Abstract
In this study, we investigated the physiological function of glycerophosphodiesterase 5 (GDE5) in the proliferation of NIH3T3 fibroblasts. We used transcription activator-like effector nuclease (TALEN) in NIH3T3 cells with an intron targeting-mediated GDE5 gene knockout. The heterozygously GDE5-targeted NIH3T3 fibroblasts were isolated and showed decreased cell proliferation and up-regulation of EGFR mRNA expression, indicating that GDE5 modulates fibroblastic cell proliferation.
Key words:
Several studies show that abnormal choline metabolism is a critical trait of cancer cell proliferation. During cell division, there is an increased supply of total choline metabolites, including phosphocholine, because phosphatidylcholine (PC) must be newly synthesized, as the most abundant cellular phospholipid, in response to demand for increased plasma membrane synthesis.Citation1,2) Depending on cell types, net synthesis of PC is regulated by CDP-choline (Kennedy) pathway, which is dependent on intracellular choline.Citation3,4) In Saccharomyces cerevisiae, glycerophosphocholine (GPC) catabolized from PC is possibly reused for PC synthesis as an important source of choline.Citation5) However, the molecular mechanism underlying GPC hydrolysis in mammalian cells remains unclear. We previously isolated GDE5 and showed that it is localized in the cytoplasm and selectively hydrolyzes GPC in an in vitro reaction, suggesting that GDE5 could play a significant role as an intracellular source of choline.Citation6,7) However, it is considered that biallelic deletion of the GDE5 gene was lethal since GDE5 is essential for cellular choline metabolism. Hence, we tried to generate heterozygously GDE5-targeted cells using a combination of intron-targeting mediated by TALEN and homologous recombination. In the current study, we further examined the effect of heterozygous GDE5-targeting on the proliferation capacity of mouse NIH3T3 fibroblasts.
To isolate heterozygous GDE5-knockout NIH3T3 cells, we used Platinum TALEN technology, which was reported as highly active variant of TALEN.Citation8) Platinum TALEN was designed to cleave near exon 8, encoding the catalytic region of GDE5 (Fig. (A). Platinum TALEN plasmids were constructed as described previously with some modifications.Citation8) Briefly, each DNA-binding module was assembled into the modified ptCMV-136/63-VR vector that has EF1α promoter instead of CMV promoter using the two-step Golden Gate cloning method. The target sequence was 5′-TGCAGTGTGGATATTCCTtgattttcgttatttAAGATACTTTTGTTTTTA-3′, where uppercase and lowercase letters indicate TALEN target sequence and spacer sequence, respectively. The construct of the targeting vector was a derivative of DT-A/loxP/PGK-Neo-pA/loxP (RIKEN), containing puromycin-resistant gene sequences, sequentially connected in this order and flanked with short homologous sequences on both the 5’ and 3’ sides of the TALEN target site. The DT-A cassette was removed from the plasmid backbone. Mouse NIH3T3 cells were cultured in Dulbecco’s Modified Eagle Medium (Invitrogen) supplemented with 10% fetal bovine serum (Invitrogen), 100 U/mL penicillin, and 100 μg/mL streptomycin at 37° C in 5% CO2. When gene targeting is successful, the targeted NIH3T3 cells become resistant to puromycin. We performed transfection of the targeting vector together with the TALEN-expressing vector using GeneJuice (Merck Millipore). After puromycin-resistant colonies grew and became apparent in 1 week, clones were isolated by limiting dilution analysis in the presence of 0.2 μg/mL puromycin. We isolated 50 clones and further conducted PCR with primers: WT-F, 5′-GGATGAGACCCTAGGTTCAG-3′ and WT-R, 5′-GATATCTCCAGGCTGTTGGAC-3′; GE-F, 5′-GGATGAGACCCTAGGTTCAG-3′ and GE-R, 5′-CGAGACTAGTGAGACGTGCTAC-3′. As shown in Fig. (B), we identified two clones (clone 4 and 6), each of which showed heterozygously incorporation of the drug resistance gene at the expected gene locus. Next, as shown in Fig. (A), semi-quantitative PCR analysis was performed to evaluate expression of GDE5 mRNA between total RNAs from the two individual clones. The primers used for RT-PCR analysis were as follows: GDE5F, 5′-GACGATGGACAGTTTGGCATC-3′ and GDE5R, 5′-CACCCTGAACTACATGCTCAC-3′. The GDE5 transcript without the exon 8 from the GDE5-targeting allele was observed in clone 4 and 6. Next, quantitative PCR analysis was performed by the SYBR Green method using THUNDERBIRD SYBR qPCR Mix (TOYOBO, Osaka, Japan) and StepOnePlusTM (Applied Biosystems, Foster City, CA) with primers, GDE5F and WT-R. GDE5 mRNA level was significantly down-regulated in these two clones as compared with wild-type NIH3T3 cells (Fig. (A). We then performed indel analysis of the TALEN target sequence in the wild-type allele GDE5 gene in clone 4 and 6 by cloning followed by nucleotide sequencing of genomic PCR amplicons. In this experiment, two types of the nucleotide sequence from the wild-type allele of both clone 4 and 6 were amplified, suggesting a possibility that chromosome 2 which GDE5 gene is located on shows trisome.Citation9) As we expected, the nucleotide sequences around the target sites in the wild-type allele were found to be mutated (Fig. (B)). In order to find out whether GDE5+/− exhibited altered cellular proliferation, cell number was monitored by MTT and cell counting assays in the two heterozygously knockout clones versus the wild-type NIH3T3 fibroblastic cells. As shown in Fig. , these results clearly demonstrate that disruption of the GDE5 gene suppresses cellular proliferation significantly in the two clonally selected GDE5+/− cell lines as compared to wild-type NIH3T3 cells. We next focused on expression of growth factors and their receptors in the two GDE5+/− cell lines, because overexpression of growth factors and/or the receptors is associated with enhanced proliferation in cultured cells. In this study, we unexpectedly observed significant up-regulation of EGFR in the two GDE5+/− cell lines as compared to wild-type NIH3T3 cells (Fig. ). Aberrant overexpression of EGFR is studied in most types of epithelial cancers and EGFR overexpression is associated with large tumor size, metastasis, and poor clinical outcomes in breast cancers.Citation10,11) On the other hand, an earlier report showed that treatment with erlotinib, a small molecule inhibitor of EGFR, markedly increased EGFR mRNA in cancer cell lines, suggesting a compensatory feedback loop through up-regulation of EGFR expression.Citation12) EGFR gene promoter region lacks the classical TATA or CAAT boxes; instead p53 and early growth response-1 (Egr-1) enhance EGFR promoter activity by binding to the EGFR promoter region.Citation13–16) The tumor suppressor p53 and primary response gene Egr-1 have regulatory roles in signal transduction pathways mediating cellular proliferation and growth arrest as well as regulating apoptosis. These observations suggest a mechanistic link between the up-regulation of EGFR expression and arrest of cell proliferation. By several previous studiesCitation17–19), RNAi knockdown experiments showed relationships between enzymes in the CDP-choline pathway for PC synthesis and cellular proliferation. However, in this study, intracellular choline level was not significantly different between two GDE5+/− cell lines and wild-type NIH3T3 cells. Further analysis on levels of choline metabolites in the two GDE5+/− cell lines and wild-type NIH3T3 cells is needed to show functional interaction between choline metabolism and decreased cell proliferation.
Fig. 1. Strategy for TALEN-mediated GDE5 gene disruption.
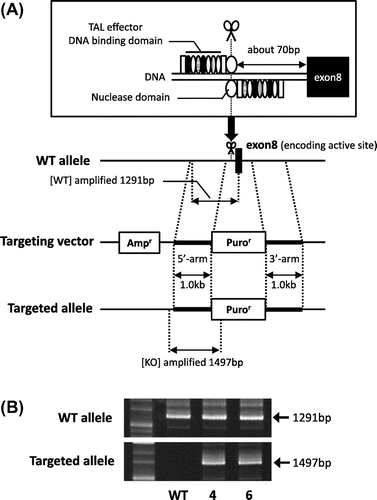
Fig. 2. Homologous recombination caused exon 8 skipping of the GDE5 gene.
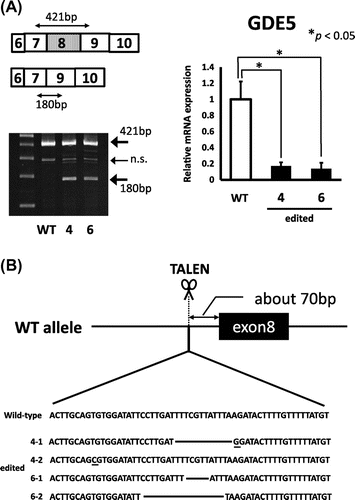
Fig. 3. Heterozygous GDE5 knockout suppressed fibroblastic cell proliferation.
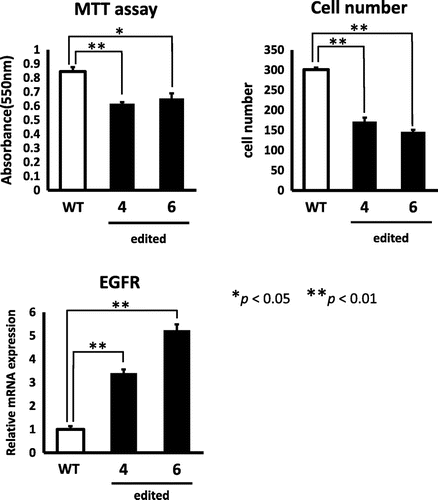
In this study, we investigated the biological role of GDE5 in the proliferation of NIH3T3 fibroblasts by successfully isolating the heterozygously GDE5-targeted NIH3T3 fibroblasts using TALEN. GDE5+/− fibroblasts showed decreased cell proliferation and up-regulation of EGFR mRNA expression. Increased EGFR expression in the two clonally selected GDE5+/− cell lines suggests not only a compensatory effect against suppression of cell proliferation, but also a biological relationship between choline metabolism and the cell sensitivity to EGF.
Author contributions
M. Nakamura conducted most of the experiments. M. Nakamura and T. Kumrungsee analyzed the results. T. Sakuma and T. Yamamoto conceived the idea for the experiments using TALEN technology. M. Nakamura and N. Yanaka designed the research, and wrote the manuscript. All the authors reviewed the results and approved the final version of the manuscript.
Disclosure statement
No potential conflict of interest was reported by the authors.
Notes
Abbreviations: GDE5, glycerophosphodiesterase 5; GPC, glycerophosphocholine; PC, phosphatidylcholine; KO, knockout; TALEN, transcription activator-like effector nuclease; WT, wild-type.
References
- Bergeron JJ, Warmsley AM, Pasternak CA. Phospholipid synthesis and degradation during the life-cycle of P815Y mast cells synchronized with excess of thymidine. Biochem. J. 1970;119:489–492.10.1042/bj1190489
- Graham JM, Sumner MC, Curtis DH, et al. Sequence of events in plasma membrane assembly during the cell cycle. Nature. 1973;246:291–295.10.1038/246291a0
- Northwood IC, Tong AH, Crawford B, et al. Shuttling of CTP: phosphocholine cytidylyltransferase between the nucleus and endoplasmic reticulum accompanies the wave of phosphatidylcholine synthesis during the G(0) –> G(1) transition. J. Biol. Chem. 1999;274:26240–26248.10.1074/jbc.274.37.26240
- Golfman LS, Bakovic M, Vance DE. Transcription of the CTP:phosphocholine cytidylyltransferase alpha gene is enhanced during the S phase of the cell cycle. J. Biol. Chem. 2001;276:43688–43692.10.1074/jbc.M108170200
- Fernández-Murray JP, McMaster CR. Glycerophosphocholine catabolism as a new route for choline formation for phosphatidylcholine synthesis by the Kennedy pathway. J. Biol. Chem. 2005;280:38290–38296.10.1074/jbc.M507700200
- Corda D, Mosca MG, Ohshima N, et al. The emerging physiological roles of the glycerophosphodiesterase family. FEBS J. 2014;281:998–1016.10.1111/febs.12699
- Okazaki Y, Ohshima N, Yoshizawa I, et al. A novel glycerophosphodiester phosphodiesterase, GDE5, controls skeletal muscle development via a non-enzymatic mechanism. J. Biol. Chem. 2010;285:27652–27663.10.1074/jbc.M110.106708
- Sakuma T, Ochiai H, Kaneko T, et al. Repeating pattern of non-RVD variations in DNA-binding modules enhances TALEN activity. Sci. Rep. 2013;3:143.10.1038/srep03379
- Leibiger C, Kosyakova N, Mkrtchyan H, et al. First molecular cytogenetic high resolution characterization of the NIH 3T3 cell line by murine multicolor banding. J. Histochem. Cytochem. 2013;61:306–312.10.1369/0022155413476868
- Freeman MR, Washecka R, Chung LW. Aberrant expression of epidermal growth factor receptor and HER-2 (erbB-2) messenger RNAs in human renal cancers. Cancer Res. 1989;49:6221–6225.
- Klijn JG, Berns PM, Schmitz PI, et al. The clinical significance of epidermal growth factor receptor (EGF-R) in human breast cancer: a review on 5232 patients. Endocr. Rev. 1992;13:3–17.
- Jimeno A, Rubio-Viqueira B, Amador ML, et al. Epidermal growth factor receptor dynamics influences response to epidermal growth factor receptor targeted agents. Cancer Res. 2005;65:3003–3010.10.1158/0008-5472.CAN-04-3586
- Deb SP, Muñoz RM, Brown DR, et al. Wild-type human p53 activates the human epidermal growth factor receptor promoter. Oncogene. 1994;9:1341–1349.
- Bheda A, Creek KE, Pirisi L. Loss of p53 induces epidermal growth factor receptor promoter activity in normal human keratinocytes. Oncogene. 2008;27:4315–4323.10.1038/onc.2008.65
- Nishi H, Nishi KH, Johnson AC. Early Growth Response-1 gene mediates up-regulation of epidermal growth factor receptor expression during hypoxia. Cancer Res. 2002;62:827–834.
- Sauer L, Gitenay D, Vo C, et al. Mutant p53 initiates a feedback loop that involves Egr-1/EGF receptor/ERK in prostate cancer cells. Oncogene. 2010;29:2628–2637.10.1038/onc.2010.24
- Glunde K, Raman V, Mori N, et al. RNA interference–mediated choline kinase suppression in breast cancer cells induces differentiation and reduces proliferation. Cancer Res. 2005;65:11034–11043.10.1158/0008-5472.CAN-05-1807
- Miyake T, Parsons SJ. Functional interactions between Choline kinase α, epidermal growth factor receptor and c-Src in breast cancer cell proliferation. Oncogene. 2012;31:1431–1441.10.1038/onc.2011.332
- Arsenault DJ, Yoo BH, Rosen kV, et al. Ras -induced up-regulation of CTP: phosphocholine cytidylyltransferase α contributes to malignant transformation of intestinal epithelial cells. J. Biol. Chem. 2013;288:633–643.10.1074/jbc.M112.347682