Abstract
Most plants do not tolerate highly saline environments; the development of salt stress tolerance is crucial for improving crop yield. An efficient way of finding genes involved in salt tolerance is to study and use data from halophytes. In this study, we used the Mesembryanthemum crystallinum (ice plant) expression data-set and selected for further study the gene McHKT2, which encodes for the Arabidopsis sodium transporter ortholog AtHKT1. In comparison with the HKT1 amino acid sequences from other plants, McHKT2 has several unique features. It seems to be localized to the plasma membrane, and its overexpression confers strong salt tolerance in Arabidopsis thaliana. Our results indicate that McHKT2 is a suitable candidate protein that can induce salt tolerance in non-halophytes. Like McHKT2, using transcriptome data-sets from halophytes such as ice plant give us an efficiency way to obtain new gene resources that might involve in plant salt tolerance.
Graphical abstract
From the ice plant expression data, we selected a gene, McHKT2, which is an ortholog to the Arabidopsis HKT1. McHKT2-OX in Arabidopsis showed salt tolerance.
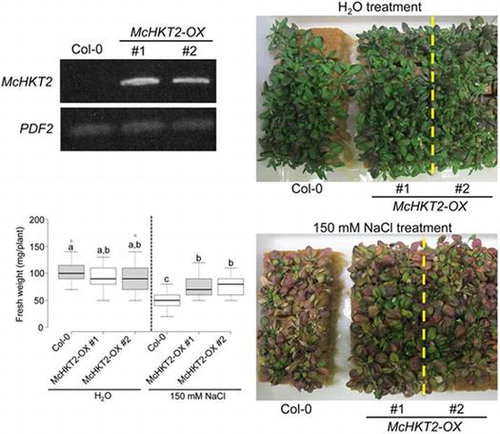
Plants cannot move away from the place where they germinated and must therefore adapt or respond to the environmental changes around them. Therefore, the regulation of gene expression—the induction or repression of certain genes—by means of which living organisms contend with unfavorable conditions, is particularly vital in plants. Salt stress, one of the abiotic environmental factors with which plants must content, is detrimental to agriculture. Sodium chloride (NaCl) inhibits water uptake by increasing osmotic stress and inhibiting enzyme activity. Consequently, high NaCl levels arrest growth and ultimately kill plants. However, sodium ion (Na+) transport is essential for regulating plant growth under high-salt conditions. For this reason, plants have several Na+ transporters.Citation1,2) It has been reported that High-Affinity K+ Transporter1 (HKT1) family proteins play important roles in plant Na+ resistance. HKT1 retrieves Na+ from the xylem stream and reduces its accumulation in the shoot.Citation3) In glycophytes, Na+ hyperaccumulation in the shoot results in firing. The glycophyte Arabidopsis thaliana has one copy of the HKT1 gene. The Arabidopsis hkt1 mutant exhibited abnormal Na+ accumulation in the shoot.Citation4,5) Type-specific overexpression of AtHKT1 in the root cells reduced Na+ accumulation in the shoot and induced salinity tolerance.Citation6) These studies indicate that Na+ transport by HKT1-type transporters is a useful tool for engineering salt tolerance in glycophytes. On the other hand, in the ice plant, a halophyte, Na+ accumulates in the leaf epidermal bladder cells. The sequestration of Na+ in specific parts of the leaves is an important component of salinity tolerance in ice plants.Citation7)
Since the athkt1 mutant accumulates Na+ in the shoot, we hypothesized that AtHKT1 ortholog expression in the ice plant might be downregulated by salt stress. We searched for an AtHKT1 ortholog in the ice plant using its root expression database previously established with RNAseq.Citation8) We found one AtHKT1 ortholog candidate, McHKT2, an ice plant HKT1 type protein that is rarely expressed in the shoot.Citation9) Comparison of the McHKT2 amino acid sequence with HKT1 from several other plant species revealed that McHKT2 has a unique amino acid sequence consisting of 13 contiguous threonine residues between the first- and second M1-P-M2 regions. We found that the salinity tolerance in Arabidopsis overexpressing McHKT2 was significantly higher than that of the control plant. Our results provide evidence for the efficacy of engineering salinity tolerance in plants by using the halophyte-type HKT1 gene.
Materials and methods
Plant materials and growth conditions
A. thaliana Columbia-0 (Col-0) was used as the wild-type. Seeds of M. crystallinum and A. thaliana were sterilized with 1% v/v bleach and 0.05% v/v Triton X-100 for 5 min then washed three times with sterilized water. Seeds were germinated over a three-day period in the dark at 4 °C on Murashige and Skoog (MS) medium (Wako Pure Chemical Industries, Ltd., Osaka, Japan) supplemented with 1% w/v sucrose and 1% w/v agarose. Plants were grown vertically in a growth chamber (Panasonic Healthcare Co. Ltd., Tokyo, Japan) at 22 °C under a 16 h light/8 h dark photoperiod.
For the salt tolerance test, seeds were placed directly onto rockwool immersed in water. After 10 d, the plants were treated with 40 mM NaCl every other day for 7 days. Then the plants were treated with 150 mM NaCl every other day for two weeks. Photographs were taken and whole plant fresh weights were determined at the end of the salt treatment.
Cloning of McHKT2 gene and generating Arabidopsis transgenic lines
Total RNA was isolated from seven-day-old ice plant roots using the RNeasy Plant mini kit (Qiagen, Hilden, Germany). The RNA was reverse-transcribed using ReverTra Ace qPCR RT Master Mix with gDNA Remover (TOYOBO, Osaka, Japan). This cDNA pool was used as the template and the McHKT2 coding sequences were amplified with the primer set indicated below. To clone the McHKT2 coding sequence, CACC sequences were attached to the 5′ end of the forward primer so that the amplified DNA cloned into pENTR-D-Topo vector (Thermo Fisher Scientific, Waltham, MA, USA). The following primer set was used: McHKT2_Fw, 5′-CACCATGGGGAGATTTGGGTTTCTT-3′ and McHKT2_Rv, 5′-TAGGAGCTTCCATGCTCTGCCTCCT-3′.
For the 35S::McHKT2 construct, plasmids were assembled using Gateway cloning technology (Thermo Fisher Scientific, Waltham, MA, USA). McHKT2 containing pENTR-D-TOPO was cloned into pGWB551Citation10) using LR Clonase II (Thermo Fisher Scientific, Waltham. MA, USA). The resulting plasmids were transferred into Agrobacterium C58C1 (pMP90) and used to transform Col-0 by the floral dip method.Citation11) The 35S::McHKT2 transformants were grown on MS medium containing 30 μg/mL hygromycin. Homozygous transgenic T3 generation lines were used in further experiments.
Transient expression assay in Arabidopsis protoplasts
Arabidopsis root protoplasts were prepared as previously described.Citation12) A protoplast suspension (100 μL:105 protoplasts/mL) was cotransfected with 10 μg McHKT2 in pGWB551. The transfected protoplasts were incubated at 22 °C for 20 h in the dark. Samples were viewed under a Zeiss LSM700 confocal microscope fitted with a 20 X objective lens (Carl Zeiss, Oberkochen, Germany) using 488 nm laser for GFP excitation.
Semi-quantitative RT-PCR
RNA was isolated from 35S::McHKT2 in seven-day-old whole imbibed Col-0 seedlings using an RNeasy plant kit (Qiagen, Hilden, Germany). First-strand cDNA was synthesized with ReverTra Ace qPCR RT Master Mix and gDNA Remover (TOYOBO, Osaka, Japan). Semi-Quantitative RT-PCR was performed with the forward (Fw) and reverse (Rv) primer pairs used to clone McHKT2, and PDF2_Rv, 5′-GTTCTCCACAACCGCTTGGT-3′. PDF2 was used as the internal control gene.Citation13)
Results
An AtHKT1 ortholog in ice plant is downregulated by NaCl treatment
To find orthologs of AtHKT1 in the ice plant, we searched the root expression database (http://dandelion.liveholonics.com/pothos/Mcr/)Citation8) using the AtHKT1 protein sequence (At4g10310.1) as a query for a BLAST strategy. In the database, we performed a tblastn search and found only one AtHKT1 ortholog candidate with a significantly low e-value (< e-3; e-value = 5.54567e-134). Mcr004515.000, the sequence ID in the database, predicted a 1632 bp ORF and a protein 543 residues long. We checked Mcr004515.000 expression levels in the database. Mcr004515.000 was repressed after the 140 mM NaCl treatment and also at higher NaCl concentrations (Fig. ).
Fig. 1. Mcr004515.000 expression in ice plant roots was strongly reduced upon NaCl treatment.
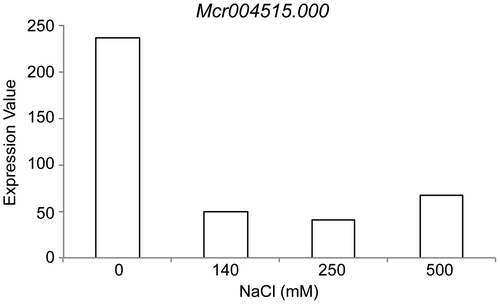
McHKT2 has unique threonine stretch in the protein sequence
To compare HKT1 amino acid sequences between halophytes and glycophytes, we conducted a BLAST search to find Mcr004515.000 orthologs in the National Center for Biotechnology Information (NCBI) database. First, we found that the Mcr004515.000 we isolated in this study was identical to the McHKT2 registered by Quigley et al. (GenBank ID: AAO73474.1). Therefore, we renamed Mcr004515.000 to McHKT2. To compare HKT amino acid sequences among plant species, we aligned McHKT2 with A. thaliana (AtHKT1), Oryza sativa (OsHKT1;1), Thellungiella salsuginea (TsHKT1;1), and Mesembryanthemum crystallinum (McHKT1) (Fig. ). McHKT2 shares 46.7, 46.7, 47.0, and 43.0% identity with McHKT1, AtHKT1, TsHKT1, and OsHKT1, respectively (Fig. ). According to the alignment, McHKT2 conserved four transmembrane protein pore domains (M1-P-M2). Moreover, McHKT2 conserved a serine residue in the first P region required for ion specificity in all HKT1.Citation14–16) According to the bioinformatics and structural analyses, this serine residue seems to be important for Na+ selectivity as the “selectivity filter”.Citation17) This result indicated that McHKT2 may be a highly selective Na+ transporter. McHKT2 has a unique amino acid sequence containing 13 contiguous threonine residues between the first and second M1-P-M2 regions (Fig. ).
Fig. 2. Amino acid sequence of McHKT2 and sequence comparison of HKT1 homologs from A. thaliana (AtHKT1), O. sativa (OsHKT1;1), T. salsuginea (TsHKT1;1), and M. crystallinum (McHKT1).
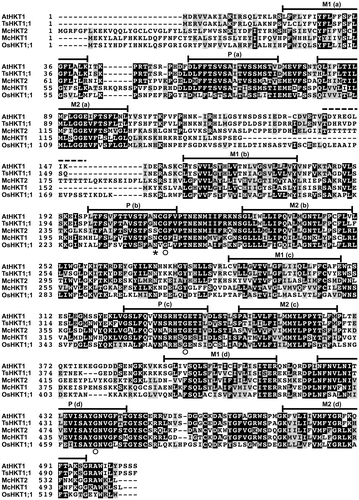
It has been reported that amino acid alterations in the P(b) region of AtHKT1 in the halophyte T. salsuginea contributes to salt tolerance in A. thaliana.Citation18) We checked this position in McHKT2 (Fig. ) and found that it has a serine in the place of aspartic acid.
Overexpression of McHKT2 in Arabidopsis increased salt tolerance
Since McHKT2 may contribute to salt tolerance in glycophytes, we cloned McHKT2 and overexpressed it in Arabidopsis. Using the cDNA pool synthesized from ice plant roots, we cloned the McHKT2 cds region via polymerase chain reaction (PCR). We then determined the cellular localization of McHKT2 in Arabidopsis protoplasts using transient expression. We found that McHKT2 appeared to be located on the plasma membrane (Fig. (A)). This finding was consistent with those for AtHKT1 and McHKT1, which were determined to be localized in the plasma membranes of Arabidopsis and ice plants, respectively.Citation3,9) Moreover, the McHKT2 cloned in this study contained functional membrane-localizing domains predicted from the amino acid sequences.
Fig. 3 Cellular localization of McHKT2 and effect of overexpression of McHKT2 in Arabidopsis.
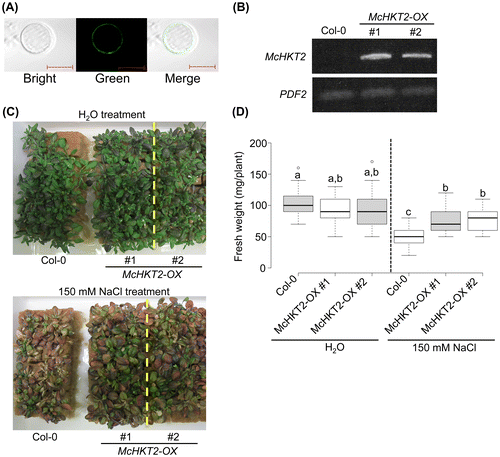
We overexpressed McHKT2 in the A. thaliana Col-0 background using a 35S promoter to verify whether McHKT2 overexpression affects Arabidopsis salt tolerance. Using RT-PCR with a McHKT2-specific primer set, we detected McHKT2 transcripts in Arabidopsis transgenic lines (McHKT2-OX) but not in the Col-0 wild-type (Fig. (B)). It has been reported that the simple overexpression of AtHKT1 with the 35S promoter increased salt sensitivity in Arabidopsis.Citation6) However, adult McHKT2-OX Arabidopsis plants showed significant salt tolerance (Fig. (C)). The fresh weights of McHKT2-OX were significantly higher than those of the wild-type following 150 mM NaCl treatment for 14 d (Fig. (D)). All these data indicate that the overexpression of McHKT2 conferred some degree of salt tolerance in Arabidopsis.
Discussion
We hypothesized that we could use halophyte proteins to engineer plant salt tolerance. We focused on the AtHKT1 ortholog in M. crystallinum (ice plant), a halophyte. Using its RNAseq database, we cloned a functional cDNA related to salt tolerance. AtHKT1 is a Na+ transporter. It retrieves the ion from the root xylem and prevents it from accumulating in the shoot.Citation3) These facts indicated that if we manipulate HKT1 function, we can introduce salt tolerance to glycophytes. Several studies have revealed the importance of HKT1 in certain plants.Citation19)
We found only one AtHKT1 ortholog in our database which, according to the BLAST search, has a significantly low e-value. Nevertheless, another HKT1 protein has been reported in ice plant.Citation9) Su et al. cloned McHKT1 from ice plant leaves and shows that McHKT1 is only slightly expressed in the roots. These findings account for the fact that we could not find McHKT1 in our database search: we compiled the ice plant expression database using root RNA alone. This observation strongly indicates that McHKT2 plays a major role in root tissues, and McHKT1 and McHKT2 function in a tissue-specific manner.
Decreasing HKT expression in the ice plant does not affect salt tolerance. This expression pattern resembles that of AtHKT1, which decreased after 75 mM NaCl treatment.Citation3) Moreover, The Arabidopsis hkt1 mutant accumulates high Na+ in the shoot and consequently suffers critical damage.Citation4,5) Mcr004515.000 transcription regulation would be required in the ice plant as well. Since the ice plant can sequester Na+ in its leaf epidermal bladder cells, low HKT levels will not significantly increase its salt sensitivity. It has been reported that McHKT1 localizes in leaf cell plasma membranes.Citation9) McHKT2 also appeared to be situated in the plasma membranes of Arabidopsis root protoplasts. Therefore, like McHKT1, McHKT2 may participate in cellular Na+ uptake.
It was reported that the constitutive overexpression of AtHKT1 driven by a 35S promoter increased salt sensitivity in Arabidopsis to a level similar to that found in the hkt1 mutant.Citation6) Nevertheless, cell-specific overexpression of AtHKT1, especially in the root stele, induced strong salt tolerance.Citation6) Møller et al. indicated that root-specific expression of HKT1 is necessary to regulate the distribution of ions like Na+ and K+ between shoot and root. Our results showed that McHKT2 overexpression driven by the 35S promoter induced significant salt tolerance in Arabidopsis. This finding may indicate functional differences between AtHKT1 and McHKT2 and may be explained by the variations in amino acid sequences between McHKT1 and AtHKT1. A candidate region that might significantly contribute to this difference is the threonine repeat located between the first and second MPM regions in McHKT2. There is no information about the molecular function of this long contiguous Thr residue sequence in plants. It may alter ion affinity or transport direction. To elucidate its function, we must delete it from McHKT2 or add it to AtHKT1 and determine whether it is involved in salt tolerance. Another distinct difference between McHKT2 and AtHKT1 is that the former has a serine instead of an asparagine in the P(b) region (Asn 211 in AtHKT1, and Ser 254 in McHKT2) (Fig. ). Apparently, the Asn 211 in AtHKT is important for ion selectivity, and substituting it with Asp confers salt tolerance in Arabidopsis.Citation18) Moreover, like McHKT2, the HKT1-type protein SbHKT1;4 in Sorghum bicolor possesses a Ser residue at the position corresponding to Asn 211 in AtHKT1.Citation20) SbHKT1;4 transports both Na+ and K+ under Na+ stress and plays a key role in sorghum salt tolerance by maintaining a proper Na/K ratio.Citation20) Several halophytes like Eucalyptus camaldulensis, Salicornia bigelovii, Suaeda salsa, and Medicago truncatula have HKT1 with Ser residues at the same position as that of McHKT2. These results indicate that the Ser residue at this position might serve an important role in ion selectivity of the HKT-type transporter. In the future, we must determine whether changing the Asn in AtHKT1 to Ser would induce plant salt tolerance.
In order to engineer salt tolerance in glycophytes, it may be necessary to determine which amino acids and their positions are important for improving HKT. The regulation of HKT expression, including cell type specificity and timing of the expression, may prove to be an efficient way to engineer salt tolerance.
Author contribution
H.T. designed experiments; T.N., M.F., S.S., and H.T. performed experiments; T.N., M.F., A.M., and H.T. analyzed experiments; H.T. wrote the paper. All the authors have read and approved the final manuscript.
Disclosure statement
No potential conflict of interest was reported by the authors.
Acknowledgements
We thank K. Nishikawa for his assistance with the cloning of McHKT2, T. Itaya for his assistance with the transient expression assay, and Dr. Y. Tada for his helpful suggestions.
References
- Greenway H, Munns RA. Mechanisms of salt tolerance in nonhalophytes. Ann Rev Plant Physiol. 1980;31:149–190.10.1146/annurev.pp.31.060180.001053
- Volkov V. Salinity tolerance in plants. Quantitative approach to ion transport starting from halophytes and stepping to genetic and protein engineering for manipulating ion fluxes. Front Plant Sci. 2015;6:873.
- Sunarpi, Horie T, Motoda, J, et al. Enhanced salt tolerance mediated by AtHKT1 transporter-induced Na unloading from xylem vessels to xylem parenchyma cells. Plant J. 2005;44:928–938.10.1111/tpj.2005.44.issue-6
- Mäser P, Eckelman B, Vaidyanathan R, et al. Altered shoot/root Na+ distribution and bifurcating salt sensitivity in Arabidopsis by genetic disruption of the Na+ transporter AtHKT1. FEBS lett. 2002;531:157–161.10.1016/S0014-5793(02)03488-9
- Berthomieu P, Conéjéro G, Nublat A, et al. Functional analysis of AtHKT1 in Arabidopsis shows that Na(+) recirculation by the phloem is crucial for salt tolerance. EMBO J. 2003;22:2004–2014.10.1093/emboj/cdg207
- Møller IS, Gilliham M, Jha D, et al. Shoot Na+ exclusion and increased salinity tolerance engineered by cell type-specific alteration of Na+ transport in Arabidopsis. Plant Cell. 2009;21:2163–2178.10.1105/tpc.108.064568
- Agarie S, Shimoda T, Shimizu Y, et al. Salt tolerance, salt accumulation, and ionic homeostasis in an epidermal bladder-cell-less mutant of the common ice plant Mesembryanthemum crystallinum. J Exp Bot. 2007;58:1957–1967.10.1093/jxb/erm057
- Tsukagoshi H, Suzuki T, Nishikawa K, et al. RNA-seq analysis of the response of the halophyte, Mesembryanthemum crystallinum (ice plant) to high salinity. PLoS One. 2015;10:e0118339.10.1371/journal.pone.0118339
- Su H, Balderas E, Vera-Estrella R, et al. Expression of the cation transporter McHKT1 in a halophyte. Plant Mol Biol. 2003;52:967–980.10.1023/A:1025445612244
- Nakagawa T, Suzuki T, Murata S, et al. Improved gateway binary vectors: high-performance vectors for creation of fusion constructs in transgenic analysis of plants. Biosci Biotechnol Biochem. 2007;71:2095–2100.10.1271/bbb.70216
- Clough SJ, Bent AF. Floral dip: a simplified method for Agrobacterium-mediated transformation of Arabidopsis thaliana. Plant J. 1998;16:735–743.10.1046/j.1365-313x.1998.00343.x
- Yoo SD, Cho YH, Sheen J. Arabidopsis mesophyll protoplasts: a versatile cell system for transient gene expression analysis. Nat Protoc. 2007;2:1565–1572.10.1038/nprot.2007.199
- Czechowski T, Stitt M, Altmann T, et al. Genome-wide identification and testing of superior reference genes for transcript normalization in Arabidopsis. Plant Physiol. 2005;139:5–17.10.1104/pp.105.063743
- Horie T, Yoshida K, Nakayama H, et al. Two types of HKT transporters with different properties of Na+ and K+ transport in Oryza sativa. Plant J. 2001;27:129–138.10.1046/j.1365-313x.2001.01077.x
- Maser P, Hosoo Y, Goshima S, et al. Glycine residues in potassium channel-like selectivity filters determine potassium selectivity in four-loop-per-subunit HKT transporters from plants. Proc Natl Acad Sci USA. 2002;99:6428–6433.10.1073/pnas.082123799
- Tholema, N, Vor der Bruggen, M, Maser, P, et al. All four putative selectivity filter glycine residues in KtrB are essential for high affinity and selective K+ uptake by the KtrAB system from Vibrio alginolyticus. J Biol Chem. 2005;280:41146–41154.10.1074/jbc.M507647200
- Waters S, Gilliham M, Hrmova M. Plant high-affinity potassium (HKT) transporters involved in salinity tolerance: structural insights to probe differences in ion selectivity. Int J Mol Sci. 2013;14:7660–7680.10.3390/ijms14047660
- Ali A, Raddatz N, Aman R, et al. A single amino-acid substitution in the sodium transporter HKT1 associated with plant salt tolerance. Plant Physiol. 2016;171:2112–2126.10.1104/pp.16.00569
- Horie T, Hauser F, Schroeder JI. HKT transporter-mediated salinity resistance mechanisms in Arabidopsis and monocot crop plants. Trends Plant Sci. 2009;14:660–668.10.1016/j.tplants.2009.08.009
- Wang TT, Ren ZJ, Liu ZQ, et al. SbHKT1;4, a member of the high-affinity potassium transporter gene family from Sorghum bicolor, functions to maintain optimal Na+ /K+ balance under Na+ stress. J Integr Plant Biol. 2014;56:315–332.10.1111/jipb.v56.3