Abstract
Previous studies have shown that some polyphenols have anti-ice nucleation activity (anti-INA) against ice-nucleating bacteria that contribute to frost damage. In the present study, leaf disk freezing assay, a test of in vitro application to plant leaves, was performed for the screening of anti-INA, which inhibits the ice nucleation activity of an ice-nucleating bacterium Erwinia ananas in water droplets on the leaf surfaces. The application of polyphenols with anti-INA, kaempferol 7-O-β-glucoside and (–)-epigallocatechin gallate, to the leaf disk freezing assay by cooling at −4–−6 °C for 3 h, revealed that both the compounds showed anti-INAs against E. ananas in water droplets on the leaf surfaces. Further, this assay also revealed that the extracts of five plant leaves showed high anti-INA against E. ananas in water droplets on leaf surfaces, indicating that they are the candidate resources to protect crops from frost damage.
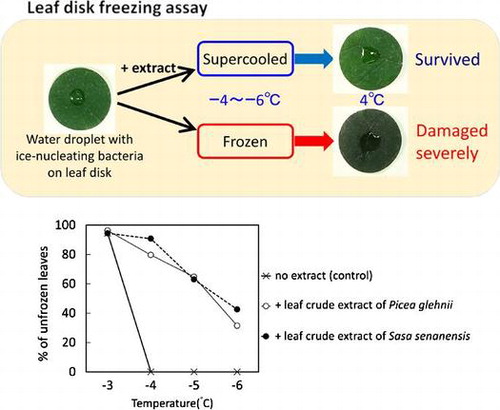
Water droplets with ice-nucleating bacteria on leaf disks were hardly frozen by addition of leaf crude extracts. Unfrozen leaf disks survived freezing injury.
Spring frost is a limiting factor for the distribution of temperate or subarctic trees; therefore, freezing resistance in plants is physiologically important during early springCitation1). After budbreak in spring, seedlings or young leaves and flowers of trees become susceptible to frost damage because freezing resistance gradually decreases owing to seasonal deacclimation. When ambient temperatures in spring are below 0 °C in the fields, plants with ice nucleators may be frosted, and the plants with low freezing resistance might experience frost damage.
The freezing of water is affected by ice nucleators and substances with anti-ice nucleation activity (anti-INA). Ice nucleators such as silver iodide (AgI), phloroglucinol, and ice nucleation proteins produced by ice-nucleating bacteria (e.g. Erwinia, Pseudomonas, and Xanthomonas spp.), promote ice nucleus formation, which rises the freezing temperature of water. Conversely, substances with anti-INA promote supercooling by inhibiting the ice nucleation activity (INA), resulting in a decline in the freezing temperature of water. Furthermore, some anti-freeze proteinsCitation2,3), polysaccharidesCitation4), terpenoidsCitation5), phenylpropanoidsCitation6), flavonol glycosidesCitation7–9), and tanninsCitation10,11) show anti-INA. The anti-INA of 40 flavonoids was previously measured in solutions containing ice nucleators, such as Erwinia ananas or AgI. The intensities of the anti-INA have been reported to depend on the flavonoid types, and several flavonoids have been found to demonstrate sufficient activity, which decrease the freezing temperature over 2 °C, in solutions containing E. ananas. Anti-INA may be influenced by both aglycone types and modifications in their structuresCitation9,12). Plants accumulate various flavonoids and their glycosides as secondary metabolites. The compositions of the accumulated flavonoids depend on various factors, such as plant species, tissues, seasons, and environmental conditions. Some flavonoids and their derivatives show antioxidative, antimicrobial, and/or antifungal activities and are used in the defense systems of plantsCitation13–15). Thus, certain flavonol glycosides may act as multifunctional compounds for defense, including protection from frost damage caused by the bacterial ice nucleation.
Frost damage is an agricultural problem that reduces the productivity and commodity values of crops. In the fields of tea tree, fruit tree (e.g. apple and grape), and vegetable (e.g. cabbage, lettuce, and asparagus), costs and efforts are needed to prevent the frost damage. The presence of ice-nucleating bacteria, which express ice nucleation proteins on cellular surfaces, is a possible factor that induces frost damage in plantsCitation16,17). In the field, crops may be protected by blower fan or heating against the decline of air temperatures near the soil. Further, the exogenous application of substances with anti-INA to crops suppresses the freezing initiated by ice nucleators on plant surfaces; this may be useful to prevent the frost damage. As a roadmap to the field application, the in vitro application of the polyphenols with anti-INA to crop leaves (leaf disks or segments) and crop seedlings in the pots may be convenient and bring useful information. However, no reports regarding the in vitro application of these polyphenols to the crops were found. Therefore, in the present study, a more convenient method, leaf disk freezing assay, was examined for the screening of anti-INA among various plants in the laboratory, as the preliminary evaluation before field applications.
To estimate anti-INA by droplet freezing assay, small water droplets (2–10 μL per droplet) containing ice nucleator and/or polyphenols with anti-INA were cooled on a plate or in tubes to subzero temperatures at a constant cooling rate, and the number of frozen droplets were counted. In the present study, to study the preventing effect of anti-INA on frost damage of crop leaves by in vitro application, small water droplets (10 μL per droplet) containing ice-nucleating bacteria and/or polyphenols with anti-INA were cooled on leaf disks or segments of the crop. This new bioassay system, “leaf disk freezing assay,” using crop leaves resembles the frosting process of leaves by bacterial ice nucleators in the field. Freezing of water is influenced by the surface characteristics. The surface condition of leaves is dependent upon each crop. Therefore, the condition of the surface where water attaches is one of the important factors for freezing of water. The leaf disk freezing assay is considered to be usable for the primary or secondary screening of plant resources with anti-INA at a laboratory scale before screening with seedlings or its application in an agricultural field.
Materials and methods
Materials and preparation of leaf extracts
For the preparation of leaf extracts, leaves of various plants grown on the campus of Hokkaido University (Sapporo, Japan) were sampled from June to September 2015 (Table ). Approximately 2 g [fresh weight (FW)] of leaves were milled in liquid nitrogen using a mortar and a pestle and extracted with 20 mL of solvent (acetone:water = 1:1). The mixture was filtered using Celite and, subjected to evaporation at 30 °C to remove the acetone, and the lyophilized extracts were obtained. The lyophilized extracts were suspended in water to a concentration of 0.20-g fresh weight per mL (0.20 g FW/mL). The suspension was centrifuged at 15,000 g for 10 min at 20 °C, and the supernatant was used in the assay described below.
Table 1. Anti-ice nucleation activity (anti-INA) of leaf extracts obtained from 65 plants in the presence of the ice-nucleating bacteria E. ananas by droplet freezing assay.
Osmotic concentration of the supernatants of the extract was measured by vapor pressure osmometer (model 5520; Wescor Inc.; Utah, USA) to estimate the value of freezing point depression.
Preparation of stock solutions of ice nucleators
For the assay of anti-INAs, ice-nucleating bacteria E. ananas TM2 (MAFF811106) was cultured according to the method of Kuwabara et al.Citation9) Bacterial cells harvested by centrifugation were suspended in water to a concentration of 10 mg/mL and stored at 4 °C for 2 d before the assay. Phloroglucinol (Tokyo Chemical Industry Co., Ltd.; Tokyo, Japan) was dissolved in water to a concentration of 0.48 M. The stock solution of AgI was freshly prepared by mixing the same volumes of 20 mM potassium iodide (Wako Pure Chemical Industries, Ltd.; Osaka, Japan) and 20 mM silver nitrate (Nacalai Tesque; Kyoto, Japan) solutions just before the assay.
Droplet freezing assay
The freezing temperature of the solution was measured by a droplet freezing assay, as described in our previous reportCitation12), which was slightly modified from the assay developed by ValiCitation18). Two μL aliquots of the sample solution containing 0.10 g FW/mL leaf extract, 2.0 mg/mL ice-nucleating bacteria, and 50 mM potassium phosphate buffer (pH 7.0) were loaded on the copper plate coated with mineral oil (Nacalai Tesque; Kyoto, Japan). The copper plate was placed in an ethylene glycol bath (FP40; Julabo GmbH; Baden-Württemberg, Germany) pre-chilled at 0 °C and cooled at a rate of 0.2 °C/min. The number of frozen droplets was counted at every 0.5 °C. In each assay, 20 droplets of each sample and the control solution without leaf extract were loaded on the same copper plate, and the assay was repeated five times or more under the same conditions for reproducibility. FT50, the temperature at which half of the droplets (10 droplets) are frozen, was calculated in each assay. The mean and standard deviation of the differences in FT50 between control and sample solutions were calculated. When FT50 of the sample solution was lower than that of the control solution, the difference in FT50 temperature was positive, implying that the sample solution had anti-INA.
In this study, the extracts with relatively high anti-INA in the presence of ice-nucleating bacteria were also used for measuring anti-INA using 0.12 M phloroglucinol or 2.5 mM AgI as an ice nucleator instead of ice-nucleating bacteria.
Leaf disk freezing assay
To simulate the freezing of water on the leaf surface by bacterial ice nucleation, a droplet freezing assay was performed using leaf disks instead of copper plates. Four plants [radish seedlings (Raphanus sativus var. longipinnatus), maize seedlings (Zea mays cv. Honey Bantam), mulberry trees (Morus bombycis), and tea trees (Camellia sinensis cv. Saemidori)] were selected, and the leaves were prepared as described below.
Radish seeds were sown on vermiculite and grown for 5 d at 23 °C (16 h in light of 70 μmol/m2/s and 8 h in the dark). Cotyledons were used for the assay. Maize seedlings were grown in a greenhouse for approximately 3 months after sowing, and leaf disks (φ, 10.5 mm) were prepared using an eyelet punch. Mature mulberry leaves were harvested from July to September from adult trees growing on the campus of Hokkaido University, and leaf disks (φ, 15 mm) were prepared using an eyelet punch. Seedlings of tea trees were cultured in the greenhouse for over 5 months. Mature leaves were harvested from young tea trees and leaf disks (φ, 9 mm) were prepared using an eyelet punch. For preparing leaf samples (cotyledons and leaf disks), all leaves were washed after harvesting and blotted using a paper towel to remove water from the leaf surfaces. The abaxial surfaces of ten leaf disks were placed on a polystyrene Petri dish (φ, 90 mm; thickness, 15 mm) and fixed by double-faced tape. Nine dishes were prepared for each assay. An aliquot of 10 μL of the sample solution was loaded on the adaxial surfaces of leaf disks. The sample solution containing 1.0 mg/mL ice-nucleating bacteria (E. ananas), 50 mM potassium phosphate buffer (pH 7.0), and two previously reported polyphenols with high anti-INA—1.0 mg/mL (−)-epigallocatechin gallate (EGCG) (Sunphenon EGCG; Taiyo Kagaku Co., Ltd.; Mie, Japan) or 0.10 mg/mL kaempferol 7-O-β-glucoside (K7G) (EXTRASYNTHESE; Genay, France)—were used for confirming the availability of this assay system. And, 0.10 g FW/mL high anti-INA leaf extract was used for confirming the effectivity of leaf extract to frost damage prevention. The Petri dishes were covered with lids, placed in a programable freezer pre-chilled at 0 °C for 30 min and then cooled to the objective temperatures of −3, −4, −5, and −6 °C at a rate of 0.2 °C/min. After being kept at an objective temperature for 3 h, the percentage of frozen droplets was calculated to evaluate anti-INA. The assay was repeated at least five times at each temperature.
After this assay, we measured the cell survival rate of typical leaf segments that were frozen and unfrozen at each temperature. Droplets on leaf segments were removed, and the survival rate was measured by electrolyte leakage methodCitation19) with minor modifications.
Results and discussion
Screening of leaf extracts with high anti-ice nucleation activity (anti-INA) by droplet freezing assay
Since a massive amount of polyphenols with anti-INA are necessary for the field application, a significant cost will be incurred in using purified polyphenols as the substances with anti-INA. Therefore, screening leaf extracts with high anti-INA as plant resources was examined for an economic advantage because using extracts containing substances with anti-INA may lower the cost for field applications compared to the purified compounds. To screen numerous samples, the droplet freezing assay was done for convenience.
Sixty-five leaf extracts (22 woody plants from 19 families and 43 herbaceous plants from 23 families) were chosen from various families of woody and herbaceous plants for the droplet freezing assay to select the plant resource candidates for field applications. Dry matter of leaf extracts was prepared, and anti-INA of the leaf extracts at the concentration of 0.10 g FW/mL were measured by the droplet freezing assay with E. ananas, at a final concentration of 2.0 mg/mL in a 2-μL aqueous solution. Further characterization of the extracts with high anti-INA with E. ananas was conducted by measuring anti-INA values in the presence of two other ice nucleators AgI or phloroglucinol. In this assay system, the freezing temperatures of the control solutions with E. ananas, AgI, and phloroglucinol were −4.7 ± 0.2 °C, −3.9 ± 0.3 °C, and −7.4 ± 0.6 °C, respectively. The osmotic concentration of all the extracts at 0.10 g FW/mL was so low (below 0.1 Osmol/kg) that they could not be measured (data not shown). Accordingly, the value of freezing point depression by these extracts was so low that it could not be measured by the droplet freezing assay.
The leaf extracts examined in this study showed various levels of anti-INAs. In particular, the leaf extracts of Picea glehnii, Sasa senanensis, Salix bakko, Laurus nobilis, Citrus sudachi, Perilla frutescens var. crispa f. purpurea, Camellia sinensis cv. Saemidori, and Taraxacum officinale showed relatively high anti-INA (>2.5 °C) (Table ). Two-thirds of the 65 plants showed moderate (approximately 2 °C) or low (around 1 °C or less) anti-INA and some showed no anti-INA. The low contents of the substances with anti-INA or coexistence of substances with high INA or possible inhibitors of anti-INA may have been a reason for the absence or low level of anti-INA in the extracts. In addition, few or no anti-INA in the leaf extracts may be dependent on the types and concentrations of polyphenols with anti-INA. Thus, there is a possibility that the substances with high anti-INA are purified even from the extracts with relatively low anti-INA (<2 °C). It has been reported that the anti-INA of pea seed extracts (0.05 g FW/mL) were approximately 4 °C in the presence of AgI in a 10-μL aqueous solutionCitation20). Anti-INA values of xylem tissue extracts of twigs of six hardwood species on a basis of 100 mOsmol/kg were ≤2 °C in the presence of E. ananas at a final concentration of 2 mg/mL in 2-μL droplets of aqueous solutionCitation21). Further studies have shown that four types of flavonol glucosidesCitation7) and four types of hydrolyzable tanninsCitation10), with anti-INA values ranging from 2–9 °C, were isolated from the xylem tissue extracts of katsura, with the highest activity (approximately 2 °C) observed among six species at concentrations of 100 mOsmol/kg. Further studies have revealed that many compounds, categorized into polyphenols (flavonoid glycosides, hydrolyzable tannins, catechin derivatives, etc.), showed various levels of anti-INA in the solutions containing ice nucleators, such as AgI or E. ananasCitation9,11). The freezing temperature of the aqueous solution is influenced by various conditions (e.g. volume and composition of assay mixture, types and concentrations of ice nucleator and substances with anti-INA, cooling rates, and purity of water). Thus, a simple comparison of anti-INA detected in this study with those reported earlier was complicated by the minor differences in assay conditions, such as types of ice nucleators or sample volumes in each report and the difference in the sample (compositions and concentrations of extracts) prepared in each study (Table 3 of Kasuga et al.Citation7)).
The leaf extracts of the 11 plants with high anti-INA were further characterized using different ice nucleators of AgI or phloroglucinol instead of E. ananas to study their potential of anti-INA (Table ). All the extracts showed high anti-INA values (>6 °C) in the presence of 2.5 mM AgI as an ice nucleator. Furthermore, the extracts from P. glehnii, S. senanensis, S. bakko, L. nobilis, and C. sinensis showed relatively high anti-INA values (>7 °C) in the presence of 0.12 M phloroglucinol as an ice nucleator, and those from Brassica juncea (red mustard), C. sudachi, P. frutescens, T. officinale, Crinum latifolium, and B. juncea spp. showed relatively low anti-INA values (approximately 2 °C) in the presence of 0.12 M phloroglucinol. The anti-INA levels against different ice nucleators were also dependent on plant species. Further, the different levels of anti-INA could also be attributed to the different compositions or the concentrations of substances with anti-INA in plants. As shown in our previous studies, anti-INAs were also detected in the commercially available mixtures of polyphenolsCitation11). Therefore, extracts may contain various types of substances with anti-INA with different degrees of activity toward ice nucleators.
Table 2. Anti-ice nucleation activity (anti-INA) of leaf extracts in the presence of different types of ice nucleators by droplet freezing assay.
Examination of leaf disk freezing assay for anti-ice nucleation activity (anti-INA)
Before applying leaf extracts with anti-INA to plants grown in the pots and fields, confirming the availability of leaf extracts by in vitro assay system using leaf disks or segments is significant for saving efforts and costs. A simple assay using leaf disks or segments was examined in the present study.
The droplet freezing assay has shown that anti-INAs of kaempferol 7-O-β-glucoside (K7G) and (–)-epigallocatechin gallate (EGCG) were 5.7 and 3.3 °C, respectively, in the previous studyCitation11,12). They were used as positive controls for the examination of leaf disk freezing assay system. Plant leaves used in this assay were randomly selected by easy preparation of seedlings or harvesting leaves from the field. Ten-μL droplets of the mixture containing ice-nucleating bacterium E. ananas and K7G or EGCG with high anti-INA were placed on the surface of leaf disks of mulberry, tee tree, and maize and that of cotyledons of radish seedlings and then cooled at subzero temperatures (−3, −4, −5, or −6 °C) for 3 h in a programable freezer. To confirm that E. ananas possess INA on leaf segments, the droplets containing phosphate buffer alone were used as a control. As a result, E. ananas promoted the freezing of droplets on the leaf segments (Supplemental Fig. 1) and confirmed that E. ananas possesses INA on leaf segments.
Almost all samples containing E. ananas and polyphenols with high anti-INA (K7G or EGCG) and the controls containing E. ananas and no polyphenols were not frozen on leaf surfaces by cooling at −3 °C for 3 h (Fig. ). Further, by cooling at −4 °C for 3 h, all the controls containing E. ananas and no polyphenols were frozen on leaf surfaces, but >80% of the samples containing E. ananas and polyphenols were unfrozen (in the supercooling state) (Fig. ). The rate (%) of unfrozen samples containing E. ananas and polyphenols gradually decreased as the treatment temperature declined. In the present study, leaf segments used were lethally damaged by freezing of water droplets on the leaves at −3 to −6 °C but not by cooling (temperature decline) at −3 to −6 °C when survival rates of leaf segments after the freezing tests were measured by electrolyte leakage method (Supplemental Fig. 2). By slow cooling, water droplets with E. ananas on the leaf disks began to freeze at −3–−4 °C on the leaf surfaces and then extracellular freezing occurred inside leaves. Most water droplets frozen at −4–−6 °C promoted extracellular freezing of apoplastic water in the leaf disks. K7G and EGCG with anti-INA inhibited freezing of water droplets caused by INA of E. ananas in the droplets on the leaf surfaces at −4–−6 °C, resulting in maintaining unfrozen state in the leaf. The application of K7G or EGCG resulted in survival of leaves at subzero temperatures. Further, the anti-INA of K7G on leaf surface was higher than that of EGCG. These results suggested that the in vitro assay system of anti-INA using leaf disks or segments can be used for the screening of anti-INA and that it is possible that the application of polyphenols with anti-INA to leaves protects freezing-induced damages at relatively high subzero temperatures.
Fig. 1. Anti-ice nucleation effects of polyphenols with anti-INA on cooling at subzero temperatures. Freezing curves of 10 μL of droplets containing polyphenols [1.0 mg/mL (−)-epigallocatechin gallate (EGCG), 0.10 mg/mL kaempferol 7-O-β-glucoside (K7G) or nothing (control)] and 1.0 mg/mL Erwinia ananas and phosphate buffer at subzero temperature (−3–−6 °C) on mulberry leaf disks (A), radish cotyledon (B), maize leaf disks (C), and tea leaf disks (D) were compared. The assay was repeated at least five times at each temperature, and the mean value of unfrozen droplets and leaves was calculated. X-mark, control; open diamond, EGCG; filled diamond, K7G.
![Fig. 1. Anti-ice nucleation effects of polyphenols with anti-INA on cooling at subzero temperatures. Freezing curves of 10 μL of droplets containing polyphenols [1.0 mg/mL (−)-epigallocatechin gallate (EGCG), 0.10 mg/mL kaempferol 7-O-β-glucoside (K7G) or nothing (control)] and 1.0 mg/mL Erwinia ananas and phosphate buffer at subzero temperature (−3–−6 °C) on mulberry leaf disks (A), radish cotyledon (B), maize leaf disks (C), and tea leaf disks (D) were compared. The assay was repeated at least five times at each temperature, and the mean value of unfrozen droplets and leaves was calculated. X-mark, control; open diamond, EGCG; filled diamond, K7G.](/cms/asset/740a1124-9ac7-4111-ba09-4647738e89b5/tbbb_a_1373587_f0001_b.gif)
Reduction of freezing damage to leaves using anti-INA in leaf extracts
As mentioned above, for applying substances with anti-INA to agricultural fields, high anti-INA of leaf extracts were examined for their potential to prevent leaves from frost damage by the in vitro leaf disk freezing assay. For this experiment, five plant extracts with high anti-INA, which can be prepared easily and massively, were selected: P. glehnii, S. senanensis, L. nobilis, C. sinensis, and T. officinale.
Most of the control droplets containing E. ananas alone (without extracts) and the sample droplets containing E. ananas and five leaf extracts with high anti-INA were hardly frozen at −3 °C on each of the leaf surfaces for 3 h (Fig. ). At −4 °C, >90% of both the control droplets containing E. ananas alone and negative control droplets containing E. ananas and Portulaca oleracea leaf extract with no anti-INA were frozen on each of the leaf surfaces; however,>80% of the sample droplets containing E. ananas and leaf extracts with high anti-INA were not frozen on each of the leaf surfaces. This result was quite similar to that of the positive controls containing E. ananas and K7G or EGCG (Fig. ). These results suggested that anti-INA of the leaf extracts in the sample droplets partly reduced the INA of E. ananas on leaf surfaces. At −5 °C, almost all control droplets containing E. ananas alone and the negative control droplets containing E. ananas and P. oleracea extract with no anti-INA were frozen on each of the leaf surfaces (Fig. ). Further, more than 50% (50%–90%) of the sample droplets containing E. ananas and the leaf extracts with high anti-INA were not frozen on each of the leaf surfaces (Fig. ). At −6 °C, the proportions of frozen sample droplets containing E. ananas and leaf extracts with high anti-INA increased. The increase in the proportion of frozen samples was dependent on the temperature and plant species used for the leaf segments. Droplets of the leaf extracts with high anti-INA and the positive control were more difficult to freeze on the surface of maize leaves and radish cotyledons than those on mulberry and tea leaves. These results show that the structural features of leaf surfaces may influence the ice nucleation of sample droplets to some extents. The freezing damage of leaf segments used here is similar to the case of K7G or EGCG, suggesting that freezing but not cooling at −4–−6 °C lethally damaged leaf samples. This was confirmed by the electrolyte leakage method (Supplemental Fig. 2).
Fig. 2. Anti-ice nucleation effects of leaf extracts with anti-INA on cooling at subzero temperatures. Freezing curves of 10 μL of droplets containing 0.10 g FW/mL leaf extracts (sample solution) or nothing (control) and 1.0 mg/mL Erwinia ananas and phosphate buffer at subzero temperature (−3–−6 °C) on mulberry leaf disks (A), radish cotyledon (B), maize leaf disks (C), and tea leaf disks (D) were compared. The assay was repeated at least five times at each temperature, and the mean value of unfrozen droplets and leaves was calculated. X-mark, control; open circle, Picea glehnii; filled circle, Sasa senanensis; open triangle, Laurus nobilis; filled triangle, Camellia sinensis cv. Saemidori; open square, Taraxacum officinale; filled square, Portulaca oleracea.
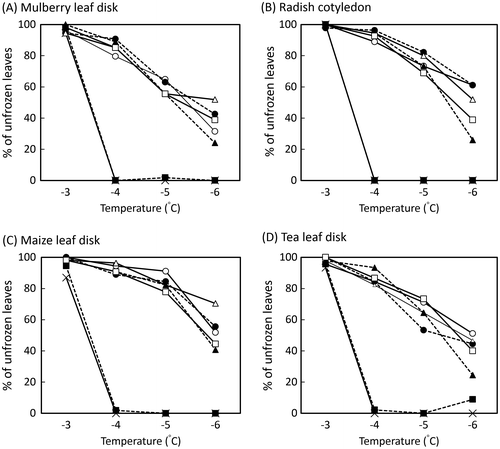
Although minor differences were observed in the sensitivity to ice nucleation, similar results were obtained by the leaf disk freezing assay using E. ananas and leaf extracts in water droplets on various leaf disks. Thus, results of leaf disk freezing assay clearly suggest that anti-INA of the leaf extracts effectively reduce the INA of E. ananas on various leaf surfaces (Fig. , Supplemental Fig. 1). Therefore, frost injury caused by ice-nucleating bacteria may be reduced by exogenous use of the leaf extracts with high anti-INA.
The mixtures of polyphenols with anti-INA may reduce ice nucleation to a higher extent than a single polyphenol with anti-INA for adaptation to various situations of ice nucleation. Therefore, plant extracts that can inhibit various types of ice nucleators may be used as cryoprotectants, protecting agricultural products from frost damage in the field as well as the hydrophobic kaolin particle filmCitation22), polyethylene glycolCitation23), chitosanCitation24), or zeoliteCitation25). In addition, field application of plant extracts with anti-INA may be more convenient in terms of costs than that of the purified substances.
Acylated kaempferol glycosides, stilbene glucosides, and flavone glycosides, whose structures are similar to those of glycosylated polyphenols with anti-INA, have been reported in L. nobilisCitation26), P. glehniiCitation27), and S. senanensisCitation28), respectively. Similar compounds can be obtained from various plant extracts, and extracts containing these compounds can be candidates for frost damage prevention. Further characterization of extracts with high anti-INA is necessary to identify the substances with anti-INA and/or to utilize anti-INA of polyphenols isolated from these extracts.
In the present study, leaf disk freezing assay, an in vitro application system, was examined for evaluation of anti-ice nucleation effects on leaves. Actually, anti-ice nucleation effects were clearly shown on the leaf segments using this assay system as well as the screening of polyphenols and leaf extracts with high anti-INA by the droplet freezing assay. After these experiments, field applications may be examined.
Supplemental data
Supplemental data for this article can be accessed at https://doi.org/10.1080/09168451.2017.1373587.
Funding
This work was partially supported by Japan Society for the Promotion of Science under a Grant-in-Aid for Scientific Research (KAKENHI) [grant number 15H04615 (K.A.)], [grant number 15K14667 (Y.F.)]
Disclosure statement
No potential conflict of interest was reported by the authors.
Author contribution
YF and KA conceived the project. SS, YF, and KA designed the experiments. SS and SF performed the experiments. SS, YF, and KA contributed to the discussions and wrote the manuscript.
Figs_S1_S2_TBBB_1373587.docx
Download MS Word (449.8 KB)Acknowledgment
The authors would like to thank Enago (www.enago.jp) for the English language review.
Some leaf samples for extracts were provided by Field Science Center for Northern Biosphere, Hokkaido University.
The authors would like to express sincere gratitude to Dr. Seizo Fujikawa, Emeritus Professor of Hokkaido University, for his useful comments.
References
- Sakai A, Larcher W. Frost survival of plants: responses and adaptation to freezing stress. Berlin: Springer-Verlag; 1987.10.1007/978-3-642-71745-1
- Wilson PW, Leader JP. Stabilization of supercooled fluids by thermal hysteresis proteins. Biophys J. 1995;68:2098–2107.10.1016/S0006-3495(95)80389-9
- Duman JG. The inhibition of ice nucleators by insect antifreeze proteins in enhanced by glycerol and citrate. J Comp Physiol B. 2002;172:163–168.
- Yamashita Y, Kawahara H, Obata H. Identification of a novel anti-ice-nucleating polysaccharide from Bacillus thuringiensis YY529. Biosci Biotechnol Biochem. 2002;66:948–954.
- Kawahara H, Masuda K, Obata H. Identification of a compound in Chamaecyparis taiwanensis inhibiting the ice-nucleation activity of Pseudomonas fluorescens KUIN-1. Biosci Biotechnol Biochem. 2000;64:2651–2656.
- Kawahara H, Obata H. Identification of compound in spices inhibiting the ice-nucleating activity of Erwinia uredovora KUIN-3. J Antibact Antifung Agents. 1996;24:95–100.
- Kasuga J, Hashidoko Y, Nishioka A, et al. Deep supercooling xylem parenchyma cells of katsura tree (Cercidiphyllum japonicum) contain flavonol glycosides exhibiting high anti-ice nucleation activity. Plant Cell Environ. 2008;31:1335–1348.10.1111/pce.2008.31.issue-9
- Kasuga J, Fukushi Y, Kuwabara C, et al. Analysis of supercooling-facilitating (anti-ice nucleation) activity of flavonol glycosides. Cryobiology. 2010;60:240–243.10.1016/j.cryobiol.2009.12.004
- Kuwabara C, Wang D, Kasuga J, et al. Freezing activities of flavonoids in solutions containing different ice nucleators. Cryobiology. 2012;64:279–285.10.1016/j.cryobiol.2012.02.012
- Wang D, Kasuga J, Kuwabara C, et al. Presence of supercooling-facilitating (anti-ice nucleation) hydrolyzable tannins in deep supercooling xylem parenchyma cells in Cercidiphyllum japonicum. Planta. 2012;235:747–759.10.1007/s00425-011-1536-3
- Kuwabara C, Wang D, Endoh K, et al. Analysis of supercooling activity of tannin-related polyphenols. Cryobiology. 2013;67:40–49.10.1016/j.cryobiol.2013.04.008
- Kuwabara C, Kasuga J, Wang D, et al. Change of supercooling capability in solutions containing different kinds of ice nucleators by flavonol glycosides from deep supercooling xylem parenchyma cells in trees. Cryobiology. 2011;63:157–163.10.1016/j.cryobiol.2011.06.001
- Rice-Evans CA, Miller NJ, Paganga G. Structures-antioxidant activity relationships of flavonoids and phenolic acids. Free Radical Biol Med. 1996;20:933–956.10.1016/0891-5849(95)02227-9
- Cushnie TPT, Lamb AJ. Antimicrobial activity of flavonoids. Int J Antimicrob Agents. 2005;26:343–356.10.1016/j.ijantimicag.2005.09.002
- Friedman M. Overview of antibacterial, antitoxin, antiviral, and antifungal activities of tea flavonoids and teas. Mol Nutr Food Res. 2007;51:116–134.10.1002/(ISSN)1613-4133
- Lindow SE, Arny DC, Upper CD. Erwinia herbicola: a bacterial ice nucleus active in increasing frost injury to corn. Phytopathology. 1978;68:523–527.10.1094/Phyto-68-523
- Lindow SE, Arny DC, Upper CD. Bacterial ice nucleation: a factor in frost injury to plants. Plant Physiol. 1982;70:1084–1089.10.1104/pp.70.4.1084
- Vali G. Quantitative evaluation of experimental results on the heterogeneous freezing nucleation of supercooled liquids. J Atmos Sci. 1971;28:402–409.10.1175/1520-0469(1971)028<0402:QEOERA>2.0.CO;2
- Arora R, Wisniewski ME, Scorza R. Cold acclimation in genetically related (sibling) deciduous and evergreen peach (Prunus persica [L.] Batsch). Plant Physiol. 1992;99:1562–1568.10.1104/pp.99.4.1562
- Caple G, Layton RG, McCurdy SN. Biogenic effects in heterogeneous ice nucleation. CryoLetters. 1983;4:59–64.
- Kasuga J, Mizuno K, Arakawa K, et al. Anti-ice nucleation activity in xylem extracts from trees that contain deep supercooling xylem parenchyma cells. Cryobiology. 2007;55:305–314.10.1016/j.cryobiol.2007.08.010
- Fuller MP, Hamed F, Wisniewski M, et al. Protection of plants from frost using hydrophobic particle film and acrylic polymer. Ann Appl Biol. 2003;143:93–98.10.1111/aab.2003.143.issue-1
- Francko DA, Wilson KG, Li QQ, et al. A topical spray to enhance plant resistance to cold injury and mortality. HortTechnology. 2011;21:109–118.
- Inoe Y, inventor; Daiichi Seimo Kk, assignee. Frost injury-preventing agents containing chitosan. Japan patent JP07-179843. 1995 Jul 18.
- Yamada Y, Yamada T, inventor; Royal Industries Kk, assignee. Method for preventing and/or decreasing frost damage of fruit tree. Japan patent JP2009-167192. 2009 Jul 30.
- Fiolini C, David B, Fourasté I, et al. Acylated kaempferol glycosides from Laurus nobilis leaves. Phytochemistry. 1998;47:821–824.
- Shibutani S, Samejima M, Doi S. Effects of stilbenes from bark of Picea glehnii (Sieb. et Zucc) and their related compounds against feeding behaviour of Reticulitermes speratus (Kolbe). J Wood Sci. 2004;50:439–444.10.1007/s10086-003-0583-1
- Matsuta T, Yamazaki M, Yajima A, et al. Flavonoids in Sasa senanensis and their antioxidative activities. Food Preserv Sci. 2009;35:11–16.