Abstract
Microbial transglutaminase (MTG) is an enzyme widely used in the food industry. Mutiple-site mutagenesis of Streptomyces mobaraensis transglutaminase was performed in Escherichia coli. According to enzymatic assay and thermostability study, among three penta-site MTG mutants (DM01-03), DM01 exhibited the highest enzymatic activity of 55.7 ± 1.4 U/mg and longest half-life at 50 °C (418.2 min) and 60 °C (24.8 min).
Transglutaminases (protein-glutamine γ-glutamyltransferase, EC 2.3.2.13) are a group of enzymes that catalyze the acyl transfer reaction between the ε-amino groups of lysine residues/primary amines (acyl acceptors) and the γ-carboxyamide groups of peptide-bound glutamine residues (acyl donors) [Citation1,2]. The formation of ε-(γ-glutamyl)lysine isopeptide bonds modified by transglutaminases inter- or intra-molecularly results in the cross-linking and polymerization of proteins [Citation3,4].
Transglutaminases are widely distributed in mammals, plants, and micro-organisms, and their functions and properties have been well studied [Citation5–7]. Among these enzymes, microbial transglutaminase (MTG) was first discovered in Streptomyces mobaraensis where it is expressed as a pre-pro-enzyme consisting of a signal peptide, a 45 amino acids (Aa) pro-region and a 331 Aa mature peptide and then secreted from the cytoplasmic compartment as an inactive pro-MTG, which is finally activated by proteolytic processing to the enzymatically active mature form [Citation8]. Owing to its rather broad substrate specificity, independence of Ca2+, and small molecular size it has been widely used as an industrial catalyst [Citation9,Citation10]. The major application of MTG is in the food industry to modify food proteins with the aim of enhancing their physicochemical properties such as viscosity, elasticity, emulsification, foaming, water-holding capacity [Citation11,12]. Various potential applications beyond food processing have also been studied in recent years such as materials science, textiles and leather processing, tissue engineering, other biotechnological fields [Citation13,14]. For some of the applications including thermoplastic processing by extrusion and the generation of thermostable microcapsules by immobilized enzymes, it would be advantageous to carry out the cross-linking reaction at higher temperatures and with increased activity [Citation15,16]. Therefore, MTG variants with higher activity and thermostability are expected to be of use not only in current processes, but also to extend the range of novel applications.
Previously, several site-directed MTG mutants with higher enzyme activity or thermostability were obtained by site-directed mutation [Citation15,16]. Here, by combining mutations (S2P; S199A; K269D) that conferred MTG with higher enzyme activity with the others (S23V-Y24N-K294L; S23V-Y24N-H289Y) that rendered MTG with better thermostability [Citation15–17], we report three penta-site MTG variants (DM01: S2P-S23V-Y24N-S199A-K294L; DM02: S2P-S23V-Y24N-K269D-K294L; DM03: S23V-Y24N-K269D-H289Y-K294L) (Table S1), which exhibited both higher enzyme activity and thermostability, were obtained from Escherichia coli.
Construction of recombinant vectors encoding MTGs was achieved by four-step round PCRs (See Supplemental materials). To investigate the expression of recombinant MTG in E. coli, vectors (pET-mtg and pET-mtg(DM01-03)) harboring genes of MTG and its variants were transformed into E. coli BL21 (DE3) individually. Based on the protocol from Yang et al., the activated MTG samples were purified from cytoplasm by immobilized metal affinity chromatography and analyzed by SDS-PAGE [Citation18]. As shown in Figure S1,a band with a molecular weight equivalent to the theoretical mass(38.85 kDa) of mature MTG-6His was detected indicating MTGs have been produced in E. coli and activated by trypsin successfully in spite of unexpected degradations were observed which had been observed by Yang et al. as well [Citation18].
In order to compare the enzymatic activity of recombinant MTGs to wide-type MTG, colorimetric hydroxamate procedure using N-benzyloxycarbonyl-L-glutaminylglycine (Z-Gln-Gly, Sigma-Aldrich Co., St. Louis, MO) as a substrate was carried out [Citation19], while protein concentrations were determined using a Bradford Protein Assay Kit (TaKaRa Shuzo). The specific activities of purified MTGs are shown in Table . Purified wild-type MTG exhibited a specific activity of 25.4 ± 0.5 U/mg, which was lower than these of all three mutants. Among these penta-site mutants, DM01 exhibited the highest specific activity of 55.7 ± 0.4 U/mg, corresponding to approximately 2.2 times that of wild-type MTG, while the activity of DM02, sharing four similar mutagenesis with DM01, was detected to be 53.4 ± 0.9 U/mg, slightly lower than DM01 but around 2.1 times that of wild-type MTG. DM03 was determined to gain the activity of 44.9 ± 0.4 U/mg. These results indicated that combining selected single mutations conferred MTG with higher activity. In our study two variants possessing S2P, DM01, and DM02, showed higher activity than DM03 which does not have S2P. It seems this mutation, S2P, has more beneficial effect on improving the activity of MTG than S199A and K269D although it was reported that only replacing Ser2 by Pro would improve the specific activity of MTG by 1.8 times [Citation15]. In Figure (A) and (B), it was shown that after completely folding, Ser2 located at the top of the entrance of active sites consisted by Cys64, Asp255, and His274 so we inferred that larger side chain of residue at position 2 would increase the polar interaction between MTG and substrate. Previously, the negative effect of one three-site mutation combination (S23V-Y24N-H289Y) on enzymatic activity of MTG was observed to be reversed by adding a K269S mutation [Citation15]. Our results confirmed this observation as in DM03 additional mutation K269D offset the activity reduction. Lys269 is located close to the β6-sheet that is part of active cleft. Replacement of positively charged residue, Lys, at this position by uncharged or negatively charged residue could increase the activity of MTG. This was probably because positive ion at position 269 hampered Z-Gln-Gly to interact with enzyme although different substrate should be tested to specify the function role of electric charge at position 269. Ser199 located at β3-strand which is closely near to the catalytic triad [Citation20]. Replacing Ser199 by Ala would increase the hydrophobicity of the catalytic triad area which might in turn increase the hydrophobic interaction between enzyme and substrate.
Table 1. Biochemical properties of wild-type MTG and its variants.
Figure 1. Schematic illustration of three penta-site MTG mutations. The mutated amino acids are colored in red and the catalytic triad is colored in blue. (A): DM01; (B): DM02; (C): DM03.
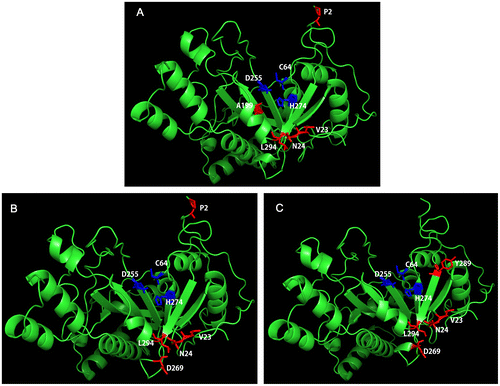
Considering that the application of MTG in food processing or others aspects may be involved in heating treatment, the thermostability of variant enzymes is an important feature. The optimal reaction temperature for MTG was studied. As shown in Figure (A), all recombinant MTGs exhibited optimal activity at 55 °C, while the optimal temperature of wild-type MTG is 50 °C, which is in agreement with the previous reports [Citation21]. This results indicated that all three mutanted MTG were less thermo-sensitive than wild-type MTG. To further explore the thermostability of MTGs, the half-life (t1/2) of enzymes at 60 and 50 °C were also studied. As shown in Table and Figure (B) and (C) wild-type MTG has t1/2(60 °C) of 2.5 min and t1/2(50 °C) of 40.1 min and all mutants were more thermostable than wild-type MTG. Among three of them, DM01 has the highest t1/2(60 °C) and t1/2(50 °C), which were 9.9 and 10.4 times higher than these of wild-type MTG. DM02 and DM03 exhibited slightly less improvement on thermostability as DM01 (DM02: t1/2(50 °C) of 357.1 min and t1/2(60 °C) of 14.4 min; DM03: t1/2(50 °C) of 377.2 min and t1/2(60 °C) of 16.1 min) (Table and Figure ). Using DNA-shuffling, Buettner et al. have reported mutant UH308-B(S23V-Y24N-K294L) gained highest residual activities after pre-incubation in 50 °C(t1/2: 409.0 min) and 60 °C(t1/2: 24.3 min) [Citation15]. After being added three pairs of carefully selected mutations to UH308-B, penta-site-mutated MTGs exhibited improved traits both in specific activity and in thermostability. According to Figure , after protein folding, S23V, Y24N and K294L gathered close to the left side wall of the active cleft and increased the hydrophobic interactions in this region resulting in higher stabilization of MTG structure at higher temperature [Citation15,20]. The mutations H289Y and K294L are located in the β7-strand of the MTG structure [Citation20]. Based on the study of Buettener et al., these two mutations increase the hydrophobic interactions in the active cleft and stabilize the structure of MTG, which explain why DM02 has the second highest thermostability among DM01-03 [Citation15]. Lys269 is located near to the area where S23V, Y24N and K294L gather (Figure (B) and C). Replacing positively charged Lysine by negatively charged aspartic acid might affect the ion distribution in this area which might explain why DM02 and DM03 had relatively lower thermostability than DM01. Our study demonstrated that by combining selected mutations that confer MTG high activity and high thermostability respectively, we successfully obtain engineered MTG variants possessing novel potentials in both activity and thermostability which would benefit food processing involving in higher temperature and offer a new inspiration for creating multiple-site mutagenesis with one-step transformation.
Author contributions
D.M. and Z.Z. conceived the study and designed the experiments. D.M., J.L., C.S., and H.L. performed the experiments. D.M. J.L., and H.L. analyzed data. D.M. wrote the manuscript. X.L., J.C., S.L., P.Y., and S.J. corrected the manuscript.
Disclosure statement
No potential conflict of interest was reported by the authors.
Funding
This work was supported by the National High Technology Research and Development Program (“863” Program) of China [grant number 2013AA102201]; the Major Project of Science and Technology of Anhui Province, China [grant number 1301031031]; the Fundamental Research Funds for the Central Universities, China [grant number JZ2016HGBZ0777] and Anhui Provincial Natural Science Foundation [grant number JZ2017AKZR0123].
Supplemental data
The supplementary material for this paper is available online at https://doi.org/10.1080/09168451.2017.1403881.
Supplemental_data-h.docx
Download MS Word (161 KB)References
- Ohtsuka T, Sawa A, Kawabata R, et al. Substrate specificities of microbial transglutaminase for primary amines. J Agric Food Chem. 2000;48(12):6230–6233.10.1021/jf000302k
- Yokoyama K, Nio N, Kikuchi Y. Properties and applications of microbial transglutaminase. Appl Microbiol Biotechnol. 2004;64(4):447–454.10.1007/s00253-003-1539-5
- Clare DA, Gharst G, Maleki SJ, et al. Effects of transglutaminase catalysis on the functional and immunoglobulin binding properties of peanut flour dispersions containing casein. J Agric Food Chem. 2008;56(22):10913–10921.10.1021/jf801641d
- Li C, Xiong YL, Chen J. Oxidation-induced unfolding facilitates myosin cross-linking in myofibrillar protein by microbial transglutaminase. J Agric Food Chem. 2012;60(32):8020–8027.10.1021/jf302150 h
- Aloisi I, Cai G, Serafini-Fracassini D, et al. Transglutaminase as polyamine mediator in plant growth and differentiation. Amino Acids. 2016;48(10):2467–2478.10.1007/s00726-016-2235-y
- Liu S, Zhang D, Wang M, et al. The order of expression is a key factor in the production of active transglutaminase in Escherichia coli by co-expression with its pro-peptide. Microb Cell Fact. 2011; 10:112. DOI:10.1186/1475-2859-10-112
- Dickneite G, Herwald H, Korte W, et al. Coagulation factor XIII: a multifunctional transglutaminase with clinical potential in a range of conditions. Thromb Haemost. 2015;113(4):686–697.10.1160/TH14-07-0625
- Pasternack R, Dorsch S, Otterbach JT, et al. Bacterial pro-transglutaminase from Streptoverticillium mobaraense – purification, characterisation and sequence of the zymogen. Eur J Biochem. 1998;257(3):570–576.10.1046/j.1432-1327.1998.2570570.x
- Chen K, Liu S, Wang G, et al. Enhancement of Streptomyces transglutaminase activity and pro-peptide cleavage efficiency by introducing linker peptide in the C-terminus of the pro-peptide. J Ind Microbiol Biotechnol. 2013;40(3–4):317–325.10.1007/s10295-012-1221-y
- Chen K, Liu S, Zhang D, et al. Altered secretary efficiency of Streptomyces hygroscopicus transglutaminase in Escherichia coli by the pro-peptide modification. Process Biochem. 2013;48(5):782–787.10.1016/j.procbio.2013.04.001
- Gaspar AL, de Goes-Favoni SP. Action of microbial transglutaminase (MTGase) in the modification of food proteins: a review. Food Chem. 2015;171:315–322.10.1016/j.foodchem.2014.09.019
- Kieliszek M, Misiewicz A. Microbial transglutaminase and its application in the food industry. Folia Microbiol (Praha). 2014;59(3):241–250.10.1007/s12223-013-0287-x
- Rachel NM, Pelletier JN. Biotechnological applications of transglutaminases. Biomolecules. 2013;3(4):870–888.10.3390/biom3040870
- Zhu Y, Tramper J. Novel applications for microbial transglutaminase beyond food processing. Trends Biotechnol. 2008;26(10):559–565.10.1016/j.tibtech.2008.06.006
- Buettner K, Hertel TC, Pietzsch M. Increased thermostability of microbial transglutaminase by combination of several hot spots evolved by random and saturation mutagenesis. Amino Acids. 2012;42(2–3):987–996.10.1007/s00726-011-1015-y
- Yokoyama K, Utsumi H, Nakamura T, et al. Screening for improved activity of a transglutaminase from Streptomyces mobaraensis created by a novel rational mutagenesis and random mutagenesis. Appl Microbiol Biotechnol. 2010;87(6):2087–2096.10.1007/s00253-010-2656-6
- Marx CK, Hertel TC, Pietzsch M. Random mutagenesis of a recombinant microbial transglutaminase for the generation of thermostable and heat-sensitive variants. J Biotechnol. 2008;136(3–4):156–162.10.1016/j.jbiotec.2008.06.005
- Yang HL, Pan L, Lin Y. Purification and on-column activation of a recombinant histidine-tagged pro-transglutaminase after soluble expression in Escherichia coli. Biosci Biotechnol Biochem. 2009;73(11):2531–2534.10.1271/bbb.90422
- Grossowicz N, Wainfan E, Borek E, et al. The enzymatic formation of hydroxamic acids from glutamine and asparagine. J Biol Chem. 1950;187(1):111–125.
- Kashiwagi T, Yokoyama K, Ishikawa K, et al. Crystal structure of microbial transglutaminase from Streptoverticillium mobaraense. J Biol Chem. 2002;277(46):44252–44260.10.1074/jbc.M203933200
- Ando H, Adachi M, Umeda K, et al. Purification and characteristics of a novel transglutaminase derived from microorganisms. Agric Biol Chem. 1989;53(10):2613–2617.