Abstract
Animals select appropriate diets to meet their nutritional requirements. Here, we demonstrate the availability for analysis of feeding preference using an orthopteran, the two-spotted cricket Gryllus bimaculatus. A time-course study of these insects, involving continuous recording and tracing behavior for 9 h, allowed us to monitor discrimination of diet that contained various nutrients.
The nutritional status of animal body alters according to various biological and physiological processes, including development and reproduction.Citation1–Citation3) It also changes the animal’s preference for nutrients to compensate for the lost and required energy. A number of studies have demonstrated that those instinctive nutritional selective behaviors have proven as “self-selection” in various species, including mammals and insects.Citation4–Citation6) Insects, in particular the fruit fly Drosophila melanogaster, have emerged as useful model animal. However, the fruit fly is too diminutive to investigate by observation and anatomical methodologies. On the other hand, it is difficult to investigate the nutrient-selective feeding behavior in other larger model insect species, such as the silkworm, Bombyx mori, and the migratory locust, Locusta migratoria because they are predominantly monophagous or phytophagous. Here, we demonstrate that a relatively large and omnivorous insect species, the two-spotted cricket Gryllus bimaculatus, is a useful model for exploring the underlying mechanisms between metabolism and selective feeding behavior.
The following are the experimental examples from our established methodology for investigating the dietary preference of crickets by monitoring their behavior. Continuous recording of cricket behavior allowed us to monitor and evaluate the feeding activities in detail. These include preference index (PI), calculated by counting the number of access to each diet per the number of total accesses to both diets during observation; locomotor activity, calculated using the velocity and distance walked; and time-course study in the transition of feeding preferences.
For the assays of artificial diets, we initially examined the relationship in dietary choice between calorie content and the amount of food intake by the crickets. The utilized artificial diets in this study was listed (Supplemental Table 1). Using four diets containing different calories (1, 2, 4, and 8 kJ/g) with the same nutritional proportion (33% casein, 33% dextrin, and 33% soybean oil), as previously described with minor modifications,Citation7) we measured the amount of food intake by crickets for 24 h. Casein, dextrin, and cellulose were purchased from CLEA Japan (Tokyo, Japan). Soybean oil was purchased from Wako Pure Chemical Industries (Osaka, Japan). Our results show that crickets preferred consuming 4 kJ/g diet more than the other diets (Fig. (A)), indicating that calorie content influences the amount of food intake and that there is an appropriate calorie for analysis of feeding behavior. Further, when the dietary calorie content was the same, the number of fecal pellets expelled by crickets that consumed 2 and 4 kJ/g diets were more strongly correlated with the food intake (R2 > 0.9, Fig. (B)). This indicates that the amount of food intake may easily be estimated by counting the number of fecal pellets expelled. From these observations, we decided to use the 4 kJ/g diet for the following experiments.
Fig. 1. Effect of dietary calorie content on the amount of food intake. (A) The amount of food intake was measured for one day (Mean ± SD, n = 7). Means with different letters indicate significant differences on Tukey’s post hoc test and (B) Relationship between fecal pellets and the amount of food intake by crickets. 1 kJ/g (open circles), 2 kJ/g (gray circles), 4 kJ/g (open squares), and 8 kJ/g diets (closed squares) were analyzed.
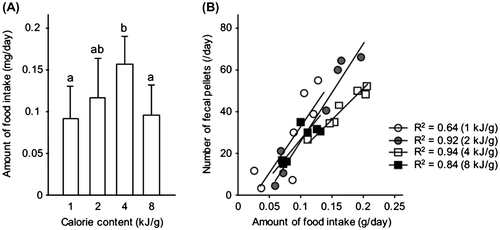
In a two-choice assay (Fig. (A)), crickets were continuously monitored in an arena (150ϕ × 20 mm), where two different diets were placed on both sides. The behavior of crickets was recorded using a video camera placed above the arena. The recorded behavior data were transferred as heat maps using a package called “kernel density estimation” in R programming language. In addition, feeding frequency from the movie data was analyzed with the “manual tracking” plug-in of Image J software and was estimated by counting the number of emergence on each diet block during the period of observation. PI was calculated as mentioned above; for two-choice assay between A and B diets as in the current study, PI was defined from the following formula; PI (%) = (the number of access to A diet) × 100/(the number of total accesses to both A and B diets). The access numbers were counted during observation (9 h). Further, video recording gave additional information about feeding behavior such as time-elapsed trend in feeding preference and locomotor activity (Fig. (A)). Heat maps gave another intuitive representation for individual feeding frequency with the data of trend in PI. Details on locomotor activity were also obtained from the velocity data. In this study, we performed the following three experiments of feeding preferences and used the analytical platforms described above for behavioral analyses.
Fig. 2. Two-choice assay using crickets. (A) Schematic diagram of the assay in this study, (B) Feeding preference in macronutrient. Feeding behavior on the diets including different nutrients (high-protein diet, high-carbohydrate, and high-lipid diet) was monitored for 6 or 9 h. Open, gray and dotted boxes represent the preference indexes (PIs) for high-protein diet, high-carbohydrate, and high-lipid diet, respectively. In the box plots, bars indicate minimum and maximum; box indicates the range of quartiles and median as a second quartile. PI was calculated by counting the ratio of feeding frequency for each nutrient-containing diet (**p < 0.01 and *p < 0.05 for one-sample Wilcoxon signed rank test, n = 7–8), (C) Feeding behavior on two different diets (high-lipid diet and low-lipid diet). Dotted and hatched boxes represent the PIs for data from crickets that consumed high-lipid diet and low-lipid diet, respectively (*p < 0.05 for one-sample Wilcoxon signed rank test, n = 5), and (D) Feeding behavior on two different diets (high-lipid diet and low-lipid# diet; identical nutrient contents except for lipid ratio in these diets). Dotted and black boxes represent the PIs for data from crickets that consumed high-lipid diet and low-lipid# diet, respectively (*p < 0.05 for one-sample Wilcoxon signed rank test, n = 7).
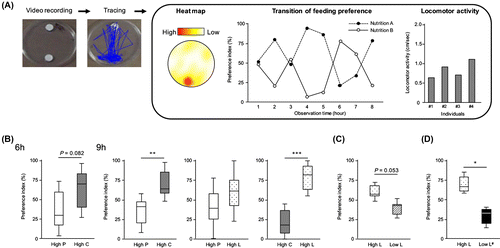
In the first experiment, we examined whether crickets can recognize different levels of macronutrients, such as protein, lipids, and carbohydrates, in artificial diets. Using high-protein diet (80% casein, 10% dextrin, and 10% soybean oil of total calorie content), high-carbohydrate diet (10% casein, 80% dextrin, and 10% soybean oil), and high-lipid diet (10% casein, 10% dextrin, and 80% soybean oil; Supplemental Table 1), we performed two-choice assays. To determine the adequate recording time for evaluating the preference, we analyzed the preference between high-protein diet and high-carbohydrate diet for 3, 6, 9, and 12 h. We could not obtain adequate preference data because of less frequencies of access to the diet from the 3 h observation (data not shown). During the 6 and 9 h observations, we found that crickets exhibit remarkable preference toward high-carbohydrate diet compared to high-protein diet (Fig. (B)). As data from the 12 h observation were similar to those from the 9 h observation; 9 h observation: p = 0.0173; 12 h observation: p = 0.0166 (data not shown), we performed the 9 h observation for further analyses of feeding preference. Among the three different diets, high-lipid diet was more preferred compared to high-carbohydrate diet, while high-protein diet and high-lipid diet were equally selected (Fig. (B)). The resulting data were somehow different from the predicted preference of high-lipid diet to high-protein diet. To resolve the conflict, we have to deal with many factors such as composition and components in the further study. These data concluded that the crickets perceived the nutrient content in the diet block and indicate their nutrient preference.
In the second experiment, we assayed the preference in the different lipid content using high-lipid diet (10% casein, 10% dextrin, and 80% soybean oil) and low-lipid diet (45% casein, 45% dextrin, and 10% soybean oil; Supplemental Table 1). The observation for 9 h revealed that crickets preferred high-lipid diet (Fig. (C)). To confirm whether this preference was because of the lipid content in the diet, we performed a two-choice assay using the high-lipid diet and the low-lipid# diet (Low L# in Fig. (D)) that contained one-eighth of the soybean oil but otherwise identical with respect to other nutrients as listed in Supplemental Table 1. We found that crickets preferred the high-lipid diet (Fig. (D)). These data indicate that crickets can discriminate the lipid content of the diet.
In our recent study, we found that palmitic acid (16:0), stearic acid (18:0), oleic acid (18:1), and linoleic acid (18:2) are the four predominant fatty acids present in the body of crickets, data not shown. Here, we studied the preference among the four fatty acids in the third experiment. Consequently, we found that crickets preferred the diet containing 18:0 over 16:0 (Fig. ). While 18:0 and 18:1 diets were equally selected, 18:2 diet seemed to be selectively avoided by crickets (Fig. ). These results indicate that crickets discriminate the chemical nature of fatty acids, as observed in D. melanogaster,Citation8) implying that crickets are good models for the analyses of nutrient-selective feeding behavior.
Fig. 3. Feeding preference on fatty acids. Feeding behavior on diets containing one of the four fatty acids (i.e. 16:0, 18:0, 18:1, and 18:2) was assayed for 9 h. Open, gray, dotted, and hatched boxes represent the PIs for 16:0, 18:0, 18:1, and 18:2, respectively. In the box plots, bars indicate minimum and maximum; box indicates the range of quartiles and median as a second quartile. Circles represent outliers. PI was calculated by percentiles of access frequency to two different diets (*p < 0.05 for one-sample Wilcoxon signed rank test, n = 5–10).
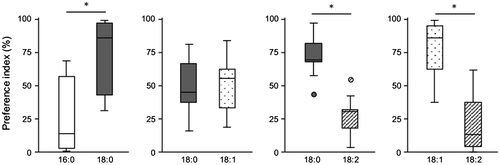
In summary, we demonstrated that crickets (Fig. ) can be used as models for the evaluation of nutritional selection or feeding preference. We also showed the preference of fatty acid by crickets, which may lead to understanding the relationship between nutritional requirement and feeding preference. It is known that dietary lipid is detected by the gustatory system in various species, including insects,Citation9,10) and nutrient sensing is influenced by metabolic state and is perceived by sensors including the antennae. However, studies on feeding preference of fatty acids is lacking except for a few behavioral studies.Citation8) It is noteworthy that the preference assay in crickets, described in this study, required a shorter period for gathering data on selective feeding (i.e. within half a day), while experiments using mice require approximately three days for the same.Citation11) In addition, experiments using crickets are less expensive than those using other animals. Hence, we propose that crickets could be the first choice of a model for studies on nutritional selection.
Supplemental data
The supplementary material for this article is available online at https://doi.org/10.1080/09168451.2017.1343119
Author contribution
KF performed the experiments. KF and SN analyzed the data. KF, SN designed this project. KF and SN wrote the paper.
Disclosure statement
No potential conflict of interest was reported by the authors.
Funding
This work was partially supported by Grants-in-Aid for Scientific Research-Japan Society for the Promotion of Science (JSPS)-KAKENHI [grant number 15H04609] and [grant number 16K15066].
Supplemental_Table.jpg
Download JPEG Image (84.7 KB)References
- Lorenz MW, Anand AN. Changes in the biochemical composition of fat body stores during adult development of female crickets, Gryllus bimaculatus. Arch Insect Biochem Physiol. 2004;56:110–119.10.1002/(ISSN)1520-6327
- Lin X, Yao Y, Wang B, et al. Ecological trade-offs between migration and reproduction are mediated by the nutrition-sensitive insulin-signaling pathway. Int J Biol Sci. 2016;12:607–616.
- Smykal V, Raikhel AS. Nutritional control of insect reproduction. Curr Opin Insect Sci. 2015;11:31–38.
- Richter CP, Holt LE Jr., Barelare B Jr., et al. Nutritional requirements for normal growth and reproduction in rats studied by the self-selection method. Am J Physiol. 1938;122:734–744.
- Friedman S, Waldbauer GP, Eertmoed JE, et al. Blood trehalose levels have a role in the control of dietary self-selection by Heliothis zea larvae. J Insect Physiol. 1991;37:919–928.
- Simpson SJ, Abisgold JD. Compensation by locusts for changes in dietary nutrients: behavioural mechanisms. Physiol Entomol. 1985;10:443–452.
- Tsukamoto Y, Kataoka H, Nagasawa H, et al. Mating changes the female dietary preference in the two-spotted cricket Gryllus bimaculatus. Front Physiol. 2014;5:95.
- Fougeron AS, Farine JP, Flaven-Pouchon J, et al. Fatty-acid preference changes during development in Drosophila melanogaster. PLoS ONE. 2011;6:e26899.
- Gaillard D, Laugerette F, Darcel N, et al. The gustatory pathway is involved in CD36-mediated orosensory perception of long-chain fatty acids in the mouse. FASEB J. 2008;22:1458–1468.
- Masek P, Keene AC. Drosophila fatty acid taste signals through the PLC pathway in sugar-sensing neurons. PLoS Genet. 2013;9:e1003710.
- von Holstein-Rathlou S, BonDurant LD, Peltekian L, et al. FGF21 mediates endocrine control of simple sugar intake and sweet taste preference by the liver. Cell Metab. 2016;23:335–343.