Abstract
Most Brassicaceae vegetables are ideal dietary sources of antioxidants beneficial for human health. Cardamine fauriei (Ezo-wasabi in Japanese) is a wild, edible Brassicaceae herb native to Hokkaido, Japan. To clarify the main antioxidative phytochemical, an 80% methanol extraction from the leaves was fractionated with Diaion® HP-20, Sephadex® LH-20, and Sep-Pak® C18 cartridges, and the fraction with strong antioxidant activity depending on DPPH method was purified by HPLC. Based on the analyses using HRESIMS and MS/MS, the compound might be N1, N14-diferuloylspermine. This rare phenol compound was chemically synthesized, whose data on HPLC, MS and 1H NMR were compared with those of naturally derived compound from C. fauriei. All results indicated they were the same compound. The radical-scavenging properties of diferuloylspermine were evaluated by ORAC and ESR spin trapping methods, with the diferuloylspermine showing high scavenging activities of the ROO·, O2·−, and HO· radicals as was those of conventional antioxidants.
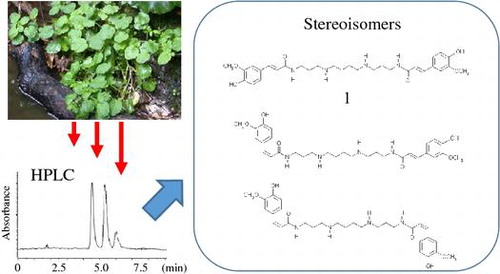
The increase of oxidative stress in an organism can be the cause of several diseases. Oxidative stress is caused by Reactive Oxygen Species (ROS); including the ROO·, O2·− and HO· radicals.Citation1) In general, ROS are produced by respiration, photosynthesis, and some cell-mediated immune functions.Citation2,3) ROS induced oxidative damage to biomolecules such as lipids, nucleic acids, proteins, and carbohydrates can result in aging, cancer, and many other diseases. The HO· radical is very reactive among ROS, and leads to damage in cellular components because it can rapidly attack several molecules.Citation4) Furthermore, the HO· radical leads to lipid peroxidation because it is capable of starting oxidation of polyunsaturated fatty acids. Antioxidants, such as those rich varieties found in fruits and vegetables, edible horticultural products, can protect from ROS.Citation5) The consumption of vegetables and fruits can decrease the risk of heart disease and many types of cancer.Citation6,7) Typical dietary antioxidants include ascorbic acid, tocopherols, carotenoids, and flavonoids.Citation2) Phenolic compounds, ubiquitous in plants are an essential part of the human diet, and are of considerable interest due to their antioxidant properties.Citation8) The Brassicaseae plant is a rich source of these antioxidants.Citation9)
Cardamine fauriei Maxim., also known in Japan as Ezo-wasabi, is a plant native to Hokkaido, Japan, and is a perennial Brassicaceae plant. The plant has a unique wasabi-like flavor caused by three glucosinolates,Citation10) making it a popular edible wild herb in Hokkaido.Citation11) Micropropagation technique and hydroponic culture system for the cultivation of the herb had been established.Citation12) However, C. fauriei is not a commercial vegetable yet. To stimulate interest in cultivating C. fauriei as a novel vegetable and as an ingredient of functional foods, it is important to clarify the beneficial antioxidant component contained in the plant. In this study, we examined the C. fauriei plant for any antioxidative phytochemicals.
Materials and methods
Reagents
For the fractionation process: Diaion® HP-20 was purchased from Mitsubishi Chemical (Tokyo, Japan), Sephadex® LH-20 from Sigma Aldrich Japan (Tokyo, Japan) and the Sep-Pak® C18 cartridges from Waters (Tokyo, Japan). For the chemical synthesis: 4-hydroxy-3-methoxycinnamic acid ethyl ester (ethyl ferulate), pyridinium p-toluenesulfonate (PPTS), 3,4-dihydro-2H-pyran (DHP), N,N′-dicyclohexylcarbodiimide (DCC), and N-hydroxysuccinimide (NHS) were purchased from Tokyo Chemical Industry (Tokyo, Japan) and spermine from Nacalai Tesque (Kyoto, Japan). For the antioxidant activity analysis: 2,2-diphenyl-1-picrylhydrazyl (DPPH) and fluorescein sodium salt were purchased from Sigma Aldrich Japan (Tokyo, Japan), 2,2′-azobis(2-amidinopropane)dihydrochloride (AAPH), H2O2, diethylenetriaminepentaacetic acid (DTPA), glycine and riboflavin from Wako Pure Chemical (Tokyo, Japan), 2- (5,5-dimethyl-2-oxo-2λ5-[1,3,2] dioxaphosphinan-2-yl) -2-methyl-3,4-dihydro-2H-pyrrole 1-oxide (CYPMPO) from Radical Research (Hino, Japan). For the standards: 6-hydroxy-2,5,7,8-tetramethylchroman-2-carboxylic acid (Trolox) was purchased from Sigma Aldrich Japan (Tokyo, Japan), ferulic acid, L-ascorbic acid and α-lipoic acid from Wako Pure Chemical (Tokyo, Japan), and quercetin from Kanto Chemical (Tokyo, Japan).
Plant material
The C. fauriei plants utilized for this study were hydroponically cultured as described previously.Citation12) Mature leaves were frozen with liquid nitrogen and lyophilized. These freeze-dried samples were then powdered.
Extraction, fractionation, and purification of antioxidative phytochemical
All fractions were analyzed by DPPH method for screening antioxidative phytochemical, and the highest active fraction was separated by subsequent chromatography. Analysis using this artificial radical was carried out as described previously.Citation13) The fractions evaporated and dissolved in 80% EtOH were used as samples for DPPH assay. The 50 μL of the samples or the standards were added to a 150 μL solution of DPPH (400 μM in EtOH): morpholinoethanesulfonic acid (MES) buffer (pH 6.0, 200 mM): 20% EtOH = 1:1:1 (v/v/v) into 96-well plate. The mixture was left to stand at room temperature for 20 min; then the absorbance was read at 520 nm in a microplate reader (Powerscan HT; DS Pharma Biomedicals, Osaka, Japan). DPPH radical scavenging activity was estimated as the μmol Trolox equivalent of a sample using the standard curve of Trolox.
The lyophilized leaves (14 g) of C. fauriei were extracted with 1.0 L of 80% (v/v) MeOH for 24 h. The extract was filtrated and evaporated to give crude material, which was subjected to column chromatography using a glass column (500 mm × 20 mm) packed with Diaion® HP-20 (50 g) and eluted by a stepwise gradient of water and MeOH. The 80% MeOH fraction was chromatographed using a glass column (500 mm × 20 mm) packed with Sephadex® LH-20 (30 g) and eluted with MeOH. The mixed fraction having strong antioxidant activity was further purified by Sep-Pak® C18 cartridges and eluted with a stepwise gradient of water and MeOH. The 50% MeOH fraction was finally purified by HPLC to afford natural compound 1 (0.5 mg); 1H NMR (MeOH-d4, 500 MHz) and 13C-NMR (MeOH-d4, 125 MHz): see Tables and ; HRESIMS: m/z 555.3177 [M + H]+ (calcd for C30H43O6N4, 555.3183).
Table 1. 1H-and 13C-NMR dataTable Footnotea of compound 1 (E form) in MeOH-d4.
Table 2. 1H-and 13C-NMR dataTable Footnotea of compound 1 (Z form) in MeOH-d4.
Synthesis of N1,N14-diferuloylspermine
The synthesis of N1, N14-diferuloylspermine was carried out with some modifications, as described previously.Citation14)
Synthesis of compound 4
To a stirred solution of compound 2 (5 g, 22.5 mmol) in CH2Cl2 (70 mL) was added PPTS (630 mg, 2.51 mmol) and DHP (4.4 g, 51.8 mmol), and the reaction mixture was further stirred for 12 h. The usual workup was employed and the resulting material was subjected to silica gel column chromatography (Si 150 g, EtOAc: n-hexane = 2:8) to afford compound 3. To a stirred mixture of compound 3 in EtOH (40 mL) was added KOH (2.2 g, 39.2 mmol), and the reaction mixture was further stirred for 12 h. The usual workup was employed, and the resulting material was subjected to silica gel column chromatography (Si 140 g, EtOAc: n-hexane = 2:8) to compound 4 (2.52 g, 9 mmol, 40% from compound 2); 1H NMR (CDCl3, 270 MHz) δ 7.70 (1H, d, J = 16.0 Hz, H-7), 7.11–7.06 (3H, m, H-2, H-5 and H-6), 6.29 (1H, d, J = 16.0 Hz, H-8), 5.46 (1H, m, THP) 3.87 (4H, complex, OCH3 and THP), 3.61 (1H, m, THP), 1.63–2.09 (6H, m, THP); EIMS m/z 278 [M]+ (3) 194 (100), 85 (37), 41 (21).
Synthesis of compound 5
To a stirred mixture of compound 4 (2.52 g, 9 mmol) in DMF (20 mL) and THF (60 mL) was added NHS (4.1 g, 36 mmol) and DCC (3.6 g, 18 mmol), and the reaction mixture was further stirred for 12 h. The usual workup was employed, and the resulting material was subjected to silica gel column chromatography (Si 150 g, EtOAc: n-hexane = 4:6) to compound 5 (200 mg, 0.5 mmol, 6%); 1H NMR (CDCl3, 270 MHz) δ 7.84 (1H, d, J = 16.0 Hz, H-7), 7.16–7.07 (3H, m, H-2, H-5 and H-6), 6.43 (1H, d, J = 16.0 Hz, H-8), 5.47 (1H, m, THP), 3.88 (4H, complex, OCH3 and THP), 3.60 (1H, m, THP), 2.85 (4H, s, OSu), 1.54–2.02 (6H, m, THP); FDMS m/z 377 (3) [M]+, 85 (100), 290 (94), 375 (44).
Synthesis of compound 1
To a stirred mixture of compound 5 (200 mg, 0.5 mmol) in CH2Cl2 (40 mL) cooled with ice was added compound 6 (54.46 mg, 0.27 mmol), and the reaction mixture was further stirred for 12 h. The resultant mixture was roughly purified to give compound 7. The protective group of compound 7 was removed using PPTS (100 mg, 0.4 mmol) in MeOH according to the usual manner. The resultant crude mixture was purified by HPLC, whose condition was mentioned above, to afford compound 1 (2 mg, 14 μmol, 3%).
HPLC
The fraction obtained from the Sep-Pak® C18 cartridges was separated and purified by HPLC under the following conditions: intelligent pump, L-2160 (Hitachi, Tokyo, Japan); column, Inert Sustain C18 (3 × 150 mm, GL Sciences, Tokyo, Japan); column temperature, 40 °C (Model CO631A, GL Sciences, Tokyo, Japan); PDA detector, 280 nm (Model L-2455U, Hitachi, Tokyo, Japan); Auto sampler, L-2200U (Hitachi), flow rate, 0.5 mL min−1; mobile phase, gradient analysis of aq.1.5% formic acid (v/v) and MeOH.
HRESIMS, MS/MS, EIMS and FDMS
The purified fraction from HPLC was analyzed in positive ion mode using a LTQ-Orbitrap XL (ThermoScientific, Waltham, USA) under the following conditions: ionization, electro-spray ionization; m/z, 150–2000; spraying voltage, 2.1 kV; capillary temp., 200 °C; capillary voltage, 40 V; tube lens voltage, 180 V; activation type, collisionally induced dissociation (CID); normalized collision energy, 35%; isolation width, 1.0; activation time, 30 ms. The synthesized compound was analyzed by EIMS and FDMS spectra, and these analyses were recorded with JMS-SX102A (JEOL Tokyo, Japan) and JMS-T 100GCV (JEOL, Tokyo, Japan) spectrometers.
NMR
1H NMR, 13C NMR, 1H-1H COSY, HMBC and HSQC were recorded on a Bruker AMX-500 or a JEOL JNM EX-270. A sample of approximately 4 mg was dissolved in MeOH-d4 or CDCl3 and used for recording the spectra. Chemical shift values were expressed in ppm relative to the internal standard, tetramethylsilane.
Antioxidant activity for natural ROS
The synthesized N1, N14-diferuloylspermine (diferuloylspermine) and conventional antioxidants (ferulic acid, quercetin, ascorbic acid and Trolox) were dissolved in MWA (methanol: water: acetic acid = 90:9.5:0.5 (v/v/v)). The activity of scavenging natural ROS (ROO·, O2·− and HO· radical, respectively) was estimated utilizing the following methods.
ORAC method
Analysis was carried out according to the previous method.Citation15) Thirty-five microliters of the synthesized diferuloylspermine, conventional antioxidants, Trolox standards or a blank were added to a 115 μL solution of fluorescein (110.7 mmol/L) and a 50 μL solution of AAPH (31.7 mmol/L) into a 96-well plate. After covering the plate with a film (NJ-500; Takara Bio, Otsu, Japan), the fluorescence intensity (excitation at 485 nm, emission at 530 nm) was monitored at 37 °C every 2 min for a total of 90 min using a microplate reader. The net area under the curve (AUC) was calculated by subtracting the AUC for the blank from the reagents or standards. The ORAC value was estimated as the μmol Trolox equivalent of a sample using the standard curve of Trolox.
ESR spin trapping method
Analysis was carried out according to the method as described previously.Citation16–18) The 50 μL of the synthesized diferuloylspermine, conventional antioxidants, the standards or a blank were added to a 50 μL solution of precursor/sensitizer, a 20 μL solution of CYPMPO (10 mmol/L) and an 80 μL solution of sodium phosphate buffer into an ESR flat cell. In these cases, the precursor/sensitizer reagents utilized for superoxide and hydroxyl radicals were riboflavin and hydrogen peroxide, respectively. α-lipoic acid and ascorbic acid were used as the standard scavengers for superoxide and hydroxyl radicals, respectively. The ESR flat cell was set in an ESR cavity, and was then irradiated 5 s with ultraviolet ray for producing radicals. At this time, the ESR spectrum was immediately measured using an X-band ESR spectrometer (JES-RE1X, JEOL, Tokyo, Japan) with a 100 kHz field modulation. The spectrometer conditions were as follows: resonance field, 3521 G; field modulation width, 1.0 G; microwave power, 6 mW; light source, 200 W medium pressure mercury/xenon arc lamp (LC-8, Hamamatsu Photonics K.K., Hamamatsu, Japan); UV irradiation intensity for photolysis, 2.78 mW/cm2 (LC-8, Hamamatsu Photonics K.K., Hamamatsu, Japan) measured by a UV intensity meter (Cole-Parmer International, Illinois, USA); the band-pass filter, G-533 (HOYA, Tokyo, Japan). The analysis of adducted signal was carried out as described by Kameya et al.Citation17). The scavenging activities were estimated as the μmol standard equivalent of a sample using the standard curve.
Statistical analyses
Analysis of the antioxidant activity of the synthesized compound and each standard was performed three times independently. Results are shown as an average ± SE (n = 3). Data were analyzed statistically using analysis of variance (ANOVA) and Fisher’s F-test followed by Tukey’s Multiple Range Test.
Results and discussion
Elucidation of the antioxidative phytochemical in C. fauriei
The DPPH method has been used popularly for screening antioxidant potential of both individual phenolics and biologically relevant samples like foods,Citation19) and for measuring radical scavenging capacity of pure compounds, food constituents, plant extracts, and the other samples such as synthesized compoundsCitation2). Therefore, we employed the DPPH method for screening antioxidative compounds. Using several purification steps (Fig. S1), a most active ingredient, compound 1 (0.5 mg, Fig. ), having antioxidant property was purified from lyophilized leaves (14 g) of C. fauriei. Although the compound 1 was once purified, re-chromatogram of compound 1 using HPLC gave three major peaks in HPLC feature (Fig. ).
The purified compound showed the accurate mass values at m/z 555.3177 and the molecular formula was estimated to be C30H43O6N4 [M + H] +. In the ESIMS/MS spectra, typical fragments were observed at m/z 177, 234, and 305, which are distinctive for diferuloylspermine residues.Citation20) Furthermore, the 1H NMR spectrum showed resonances of aromatic protons at 6.75–7.34 ppm, methyl proton at 3.87 ppm and methylene in spermine part at 1.73–3.41 ppm. Therefore, it was hypothesized that natural compound might be N1, N14-diferuloylsperimine. To confirm the hypothesis, the N1, N14-diferuloylspermine was chemically synthesized according to the reported method with some modifications (Fig. S2).Citation14) The 1H-NMR (Fig. (A)) and MS data of synthesized compound showed well coincidence with those of naturally obtained diferuloylspermine, compound 1. Therefore, the chemical structure of the isolated natural compound 1 was determined to be N1, N14-diferuloylsperimine.
Fig. 3. 1H NMR analysis of naturally obtained and synthesized compound 1.
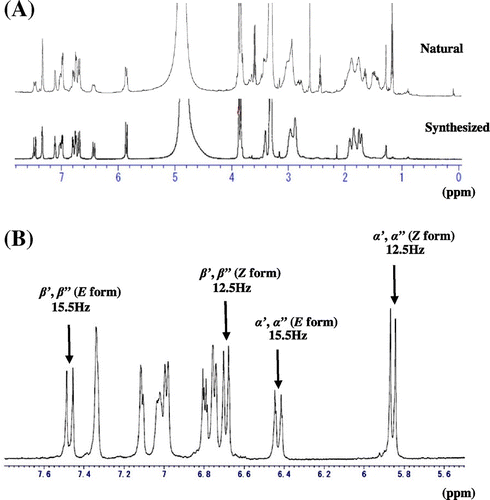
It has been generally accepted that (E) geometry of olefin in α, β unsaturated carbonyl moiety should be more stable than (Z) one, although these are interchangeable, and we had guessed that the synthesized compound should have (E) geometry of olefin due to use of compound 2 as a starting material. However, the 1H NMR spectrum of synthesized compound 1 was complicated as same as that of naturally derived N1, N14- diferuloylspermine (1). Therefore, it was determined that some parts of (E) geometry of olefin were interconverted to (Z) configuration when the coupling reaction of 5 and 6 or leaving the protection group for phenolic hydroxyl moiety in 7 was performed. The cross peak in HMBC between δ 170.1 (–CONH–)/δ 6.43 (–CHβ = CHα–, J = 15.5 Hz, Fig. (B)) and 3.41 (H-2) were observed, which established the 13C and 1H assignments of (E) form part together with the information of HSQC which confirmed direct connectivity between H and C and 1H–1H COSY which indicated the connectivity of H-2/H-3/H-4 and H-6/H-7. Applying the same strategy of building up the connectivity using the information of cross peaks between δ 171.0 (–CONH–)/δ 5.85 (–CHβ = CHα–, J = 12.5 Hz, Fig. (B)) and 3.34 (H-2) in HMBC spectra together pursuing the cross peaks in HSQC and 1H–1H COSY spectra, the 13C and 1H assignments of (Z) form moiety were determined. Since we could not find the cross peak between δ 2.97 (H-4 and 6 of E form) and 46.6 (C-4 and 6 of Z form) nor δ 2.88 (H-4 and 6 of Z form) and 46.4 (C-4 and 6 of E form), we established above total assignments. It might be the possibility that the assignments of H-6 and C-6 were δ 2.97 and 46.4 in (Z) form and δ 2.88 and 46.6 in (E) geometry. This was the reason why we put the interchangeable possibility in Tables and . We could not determine for abundance ratio of the (E, E), (E, Z), and (Z, Z) stereoisomers of the synthesized and naturally obtained compound 1 at room temperature. But, we reached conclusion that each (E, E), (E, Z), and (Z, Z) stereoisomer existed because re-chromatogram of purified naturally obtained and synthesized compound 1 gave three peaks around 5 min having the accurate mass values at m/z 555.3177 in HRESIMS analysis (Fig. ). Therefore, data of Tables should be considered to be resulted from mixture of (E, E), (E, Z), (Z, Z) forms (Figs. S3 and S4). To clarify the abundance ratio of the isomers, it should be necessary to analyze prior to change into the stereoisomers such as using HPLC connecting with NMR. Finally, the total assignments of 1H and 13C NMR of N1, N14-diferuloylsperimine (1) for (E) and (Z) forms were firstly given in this paper (Tables and ).
Above-mentioned confirmation, (E) and (Z) mixture, was agreed with the previous reports,Citation21–23) in which they reported that the complexity of the NMR spectrum of hydroxycinnamic acid spermidines was attributed to the mixture of E–Z configurational isomers. Furthermore, it was also found that the isomers (E or Z form) of spermidine conjugate changed dramatically and rapidly upon exposure to sunlight and irradiation by UV.Citation22,23) Based on our synthetic experiment, it was firstly reconfirmed that diferuloylspermine can be easily converted into (E, E), (E, Z), and (Z, Z) stereoisomers.
Antioxidant activity of diferuloylspermine
Although diferuloylspermine was found to be an antioxidative phytochemical for DPPH· artificial radical in this study, it was not clear if this compound could have scavenging activity for naturally occurred ROS. So, the antioxidant activity of diferuloylspermine was evaluated by the ORAC (ROO· radical) and ESR spin trapping (O2·− and HO· radicals) methods, then compared with those of conventional antioxidants: quercetin, ferulic acid, ascorbic acid, and Trolox (Fig. ). In this case, the content of DFSM (1.01 μmol g DW−1) in C. fauriei leaves was greater than that of quercetin(0.12 μmol g DW−1), a major antioxidant in Brassica vegetables.Citation24) With the ORAC (ROO· radical) and ESR spin trapping (O2·− radical) assay, the radical scavenging activities of diferuloylspermine were the same or larger than those of conventional antioxidants except for quercetin with the highest values. These results supported the previous fact that quercetin showed higher scavenging activities than Trolox for both ROO· and O2·− radical.Citation5) In addition, with the ESR spin trapping (HO· radical) assay, diferuloylspermine showed the highest activity among antioxidants examined. It has been considered that ferulic acid and ascorbic acid are powerful antioxidants.Citation2,25,26) Furthermore, flavonoids including quercetin is recognized to be antioxidants, and they have high scavenging activity for HO· radical.Citation2,27) Since diferuloylspermine showed the same scavenging activities for ROO· and O2·− radicals as ferulic acid and ascorbic acid, and the highest scavenging activity for HO· radical, it might be that diferuloylspermine has a good potential of natural antioxidant correspond to conventional antioxidants. It was reported that N1, N5, N10-Tris (4-hydroxycinnamoyl) spermidine showed scavenging activity for DPPH radical, whereas analog hydroxyl groups which were methylated exhibited a very weak activity.Citation28) This report also indicated that the scavenging activity was caused by presence of the phenolic OH groups of hydroxycinnamoyl moieties. Furthermore, spermine was reported to exhibit HO· radical scavenging activity.Citation29) Therefore, it seemed that the high HO· radical scavenging activity of diferuloylspermine was due to OH groups of ferulic acid and spermine moiety.
Fig. 4. Antioxidant activity about synthesized diferuloylspermine and antioxidant standards.
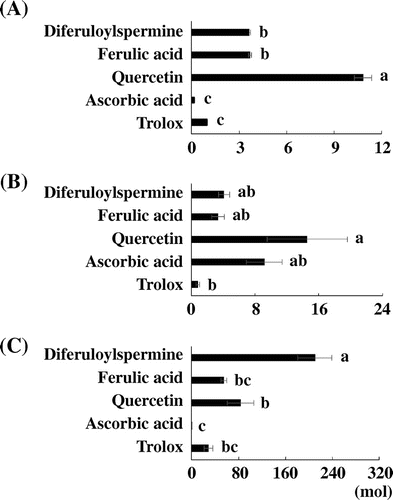
As physiological function of diferuloylspermine, N1, N14-bis (dihydrocaffeoyl) spermine, Kukoamine A, was isolated from the root bark of Lycium chinese as clinically effective hypotensive compound.Citation30) Furthermore, Kukoamine A and the analogs showed anti-trypanosomal activity due to inhibit of trypanothione reductase.Citation31) Since the structure of diferuloylspermine is analogs to Kukoamine A, this compound might also have those physiological functions. Previously, diferuloylspermine was found only in the reproductive organs of Ananas comosms, Gomphrena globose, and Zea mays.Citation32,33) This is the first report on diferuloylspermine isolated from Brassicaceae plants, especially not from reproductive organs but vegetative parts of the plant.
Author contributions
Study concept and design: Takashi Suzuki. Acquisition of data: Keima Abe, Hideyuki Matsuura, and Mitsuko Ukai. Analysis and interpretation of data: Keima Abe, Hideyuki Matsuura, Mitsuko Ukai, Hanako Shimura, Hiroyuki Koshino, and Takashi Suzuki. Drafting of the manuscript: Keima Abe, Hideyuki Matsuura, and Takashi Suzuki. All authors reviewed and approved the final manuscript.
Disclosure statement
No potential conflict of interests was reported by the authors.
Funding
This work was supported by Japan Society for the Promotion of Science [grants-in-Aid for Scientific Research (B) number 25292017].
Supplemental material
Supplemental material for this article can be accessed at https://doi.org/10.1080/09168451.2017.1356214.
Supplemental_Fig._S4.ppt
Download MS Power Point (166.5 KB)Supplemental_Fig._S3.ppt
Download MS Power Point (180.5 KB)Supplemental_Fig._S2.ppt
Download MS Power Point (109 KB)Supplemental_Fig._S1.ppt
Download MS Power Point (262.5 KB)Acknowledgments
We thank Dr. K. Sugiyama, Dr. D. Kami and Ms. N. Murata (National Agricultural Research Center for Hokkaido Region) for technical assistance on extraction and separation, Dr. J. Watanabe (National Food Research Institute, National Agriculture and Food Research Organization) on ORAC method, and Ms. S. Oka (Center for Instrumental Analysis, Hokkaido University) on mass spectrometry.
References
- Rucker RB. Reactive oxygen species: production, regulation, and essential functions. In: Zampelas A, Micha R, editors. Antioxidants in health and disease. New York (NY): CRC Press; 2015. p. 3–22.10.1201/b18539
- Gulcin I. Antioxidant activity of food constituents: an overview. Arch Toxicol. 2012;86:345–391.10.1007/s00204-011-0774-2
- Scandalios GJ. Oxygen stress and superoxide dismutases. Plant Physiol. 1993;101:7–12.10.1104/pp.101.1.7
- Diplock TA, Charleux LJ, Crozier-Willi G, et al. Functional food science and defense against reactive oxidative species. British J Nutr. 1998;80(Suppl. 1):S77–S112.10.1079/BJN19980106
- Tabart J, Kevers C, Pincemail, et al. Comparative antioxidant capacities of phenolic compounds measured by various tests. Food Chem. 2009;113:1226–1233.10.1016/j.foodchem.2008.08.013
- Mazza G. Diet and human health: functional foods to reduce disease risks. Acta Hortic. 2004;642:161–172.10.17660/ActaHortic.2004.642.18
- Weisburger HJ. Mechanisms of action of antioxidants as exemplified in vegetables, tomatoes and tea. Food Chem Toxicol. 1999;37:943–948.10.1016/S0278-6915(99)00086-1
- Balasundram N, Sundram K, Samman S. Phenolic compounds in plants and agri-industrial by-products: Antioxidant activity, occurrence, and potential uses. Food Chem. 2006;99:191–203.10.1016/j.foodchem.2005.07.042
- Bjorkman M, Klingen I, Birch NEA, et al. Phytochemicals of Brassicaceae in plant protection and human health—Influences of climate, environment and agronomic practice. Phytochemistry. 2011;72:538–556.10.1016/j.phytochem.2011.01.014
- Abe K, Kido S, Maeda T, et al. Glucosinolate profiles in Cardamine fauriei and effect of light quality on glucosinolate concentration. Sci Hortic. 2015;189:12–16.10.1016/j.scienta.2015.03.028
- Kami D, Kido S, Otokita K, et al. Cryopreservation of shoot apices of Cardamine yezoensis in vitro-cultures by vitrification method. Jpn Soc Cryobiol Cryotechnol. 2010;56:119–126 . (In Japanese with English abstract).
- Maeda T, Kami D, Kido S, et al. Development of asexual propagation system via in vitro culture in Cardamine yezoensis Maxim. and its application to hydroponic cultivation. J Jpn Soc Hort Sci. 2008;77:270–276.10.2503/jjshs1.77.270
- Suda I. Determination of DPPH radical scavenging activity by spectrophotometry. In: Shinohara K, Suzuki T, Kaminogawa S, editors. The methods of food functions analysis. Tokyo: Korin; 2000. p. 218–220 . (in Japanese).
- Garnelis T, Athanassopoulos MC, Papaioannou D, et al. Very short and efficient syntheses of the spermine alkaloid kukoamine A and analogs using isolable succinimidyl cinnamates. Chem Lett. 2005;34:264–265.10.1246/cl.2005.264
- Watanabe J, Oki T, Takebayashi J, et al. Method validation by interlaboratory studies of improved hydrophilic oxygen radical absorbance capacity methods for the determination of antioxidant capacities of antioxidant solutions and food extracts. Anal Sci. 2012;28:159–165.10.2116/analsci.28.159
- Kameya H, Ukai M. Hydroxyl radical scavenging ability of instant coffee evaluated by ESR spin trapping. J Cookery Sci Jpn. 2012;45:33–36 . (In Japanese with English abstract).
- Kameya H, Watanabe J, Takano-Ishikawa Y, et al. Comparison of scavenging capacities of vegetables by ORAC and EPR. Food Chem. 2014;145:866–873.10.1016/j.foodchem.2013.09.015
- Ukai M, Kawamura S, Kishida K, et al. Superoxide radical scavenging ability of green tea measured by the ESR spin trapping method. J Cookery Sci Jpn. 2013;46:45–49 . (In Japanese with English abstract).
- Roginsky V, Lissi AE. Review of methods to determine chain-breaking antioxidant activity in food. Food Chem. 2005;92:235–254.10.1016/j.foodchem.2004.08.004
- Zamble A, Hennebelle T, Sahpaz S, et al. Two new quinoline and tris (4-hydroxycinnamoyl) spermine derivatives from microdesmis keayana roots. Chem Pharm Bull. 2007;55:643–645.10.1248/cpb.55.643
- Bokern M, Witte L, Wray V, et al. Trisubstituted hydroxycinnamic acid spermidines from Quercus dentata pollen. Phytochemistry. 1995;39:1371–1375.10.1016/0031-9422(95)00151-V
- Werner C, Hu W, Lorenzi-Riatsch A, et al. Di-coumaroylspermidine and tri-coumaroylspermidines in anthers of different species of the genus Aphelandra. Phytochemistry. 1995;40:461–465.10.1016/0031-9422(95)00288-I
- Sobolev SV, Sy AA, Gloer BJ. Spermidine and flavonoid conjugates from peanut (Arachis hypogaea) flowers. J Agric Food Chem. 2008;56:2960–2969.10.1021/jf703652a
- Cartea ME, Francisco M, Soengas P, Velasco P. Phenolic compounds in Brassica vegetables. Molecules. 2011;16:251–280.
- Bendich A, Machlin JL, Scandurra O. The antioxidant role of vitamin C. Free Radic Biol Med. 1986;2:419–444.10.1016/S8755-9668(86)80021-7
- Srinivasan M, Sudheer RA, Menon PV. Ferulic acid: therapeutic potential through its antioxidant property. J Clin Biochem Nutr. 2007;40:92–100.10.3164/jcbn.40.92
- Husain RS, Cillard J, Cillard P. Hydroxyl radical scavenging activity of flavonoids. Phytochemistry. 1987;26:2489–2491.10.1016/S0031-9422(00)83860-1
- Zamble A, Sahpaz S, Hennebelle T, et al. N1, N5, N10-Tris (4-hydroxycinnamoyl) spermidines from Microdesmis keayana roots. Chem Biodiversity. 2006;3:982–989.10.1002/(ISSN)1612-1880
- Ha CH, Sirisoma SN, Kuppusamy P, et al. The natural polyamine spermine functions directly as a free radical scavenger. PNAS. 1998;95:11140–11145.10.1073/pnas.95.19.11140
- Funayama S, Yoshida K, Konno C, et al. Structure of kukoamine A, a hypotensive principle of Lycium Chinense root barks. Tetrahedron Lett. 1980;21:1355–1356.10.1016/S0040-4039(00)74574-6
- Ponasik AJ, Strickland C, Faerman C, et al. Kukoamine A and other hydrophobic acylpolyamines: potent and selective inhibitors of Crithidia fasciculata trypanothione reductase. Biochem J. 1995;311:371–375.10.1042/bj3110371
- Martin-Tanguy J, Cabanne F, Perdrizet E, et al. The distribution of hydroxycinnamic acid amides in flowering plants. Phytochemistry. 1978;17:1927–1928.10.1016/S0031-9422(00)88735-X
- Martin-Tanguy J, Deshayes A, Perdrizet E, et al. Hydroxycinnamic acid amides (HCA) in zea mays distribution and changes with cytoplasmic male sterility. FEBS Lett. 1979;108:176–178.10.1016/0014-5793(79)81203-X