Abstract
In the current study, we examined the antioxidant and skin-whitening properties of Prunus mume extract (PME). The ability of PME to scavenge 2,2-diphenyl-1-picrylhydrazyl (DPPH) radicals was investigated in vitro. At a concentration of 1000 μg/mL, PME neutralized >45% free radical activity. Cell viability assessment with the 3-(4,5-dimethylthiazol-2-yl)-2,5-diphenyltetrazolium bromide (MTT) assay revealed that at concentrations <1500 μg/mL, PME does not exert cytotoxic effects on murine B16 melanoma (B16) cells. Morphological analysis disclosed that melanin production is inhibited in B16 cells treated with 250 nM α-melanocyte-stimulating hormone (α-MSH) and PME. We conclude that fruit extracts of P. mume exert a skin-whitening effect by inhibiting melanin production via regulation of melanogenesis-associated protein expression in melanocytes.
We conclude that fruit extracts of P. mume exert a skin-whitening effect by inhibiting melanin production via regulation of melanogenesis-associated protein expression in melanocytes.
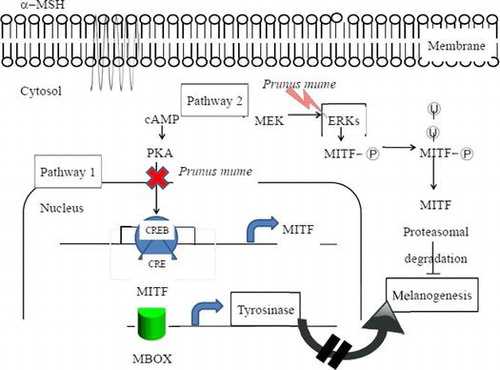
Skin color is determined by the melanin content synthesized by melanocytes in the epidermal-dermal junction. The physiological function of skin deteriorates in response to aging or extreme overproduction of melanin on the skin surface.Citation1) Melanogenesis is the process by which melanin, the dark pigment in melanocytes, is synthesized. Melanin determines skin color and protects the skin against UV light. However, abnormal accumulation of melanin can result in hyper pigmentary disorders, such as freckles, skin discoloration, and pigmented aged spots.Citation2) In mammalian melanocytes, melanin synthesis is controlled by tyrosinase, the key enzyme in pigment production. Specifically, synthesis of melanin starts from conversion of L-tyrosine to dihydroxyphenylalanine (DOPA) by tyrosinase, the enzyme catalyzing the rate-limiting step of melanin biosynthesis with O2. DOPA is oxidized to DOPA quinone.Citation3)
The majority of whitening or lightening agents act by specifically reducing tyrosinase activity through several mechanisms. For example, C2 ceramide and tretinoin suppress transcription of tyrosinase while hydroquinone and arbutin act as competitive inhibitors and linoleic acid and α-linolenic acid induce degradation of tyrosinase.Citation4) Plant extracts of Cortex Mori Radicis, Broussonetia kazinoki, Glycyrrhiza uralensis, and algae have recently been reported to inhibit melanogenesis.Citation5–7) Many synthetic skin-lightening compounds exert toxic and/or mutagenic effects, stimulating a search for novel natural agents.Citation8–10)
Maesil (Prunus mume) has long been used as a traditional drug and health food in East Asian countries. Earlier studies report that the compound has antioxidant and anti-obesity properties.Citation11,12) Maesil is regarded as an effective constituent of skin care products in Korea and used in a number of cosmetic preparations. In the melanin biosynthesis pathway, microphthalmia-associated transcription factor (MITF), tyrosinase, tyrosinase-related protein-1 (TRP-1), and tyrosinase-related protein-2 (TRP-2) contribute to the production of melanin.Citation13) Depigmentation can be accomplished by attenuating the transcription and activity of melanogenesis-related proteins, including TRP-1, TRP-2, and tyrosinase.Citation5) Limited studies to date have focused on the anti-melanogenic properties of Prunus mume extracts. To determine whether Prunus mume can be used as an effective skin-whitening agent, we assessed the antioxidant activity and inhibitory effects of Prunus mume extracts on tyrosinase activity and melanogenesis in B16-F10 melanoma cells. Immunoblot analyses were employed to examine the molecular mechanisms underlying the effects of PME on the α-MSH-stimulated melanogenesis signaling pathway investigated, including expression and activation of melanogenesis-related proteins. Based on the collective results, we propose that Prunus mume is a potential source of natural antioxidants and a whitening agent that may be valuable as a therapeutic tool for preventing or slowing the progress of aging as well as age- and oxidant stress-related degenerative diseases.
Materials and methods
Prunus mume extraction
Prunus mume was supplied by the Institute of Native Genetic Resources Inc. (Yongin, Korea). Dried powder was extracted via water ultrasonication at room temperature overnight four times, followed by filtration. The resulting extracts were freeze-dried and incubated at −20 °C until use.
Chemicals and antibodies
Dulbecco’s Modified Eagle’s medium (DMEM), Trypsin-EDTA, penicillin/streptomycin, and fetal bovine serum (FBS) were obtained from Lonza (Walkersville, MD, USA). CCK-8 assay and western blot detection kits were purchased from Dojindo Co (Japan) and Elpis Co (Daejeon, South Korea), respectively. Antibodies against MITF (cat. Sc-25386, H-50), tyrosinase (cat. Sc-15341, H-109), TRP1 (cat. Sc-25543, H-90), TRP2 (cat. Sc-25544, H-150), phosphor-Erk (cat. Sc-7383, E-4), Erk (cat. Sc-94, K-23), phosphor-Akt (cat.Sc-33437, Ser473), and Akt (cat.Sc-1618, C-20) were obtained from Santa Cruz Biotechnology (Santa Cruz, CA, USA). β-Actin was supplied by Sigma-Aldrich (USA). Antibodies against phosphor-MEK (Ser217/221, #9121), MEK (#9122), β-catenin (#9323), and horseradish peroxidase-conjugated anti-rabbit and anti-mouse secondary antibodies were purchased from Cell Signaling Technology (Danvers, MA, USA). All the other chemicals and reagents such as ascorbic acid, arbutin, and α-MSH were obtained from Sigma Aldrich.
Cell cultures and drug treatments
B16/F10 murine melanoma cells were purchased from American Type Culture Collection (ATCC, USA) and cultured in Dulbecco’s modified Eagle’s medium (DMEM) supplemented with 10% (v/v) fetal bovine serum at 37 °C in a humidified CO2-controlled (5%) incubator. Cells were seeded at the appropriate density in 24-well or 6-well plates. After one day of incubation, cells were treated with various concentrations of drugs in the absence or presence of stimulation agent (250 nM α-MSH or PME) in DMEM for 3 days, harvested and subjected to various assays.
Measurements of cell viability
Proliferation of B16/F10 murine melanoma cells was indirectly assayed using the CCK-8 kit, which stains living cells. After incubation of cells with various concentrations of PME for 48 h, CCK-8 reagent (10 μl) was added to each well and incubated at 37 °C for 3 h. Optical density at 450 nm was measured using an automatic microplate reader (Bio-Tek, Winsooski, VT, USA).
Determination of melanin content
Cells were treated with samples in DMEM containing 10% FBS for 3 days. After concentration via centrifugation [at 2000×g 4 °C], cell pellets were dissolved in 1 mL NaOH at 100 °C for 30 min and re-centrifuged for 20 min at 16,000 g. Optical densities of the supernatant fractions were measured at 475 nm using a microplate reader.
Determination of DPPH radical scavenging activity
The free radical scavenging activity of P. mume extracts, based on the activity of stable -diphenyl-2-picrylhydrazyl (DPPH) free radicals, was determined using the method described by Braca et al.Citation12) Various concentrations of P. mume extracts (0.1 mL) and 0.1 mL freshly prepared 0.2 mM DPPH solution were thoroughly mixed and maintained in the dark for 30 min. A mixture of equal amounts of methanol and DPPH served as the control. Absorbance of the reaction mixture at 517 nm was measured with a spectrophotometer (Bio-Tek, Winsooski, VT, USA).
Table 1. Concentrations of P. mume extracts and their IC50 values for DPPH inhibition.
Tyrosinase inhibition
Tyrosinase was released from melanosomes of untreated B16/F10 melanoma cells after exposure to RIPA buffer (10 mM Tris-HCl, pH 7.5, 1% NP-40, 0.1% sodium deoxycholate, 0.1% SDS, 150 mM NaCl, and 1 mM EDTA) containing protease inhibitors (CompleteTM protease inhibitor mixture, Roche, Switzerland). Lysates were centrifuged [at 2000×g 4 °C] and supernatant fractions containing soluble tyrosinase incubated with or without PME at the indicated concentrations for 1 h in reaction mixture. Tyrosinase activity was determined by measuring the amount of L-DOPA chrome produced using L-DOPA as the substrate, compared to control. The reaction mixture contained 25 mM L-DOPA (40 μL), 5 μL of 1.5 mM L-tyrosine, and 120 μl of 67 mM sodium phosphate buffer (pH 6.8), and the supernatant (80 μL). After incubation at 37 °C for at least 2 h, absorbance measured at 475 nm.
Cell morphology
Cell morphology was monitored under a light microscope. Cells were seeded in 24-well plates at a density of 2 × 10Citation4 cells/well and allowed to attach overnight. Thereafter, cells were treated with the appropriate concentrations of PME for 48 h and morphological images obtained (10× magnification).
Preparation of EB/AO stain
A 100X stock solution of Ethidium Bromide/Acridine Orange stain was prepared and frozen according to the protocol provided in the Manual of Immunological Methods.Citation14) A 1X working solution was prepared from a thawed 1 mL aliquot of 100X stock solution using the recommended protocol and stored in an amber bottle at 4 °C until use.
Protein extraction and quantification
Cells (1.5 × 107) washed with PBS were isolated via centrifugation [at 2000×g 4 °C], and proteins extracted following cell lysis. The lysis buffer solution contained 7 M urea, 2 M thiourea, 4% CHAPS, 1% DTT, 10% glycerol, 0.5% carrier ampholytes. Cells in lysis buffer were sonicated for 1 min, followed by incubation for 30 min at room temperature. After centrifugation at 4000×g for 5 min, the supernatant was collected and stored at −20 °C. The amount of total protein extracted was measured with the BCA protein assay kit (Pierce) using bovine serum albumin as the standard.
Western blotting
Cells were washed with PBS, scraped into 100 μl of lysis buffer (50 mM Tris-HCl, pH 7.4, 150 mM NaCl, 1% NP-40, 1 mM EDTA, 1 mM PMSF, 1 mM Na3VO4, 1 mM NaF, 1.1 μg/mL aprotinin, 1 μg/mL pepstatin, 1 μg/mL leupeptin supplemented with proteinase inhibitors) and solubilized for 60 min at 4 °C, followed by centrifugation [at 2000×g 4 °C] for fractionation. Proteins (30μg) were resolved by 10% SDS-PAGE, and transferred to polyvinylidene difluoride membrane (Millipore, Bedford, MA, USA). The membrane was blocked with 5% non-fat milk in PBS containing 0.1% Tween-20 for 1 h at room temperature and probed with antibodies specific for MITF, TRP-1, TRP-2, MEK, ERK, Akt, GSK3β, and β-catenin (Santa Cruz Biotechnology, Santa Cruz, CA, USA) or β-actin (Santa Cruz Biotechnology). On both cases, the membranes were stripped and reprobed with anti-β- catenin or anti-β-actin antibody. Subsequently, the membrane was incubated with HRP-conjugated secondary antibody for 1 h at room temperature. The enhanced chemiluminescence detection method was used for membrane development and protein visualization (LAS 2000, Fuji Photo Film Co Ltd, Tokyo, Japan).
Statistical analysis
All data are presented as mean ± STD. Differences among treatments were assessed by analysis of variance (ANOVA), followed by Dunnett’s test. p values < 0.05 were considered to be significant.
Results
Scavenging activity of PME on DPPH
The DPPH test, based on the ability of DPPH, a stable free radical, to decolorize in the presence of antioxidants, is a direct and reliable method for determining radical scavenging action. Ascorbic acid was selected as the reference antioxidant. Fig. () shows linear regression curves of plots of ascorbic acid standard and PME at different concentrations in relation to percentage inhibition of DPPH. The regression coefficients (R2) of the logarithmic trend line obtained for PME and standard were 0.98 and 0.34 respectively. Inhibition of DPPH was significantly low at 100 μg/mL PME (Table ), compared to all other concentrations examined. The DPPH inhibitory activity of P. mume extract was not significantly different from that of the well-known antioxidant, ascorbic acid, since the IC50 value for PME was 38.7 μg/mL and that for the ascorbic acid standard was 39 μg/mL (Table ). Scavenging of DPPH radicals was enhanced with increasing extract concentrations. However, PME concentrations above 1000 μg/mL did not induce a corresponding increase in DPPH inhibition, indicating that for optimum free radical scavenging, a concentration of 1000 μg/mL PME is adequate.
Inhibitory effects of PME on cell viability of B16-F10 melanocyte
To determine whether the inhibitory effects of PME are accompanied by possible cytotoxic effects on B16/F10 melanocytes, we treated cells with various concentrations of PME and performed the MTT assay, a sensitive, quantitative, and reliable colorimetric determinant of cell viability. Data were expressed as percentage viability relative to untreated control cells (Fig. ). PME did not exert cytotoxicity up to 1500 μg/mL, since cell viability remained higher than 95%, with no changes in cell morphology. Notably, however, at a concentration of 3000 μg/ml, viability was rapidly decreased to 50% (data not shown).
Fig. 2. Effect of PME on B16F10 cell viability.
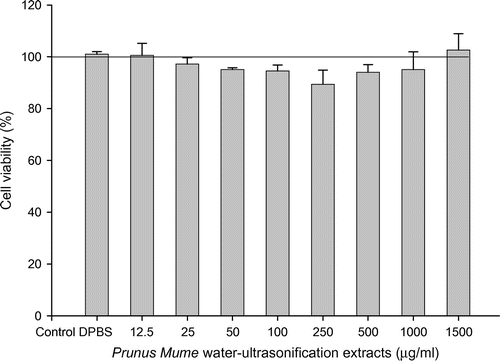
Inhibition of melanin synthesis and melanosome formation by PME
Since melanin synthesis is ultimately regulated by tyrosinase, the direct inhibitory effect of PME on tyrosinase in B16/F10 melanoma cells was examined with an intracellular tyrosinase assay. We further determined whether PME exerts an indirect effect on cellular tyrosinase activity in α-MSH-stimulated B16/F10 melanoma cells. To this end, cells were incubated with or without α-MSH and/or PME at the indicated concentrations for 72 h. Arbutin was used as the positive control because of its powerful melanosynthesis reducing activity. The melanin content induced by α-MSH was decreased to 52.9% that of control in the PME-treated (1500 μg/mL) group. The residual melanin content in arbutin-treated cells was 75.8% that of the control group (Fig. (A)). Following culture of B16/F10 melanoma cells in medium containing PME, α-MSH-stimulated tyrosinase activity was reduced by 13.2, 25.6, 45, 49.3, 65.9, and 72.7% at 50, 100, 250, 500, 1000, and 1500 μg/mL PME, respectively. PME exerted a greater inhibitory effect on tyrosinase activity than arbutin in a dose-dependent manner (Fig. (B)). While up to 72.7% inhibition occurred in response to 1500 μg/mL PME, the viability of α-MSH-treated B16/F10 melanoma cells was not affected by this concentration of PME (Fig. ). Our results demonstrate that PME inhibits melanin synthesis via suppressing cellular tyrosinase activity indirectly in α-MSH-stimulated B16/F10 melanoma cells, clearly providing evidence of PME-induced downregulation of tyrosinase expression.
Fig. 3. Effect of the PME extract on melanin production and tyrosinase activity in α-MSH-stimulated B16/F10 Melanoma cells.
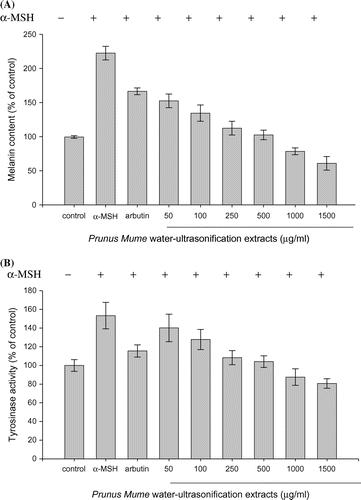
Effect of PME on morphology of α-MSH-treated B16F10 cells
Cells treated with α-MSH displayed normal morphology under a light microscope (Fig. ). AO/EB staining was used to visualize melanin synthesized in based on the formation of a brown–black pigment. Treatment of cells with PME for 72 h did not lead to significant alterations in cell morphology. However, at a dose of 1500 μg/mL, PME markedly reduced pigment formation, indicative of decreased melanin synthesis.
Fig. 4. Effects of PME on morphology of B16F10 melanoma cells treated with α-MSH.
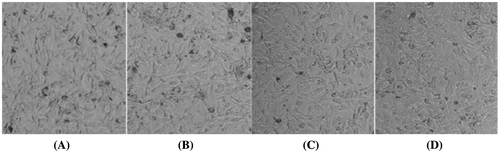
Effect of PME on the melanogenesis pathway
Immunoblot analyses were performed to investigate the effects of PME on expression of MITF, tyrosinase, TRP1, and TRP2. Cells were exposed to 250 nM α-MSH in the presence of PME or arbutin for 72 h, and the protein extracts subjected to SDS-PAGE and western blot analysis. Protein levels of MITF, tyrosinase, TRP1, and TRP2 in α-MSH-stimulated cells were significantly reduced upon PME treatment (Fig. ), consistent with PME-induced suppression of tyrosinase via downregulation of MITF, a key transcription factor of the tyrosinase gene. TRP-1 and TRP-2 are reported to regulate tyrosinase activity.Citation15) Our results indicate that PME suppresses TRP-1 and tyrosinase expression (Fig. ), in turn, inhibiting tyrosinase activity and reducing melanin production in B16F10 cells.
Fig. 5. PME inhibits the protein levels of MITF, Tyrosinase, TRP1, and TRP2.
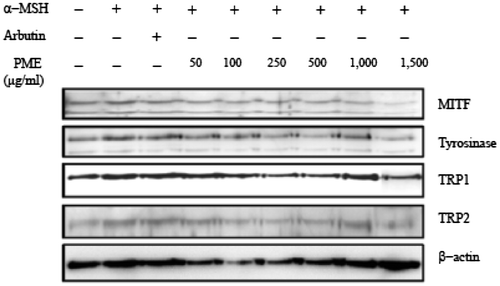
Influence of PME on inhibitory pathways of melanogenesis
Cells were treated with or without α-MSH and/or PME for 6 h and harvested. Total protein was extracted and subjected to western blot analysis. We examined the influence of PME on MEK, ERK1/2, Akt, and GSK3β levels using antibodies against phosphor-MEK (an upstream activator of ERK), phosphor-ERK1/2, phosphor-Akt, and phosphor-GSK3β, respectively. Western blot analysis revealed a marked increase in these proteins 6 h after α-MSH treatment (Fig. ), suggesting that the mechanism underlying the suppressive effect of PME on melanogenesis involves activation of ERK, pMEK, pAKT, pGSK3β kinase and β-catenin. Previous studies have suggested that inhibition of the ERK pathway and p-Akt enhances melanogenesis.Citation16,17) The common signaling pathways involved in the regulation of melanogenesis are depicted in Fig. . Prunus mume appears to exert a strong anti-melanogenesis effect. One proposed underlying mechanism is that Prunus mume suppresses CREB activation, which prevents CREV binding to the CRE motif on the MITF promoter, leading to reduced MITF expression. MITF binds to the M-Box on the tyrosinase gene promoter to a lower extent, inducing a decrease in melanogenesis (Pathway 1). Another possibility is that Prunus mume continuously activates ERKs, resulting in MITF protein degradation and a consequent decrease in melanogenesis (Pathway 2).
Fig. 6. Effects of PME on expression levels of melanogenic proteins in B16F10 melanoma cells.
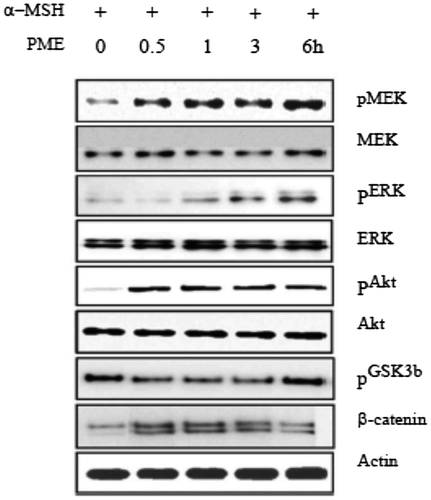
Discussion
Prunus mume has been widely cultivated as an ornamental plant in Japan, China, and Korea, and its fruit generally used as a food garnish (pickled ume) and drink (ume brandy). In particular, the flowers have been prescribed for the treatment of skin disorders, eye pain, stomach ache, as well as detoxification expectorant and sedative purposes. While a number of studies have focused on the constituents of P. mume and radical scavenging activity,Citation18) its effects on melanogenesis remain to be elucidated.
Data from the current study showed that P. mume exerts inhibitory effects on α-MSH-induced melanogenesis in B16/F10 murine melanoma cells. Moreover, the effects were dose-dependent, with no significant cytotoxicity. P. mume-induced melanin reduction was accompanied by a corresponding decrease in tyrosinase activity, which may represent a possible mechanism underlying its inhibitory action. Notably, the depigmentation effects of P. mume were stronger than those of arbutin used widely as an ingredient of whitening cosmetics, suggesting that P. mume from natural plant sources could be effectively utilized as a safe skin-whitening agent.
Melanogenesis is regulated by members of the tyrosinase gene family, including tyrosinase, TRP1, and TRP2. In melanocytes and melanoma cells, melanin production is mainly controlled by the expression and activation of tyrosinase, which catalyzes the rate-limiting step of the melanogenic process. Thus, downregulation of tyrosinase may be responsible for decreased melanin production. P. mume strongly inhibited intracellular tyrosinase activity in α-MSH-stimulated B16/F10 murine melanoma cells, as demonstrated by the cellular tyrosinase assay, suggesting that the decrease in tyrosinase activity by P. mume is not due to inhibition of enzyme activity. In addition, P. mume-mediated inhibition of tyrosinase expression was dose-dependent. However, P. mume had no effect on protein expression of TRP1 and TRP2, which act downstream of tyrosinase in the melanin biosynthetic pathway. These results are in concurrence with an earlier report demonstrating that pyrroloquinoline quinone (PQQ) inhibits tyrosinase expression in α-MSH-stimulated B16 melanoma cells in a dose-dependent manner, but not TRP1 and TRP2 expression.Citation19) Our findings clearly indicate that P. mume inhibits tyrosinase expression at the transcriptional level, resulting in downregulation of melanin production.
On the basis of these results, we further investigated the signaling pathway underlying transcriptional downregulation of the tyrosinase gene by P. mume in α-MSH-stimulated B16/F10 murine melanoma cells (Fig. ). P. mume inhibited protein expression of MITF, a major transcription regulator of tyrosinase that plays a critical role in melanin biosynthesis, in a dose-dependent manner.
Previous studies have suggested that the ERK signaling pathway is involved in the regulation of melanogenesis. ERK activation induces phosphorylation of MITF at serine 73, leading to its ubiquitination, followed by proteasome-mediated degradation. Moreover, sustained activation of ERK by C2 ceramide and sphingosine-1-phosphate (S1P) is reported to trigger MITF phosphorylation and subsequent degradation, resulting in reduced tyrosinase and melanin levels. Consistent with these reports, our experiments demonstrated that P. mume induces reduction of tyrosinase and melanin levels as well as prolonged ERK activation.
cAMP response element binding (CREB) phosphorylation by the cAMP pathway induces MITF expression and subsequent upregulation of tyrosinase, leading to increased melanin synthesis. α-MSH is known to induce CREB phosphorylation and MITF expression. The promotory effects of α-MSH on melanogenesis are mediated through activation of the cAMP pathway and PKA. Our results demonstrated that P. mume inhibits CREB phosphorylation and MITF expression in α-MSH-stimulated B16 melanoma cells. The ERK signaling pathway is activated during cAMP-induced melanogenesis in B16 melanoma cells, indicating the involvement of a complex network of signaling pathways in the regulation of melanin synthesis.
Here, we have demonstrated that P. mume induces downregulation of melanogenesis through ERK activation and decreased CREB phosphorylation, leading to reduction of MITF expression, and consequently, tyrosinase expression in α-MSH-stimulated B16 melanoma cells. Further studies are required to elucidate the precise mechanisms involved in downregulation of MITF expression by P. mume.
In conclusion, the P. mume extract (PME) appears to be involved in various physiological activities via DPPH scavenging activity. Treatment with PME led to inhibition of tyrosinase expression in B16F10 mouse melanoma cells. This inhibitory effect of PME was attributed to decreased MITF transcription, since PME induced a significant decrease in transcriptional activity of the MITF promoter in α-MSH-induced B16F10 cells. Our data suggest for the first time that PME exerts anti-melanogenesis effects via inhibition of CREB and maintenance of ERK activation. This finding is of significant cosmeceutical importance for designing novel tanning products with the potential to reduce skin cancer risk.
Author contributions
KB Pi and KB Lee conceived and designed the study. KB Pi performed the experiments. KB Lee analyzed the data, wrote the manuscript, reviewed and edited the manuscript.
Disclosure statement
The authors do not state any potential conflict of interest.
Funding
This work was supported by a grant of the 3G-Bio based Eco-Biomaterial R&D Project, Ministry of Knowledge Economy, Republic of Korea
References
- Hill HZ, Li W, Xin P, et al. Melanin: a two edged sword? Pigment Cell Res. 1997;10:158–161.10.1111/pcr.1997.10.issue-3
- Curto EV, Kwong C, Hermersdörfer H, et al. Inhibitors of mammalian melanocyte tyrosinase: in vitro comparisons of alkyl esters of gentisic acid with order putative inhibitors. Biochem Pharmacol. 1999;57:663–672.10.1016/S0006-2952(98)00340-2
- Sulaimon SS, Kitchell BE. The biology of melanocytes. Vet Dermatcol. 2003;14:57–65.10.1046/j.1365-3164.2003.00327.x
- Parvez S, Kang MK, Chung HS, et al. Survey and mechanism of skin depigmenting and lightening agents. Phytotherapy Res. 2006;20:921–934.10.1002/(ISSN)1099-1573
- Briganti S, Camera E, Picardo M. Chemical and instrumental approaches to treat hyperpigmentation. Pigment Cell Res. 2003;16:101–110.10.1034/j.1600-0749.2003.00029.x
- Zhong S, Wu Y, Ahn SM, et al. Depigmentation of melanocytes by the treatment of extracts from traditional Chinese herbs: a cell culture assay. Bio Pharm Bull. 2006;29:1947–1951.10.1248/bpb.29.1947
- Lee SH, Choi SY, Kim H, et al. Mulberroside F isolated from the leaves of Morus alba inhibits melanin biosynthesis. Biol Pharm Bull. 2002;25:1045–1048.10.1248/bpb.25.1045
- Jung GD, Yang JY, Song ES, et al. Stimulation of melanogenesis by glycyrrhizin in B16 melanoma cells. Exp Mol Med. 2001;33:131–135.10.1038/emm.2001.23
- No JK, Soung DY, Kim YJ, et al. Inhibition of tyrosinase by green tea components. Life Sci. 1999;65:241–246.
- Daozong X, Jiayi S, Jinyan G, et al. Antioxidant activity of Chinese mei (Prunus mume) and its active phytochemicals. J Med Plant Res. 2010a;4:1156–1160.
- Daozong X, Xiaoqin W, Qing Y, et al. Anti-obesity and hypolipidemic effects of a functional formula containing Prumus mume in mice fed high-fat diet. Afr J Biotechnol. 2010b;9:2463–2467.
- Braca A, De Tommasi N, Di Bari L, et al. Antioxidant principles from Bauhinia tarapotensis. J Nat Prod. 2001;64:892–895.10.1021/np0100845
- Hearing VJ, Jiménez M. Mammalian tryosinase-the critical regulatory control point in melanocyte pigmentation. Int J Biochem. 1987;19(12):1141–1147.10.1016/0020-711X(87)90095-4
- Wan P, Hu Y, He L. Regulation of melanocyte pivotal transcription factor MITF by some other transcription factors. Mol Cell Biochem. 2011;354:241-246.
- Cabanes J, Chazarra S, Garcia-Carmona F. Kojic acid, a cosmetic skin whitening agent, is a slow-binding inhibitor of catecholase activity of tyrosinase. J Pharm Pharmacol. 1994;46:982–985.10.1111/jphp.1994.46.issue-12
- Cunha ES, Kawahara R, Kadowaki MK, et al. Melanogenesis stimulation in B16-F10 melanoma cells induces cell cycle alterations, increased ROS levels and a differential expression of proteins as revealed by proteomic analysis. Exper Cell Res. 2012;318:1913–1925.10.1016/j.yexcr.2012.05.019
- Li HH, Su JH, Chiu CC, et al. Proteomic investigation of the sinulariolide-treated melanoma cells A375: effects on the cell apoptosis through mitochondrial-related pathway and activation of caspase cascade. Mar Drugs. 2013;11:2625–2642.10.3390/md11072625
- Hsieh JF, Chen ST, Cheng SL. Molecular profiling of A375 human malignant melanoma cells treated with kojic acid and arbutin. Breakthroughs Melanoma Res. 2011-11; Book Chapter:533–558.
- Racay P, Kaplan P, Lehotsky K. Control of Ca2+ homeostasis in neuronal cells. Gen Physiol Biophys. 1996;15:193–210.