid pretreatment and enzymatic hydrolysis. A pretreatment temperature of 180 °C and an enzymatic load of 6.6 FPU were used to investigate the glucose yield from the solid fractions of 14 rice, 8 wheat, and 16 sorghum varieties. The solid fractions of Ruriaoba, Mironovskaya 808, and bmr-6 produced the highest levels of glucose of the rice, wheat, and sorghum varieties investigated, respectively. The cellulose content of the solid fraction did not affect glucose yield. The residual level of the lignin aromatic regions in sorghum affects enzymatic hydrolysis efficiency.
Author contribution
HT, KS, and AK designed this study and drafted the manuscript. HT and HK performed the biomass pretreatment and compositional analysis. TS-h, FM, and JK carried out the NMR analysis. HK and CO analyzed sugars and byproducts. MY, ST, and TS-a cultivated the rice, wheat, and sorghum, respectively. HT performed enzymatic saccharification. All authors read and approved the final manuscript.
Disclosure statement
No potential conflict of interest was reported by the authors.
Funding
This work was supported in part by Grants-in-Aid from the NC-CARP project, the Ministry of Education, Culture, Sports, Science and Technology, Japan; by the Special Coordination Fund for the Promotion of Science and Technology, Creation of Innovation Centers for Advanced Interdisciplinary Research Areas (Innovative Bioproduction Kobe), MEXT, Japan; and by a KAKENHI Grant-in-Aid for Scientific Research (C) to HK [grant number JP16K07665] from JSPS, Japan.
Supplemental materials
The supplemental material for this paper is available at https://doi.org/10.1080/09168451.2017.1336922.
TBBB_1336922_Supplemental_Material.zip
Download Zip (101.1 KB)Notes
Abbreviations: 2D 1H-13C HSQC NMR, two-dimensional 1H-13C heteronuclear single quantum coherence nuclear magnetic resonance; ROI, region of interest; GC-MS, gas chromatography-mass spectrometry; FPU, filter paper units.
References
- Hou XD, Smith TJ, Li N, et al. Novel renewable ionic liquids as highly effective solvents for pretreatment of rice straw biomass by selective removal of lignin. Biotechnol Bioeng. 2012;109:2484–2493.10.1002/bit.v109.10
- Binod P, Sindhu R, Singhania RR, et al. Bioethanol production from rice straw: An overview. Bioresour Technol. 2010;101:4767–4774.10.1016/j.biortech.2009.10.079
- Mosier N, Wyman C, Dale B, et al. Features of promising technologies for pretreatment of lignocellulosic biomass. Bioresour Technol. 2005;96:673–686.10.1016/j.biortech.2004.06.025
- Wei W, Wu S, Liu L. Enzymatic saccharification of dilute acid pretreated eucalyptus chips for fermentable sugar production. Bioresour Technol. 2012;110:302–307.10.1016/j.biortech.2012.01.003
- Guragain YN, De Coninck J, Husson F, et al. Comparison of some new pretreatment methods for second generation bioethanol production from wheat straw and water hyacinth. Bioresour Technology. 2011;102:4416–4424.10.1016/j.biortech.2010.11.125
- Jia L, Sun Z, Ge X, et al. Comparison of the delignifiability and hydrolysability of wheat straw and corn stover in aqueous ammonia pretreatment. Bioresources. 2013;8:4505–4517.
- Chen F, Dixon RA. Lignin modification improves fermentable sugar yields for biofuel production. Nat Biotechnol. 2007;25:759–761.10.1038/nbt1316
- Arai-Sanoh Y, Ida M, Zhao R, et al. Genotypic variations in non-structural carbohydrate and cell-wall components of the stem in rice, sorghum, and sugar cane. Biosci Biotechnol Biochem. 2011;75:1104–1112.10.1271/bbb.110009
- Lynd LR, Wyman CE, Gerngross TU. Biocommodity engineering. Biotechnol Prog. 1999;15:777–793.10.1021/bp990109e
- Zheng Y, Pan Z, Zhang R. Overview of biomass pretreatment for cellulosic ethanol production. Int J Agric Biol Eng. 2009;2:51–68.
- Redding AP, Wang Z, Keshwani DR, et al. High temperature dilute acid pretreatment of coastal Bermuda grass for enzymatic hydrolysis. Bioresour Technol. 2011;102:1415–1424.10.1016/j.biortech.2010.09.053
- Matsuda F, Yamasaki M, Hasunuma T, et al. Variation in biomass properties among rice diverse cultivars. Biosci Biotechnol Biochem. 2011;75:1603–1605.10.1271/bbb.110082
- Kim H, Ralph J. Solution-state 2D NMR of ball-milled plant cell wall gels in DMSO-d6/pyridine-d5. Org Biomol Chem. 2010;8:576–591.10.1039/B916070A
- Okushita K, Komatsu T, Chikayama E, et al. Statistical approach for solid-state NMR spectra of cellulose derived from a series of variable parameters. Polym J. 2012;44:895–900.10.1038/pj.2012.82
- Teramura H, Sasaki K, Oshima T, et al. Changes in lignin and polysaccharide components in 13 cultivars of rice straw following dilute acid pretreatment as studied by solution-state 2D 1H-13C NMR. PLOS One. 2015;10:e0128417.10.1371/journal.pone.0128417
- Matano Y, Hasunuma T, Kondo A. Display of cellulases on the cell surface of Saccharomyces cerevisiae for high yield ethanol production from high-solid lignocellulosic biomass. Bioresour Technol. 2012;108:128–133.10.1016/j.biortech.2011.12.144
- Teramura H, Sasaki K, Oshima T, et al. Organosolv pretreatment of sorghum bagasse using a low concentration of hydrophobic solvents such as 1-butanol or 1-pentanol. Biotechnol Biofuels. 2016;9:1403.10.1186/s13068-016-0427-z
- Saini JK, Patel AK, Adsul M, et al. Cellulase adsorption on lignin: a roadblock for economic hydrolysis of biomass. Renewable Energy. 2016;98:29–42.10.1016/j.renene.2016.03.089
- Sattler SE, Saathoff AJ, Haas EJ, et al. A nonsense mutation in a cinnamyl alcohol dehydrogenase gene is responsible for the sorghum brown midrib6 Phenotype. Plant Physiol. 2009;150:584–595.10.1104/pp.109.136408