Abstract
The hypO gene from Sinorhizobium meliloti, located within the trans-4-hydroxy-L-proline metabolic gene cluster, was first successfully expressed in the host Pseudomonas putida. Purified HypO protein functioned as a FAD-containing cis-4-hydroxy-D-proline dehydrogenase with a homomeric structure. In contrast to other known enzymes, significant activity for D-proline was found, confirming a previously proposed potential involvement in D-proline metabolism.
Approximately 25% of amino acid residues in mammalian collagens are L-proline, and nearly 40% are post-translationally converted into trans-4-hydroxy-L-proline (T4LHyp; generally called “L-hydroxyproline”). Free T4LHyp, produced by the degradation of collagen, is then converted into pyruvate and glyoxylate [Citation1]. In contrast to the well-known mammalian pathway, over 50 years after the discovery of bacteria capable of growing on T4LHyp as a sole carbon source, all metabolic enzymes (genes) involved in the hypothetical degradation of T4LHyp have been elucidated at the molecular level (supplemental Figure S1A) [Citation2,3] In this pathway, T4LHyp is converted into α-ketoglutarate via three intermediates including cis-4-hydroxy-D-proline (C4DHyp) through the step-wise actions of four enzymes, and their corresponding genes are often clustered in bacterial genomes (supplemental Figure S1B).
Two different types of C4DHyp dehydrogenases, catalyzing the second step in this pathway, have heteromeric and homomeric structures containing different prosthetic group(s): the former, only FAD; the latter (α4β4γ4), 2 FAD, FMN, and a [2Fe-2S]-iron sulfur cluster (supplemental Table S1) [Citation3,4]. β-subunit of the latter has full dehydrogenase activity by itself. Up to now, it is believed that their physiological role is limited to T4LHyp (and C4DHyp) metabolism; in fact, kcat/Km values of D-proline are more than 100-fold lower than that of C4DHyp. On the other hand, C4DHyp dehydrogenase has the ability to utilize artificial electron acceptors including p-iodonitrotetrazolium violet (INT) and nitroblue tetrazolium (NBT): a so-called “dye-linked flavin-containing dehydrogenase.” Such enzymes, and also C4DHyp dehydrogenase, are frequently associated with cell or organelle membranes and are unstable in solution, which has made their expression in host cells, purification, preservation, and characterization very difficult.
A cluster consisting of 14 hyp genes of Sinorhizobium meliloti, a famous N2-fixing bacteria, includes five operons: hypR, hypD, hypH, hypS-hypT, and hypM-hypN-hypP-hypQ-hypO-hyp(RE)-hypX-hypY-hypZ (supplemental Figure S1B) [Citation5–7]. It is likely that major source of T4LHyp for this bacteria in nature is the cell wall of plants (rather than collagen), in which a large amount of so-called “hydroxyproline-rich glycoprotein” is contained. Finan and coworkers have reported that most of them are involved in our originally characterized T4LHyp pathway described above. On the other hand, the hypothetical hypO gene (protein) is annotated as a D-amino acid dehydrogenase; only 35% sequence identity with the known homomeric enzyme from Pseudomonas putida [Citation3]. Furthermore, the genetic analysis indicates the potential involvement of both T4LHyp and D-proline (see below) [Citation7]. However, when even S. meliloti is used as a host, no expression of this recombinant protein is found, probably due to the dye-linked flavin-containing dehydrogenase [Citation7]. Therefore, to estimate the enzyme function and physiological role, we first expressed the hypO gene of S. meliloti (referred to as SmhypO gene) in P. putida cells, and characterized the biochemical activity.
The SmhypO gene (SM_b20267) was introduced into a BamHI-HindIII site in pQE-81L (Qiagen), a plasmid vector for attaching an N-terminal (His)6-tag to expressed protein, to obtain pQE/SmhypO. Next, a DNA fragment of the (His)6-SmhypO-t0 terminator was introduced into an XbaI-KpnI site in pUCP26KmAhpCp [Citation8], in order to obtain pUCP/SmhypO. P. putida KT2442-oxyR1 harboring pUCP/SmhypO was grown at 30 °C overnight in LB medium containing 50 mg/L rifampicin and 50 mg/L kanamycin. SmHypO protein was purified using Ni-NTA spin column (Qiagen), as described previously [Citation3], and used within 1 day for further experiments. C4Hyp dehydrogenase activity was measured by monitoring the reduction of INT (at 490 nm) or NBT (at 530 nm) at 30 °C in a reaction mixture with the following composition: 50 mM Tris-HCl buffer (pH 9.0), 0.06 mM phenazine methosulfate (PMS; electron-transfer intermediate), 0.25 mM INT or NBT, and 10 mM C4DHyp. One unit was defined as the amount of enzyme that catalyzed the reduction of 1 μmol of INT or NBT/min. For in-gel assay (zymogram staining analysis), the purified protein was separated on non-denaturing PAGE with 10% (w/v) gel at 4 °C, and the gel was then soaked in the reaction mixture described above. Protein concentrations were determined by the method of Lowry et al. [Citation9] with bovine serum albumin as the standard. The kinetic parameters, Km and kcat, were calculated using a Lineweaver–Burk plot.
The P. putida KT2442-oxyR1 strain has been shown to constitutively produce a soluble AhpC protein, a small subunit of alkyl hydroperoxide reductase [Citation10]. Therefore, we expressed (His)6-tagged SmHypO protein under regulation of the ahpC promoter in cells of this P. putida strain, and purified to homogeneity in a single step using immobilized metal (Ni2+) affinity chromatography and a buffer system containing Tween-20 because of tight binding to the cytoplasmic membrane: yield of ~0.6 mg per liter of the culture. SDS-PAGE showed a single band with a molecular mass of ~44 kDa, which corresponded to the theoretical value of SmHypO (43432.41 Da) (Figure (b)). However, it was impossible to determine the native molecular mass because of elution in the void volume of a gel-filtration column (data not shown). Purified SmHypO protein was yellow, and had 3.92 unit/mg protein of specific activity toward C4DHyp. Furthermore, the spectrum had the characteristics of a typical flavoprotein (maxima at approximately 350 and 450 nm; Figure (c)) and the flavin compound was identified as FAD only by high-pressure liquid chromatography (HPLC) using a TSKgel ODS-80Tm column (TOSOH) (Figure (d)). These results clearly indicated the function of SmHypO protein as a homomeric C4DHyp dehydrogenase. Optimum pH of the activity was estimated to be 9.5–9.75 using acetate-NaOH (pH 4–6), K2HPO4/KH2PO4 (pH 6–8.5), Tris-HCl (pH 7.5–9), and glycine-NaOH (pH 9–11) buffer.
Figure 1. Purification and characterization of SmHypO. (a) Library of proline derivatives related to this study. (b) SDS-PAGE analysis of the purified SmHypO recombinant protein (5 μg in a 12% (w/v) gel). (c) Absorption spectra. (d) HPLC analysis of the prosthetic group. Elution profiles of extracts of SmHypO (upper) and the standard mixture (lower). (e) Zymogram staining analysis used the indicated proline derivatives as substrates together with the PMS/INT or PMS/NBT assay system. Purified enzymes (50 μg) were applied on 10% (w/v) non-denaturing PAGE.
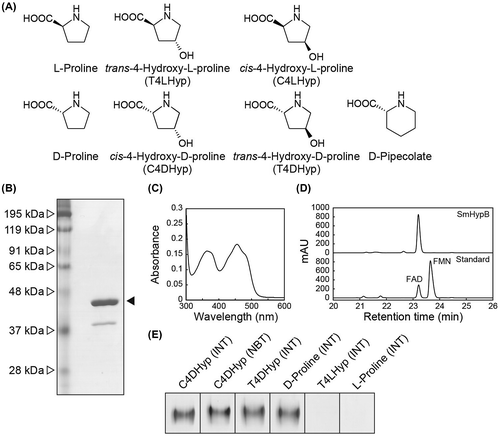
At 10 mM D-amino acid concentration, SmHypO utilized C4DHyp (100%), T4DHyp (73%), D-proline (71%), D-pipecolate (51%), D-methionine (1.8%), D-tryptophan (0.9%), and D-arginine (0.5%). L-Amino acids including L-proline and T4LHyp were inert as substrates. The enzyme was subjected to further kinetic analysis with the five former substrates and the determined parameters are listed in Table . The catalytic efficiency (kcat/Km) value with C4DHyp (665 min−1·mM−1) was 3.0-fold, 5.5-fold, and 62-fold higher than those with T4DHyp, D-proline, and D-pipecolate, mainly caused by 2.5-fold, 5.0-fold, and 88-fold lower Km values, respectively. On the other hand, the markedly low kcat/Km value with D-methionine (0.148 min−1·mM−1) was achieved not only by a 122-fold higher Km value, but also 37-fold lower kcat value. SmHypO had electron acceptor activity only in PMS/INT and PMS/NBT, but not 2,6-dichloroindophenol (Cl2Ind), ferricyanide, cytochrome c, and NAD(P)+, and a slight preference of PMS/NBT over PMS/INT was observed when C4DHyp, T4DHyp, and D-proline were used as a substrate. These substrate and electron acceptor specificities were also observed in the in-gel assay (zymogram staining; Figure (e)).
Table 1. Kinetic parameters of the SmHypO protein.
The greatest difference observed in the properties of homomeric enzymes from S. meliloti and P. putida (and the heteromeric enzymes) as C4DHyp dehydrogenases was that the former had significant activity for D-proline (and T4DHyp) (Table 1 and supplemental Table S1). Furthermore, D-pipecolate, a six-ring proline analog, was an additional substrate for SmHypO, which is the first report in the protein family including C4DHyp dehydrogenases (see below). Satomura and coworkers have reported that Rmar_0499 protein from Rhodothermus marinus JCM9785, whose gene is located within T4LHyp metabolic gene cluster, shows dye-linked dehydrogenation activities toward C4DHyp and D-proline (supplemental Table S1) [Citation11]. On the other hand, several D-amino acids including (the most preferable) D-phenylalanine are additional substrates: this enzyme may be a general D-amino acid dehydrogenase. These results indicated that SmHypO has a relative broad substrate specificity with several D-proline analogs, compared with that of known C4DHyp dehydrogenase enzymes, and that the recognition mechanism between C4DHyp and D-proline may be different from that of the R. marinus enzyme. However, it is unclear if these activities have any physiological meaning in S. meliloti. The hypO mutant of S. meliloti continues to grow on T4LHyp, but at half the wild-type rate, which may be due to alternative d-amino acid oxidases with broad specificity [Citation7]. On the other hand, the disruption of either hypO, hypMNPQ (encoding an ABC-type transporter; see supplemental Figure S1B), or hypS (encoding the Δ1-pyrroline-2-carboxylate (Pyr2C) reductase) leads to a significant decrease for hypO and hypMNPQ or complete elimination for hypS of growth not only on T4LHyp but also on D-proline [Citation6]. Furthermore, hypM and hypS (and probably hypO) are upregulated by D-proline relative to L-proline, but to a lesser extent than that by T4LHyp. Based on these insights, Finan and coworkers have speculated that the hyp gene cluster is also involved in D-proline metabolism (D-proline→Pyr2C→L-proline), in which the SmHypO protein may function as a D-proline dehydrogenase (supplemental Figure S1A) [Citation6]. Therefore, biochemical data in this study significantly supports this hypothesis.
Although both types of C4DHyp dehydrogenases (and D-amino acid dehydrogenase from R. marinus) belong to the D-amino acid oxidase superfamily (pfam01266), there is a poor phylogenetic relationship between them, and the SmHypO protein is clearly close to the known homomeric enzyme from P. putida. Sakuraba and coworkers [Citation12–15] reported several types of L-proline dehydrogenases from hyperthermophilic archaea with different subunit assembly and prosthetic groups (supplemental Table S1), among which enzymes from P. horikoshii and T. profundus have sequence similarity to heteromeric C4DHyp dehydrogenase [Citation3,12,13]. On the other hand, three-dimensional structures of enzymes from P. horikoshii (apo-form) and A. pernix (a complex with L-proline) are available [Citation12,15]. Different amino acid residues (Tyr251 and Leu289, respectively) are closely located to C4 position of the bound L-proline, which may be responsible for their distinguished activities for T4LHyp (supplemental Table S1). Since it was difficult to find equivalent amino acid residues with active sites of archaeal L-proline dehydrogenase in C4DHyp dehydrogenase due to their low sequential similarities, the crystallographic analysis would be necessary.
T4LHyp is an important marker for directly measuring the content of collagen in several biological samples by an amino acid analyzer (HPLC). We previously developed an alternative enzymatic method using a coupling system with hydroxyproline 2-epimerase and heteromeric C4DHyp dehydrogenase [Citation4], whereas the homomeric form of the latter may be more useful due to its simple structure. However, activity of SmHypO was gradually lost at 4 °C, and only 4.8% activity remained after 1 day, similar to the P. putida enzyme [Citation3]. Therefore, discovery of a more stable homomeric C4DHyp dehydrogenase would be helpful to further improve this method.
Author contribution
S. Watanabe conceived, designed, and performed the experiments, analyzed the data, and wrote the article. D. Morimoto and F. Fukumori partially performed the expression experiment of SmHypO protein in P. putida. Y. Watanabe contributed discussion to improve the article.
Disclosure statement
No potential conflict of interest was reported by the authors.
Funding
This work was supported by Japan Society for the Promotion of Science, [grant number KAKENHI/16K07297, KAKENHI/25440049] and Sumitomo Foundation [Basic Science Research Projects].
Supplemental data
Supplemental data for this article can be accessed at https://doi.org/10.1080/09168451.2017.1403887.
References
- Adams E, Frank L. Metabolism of proline and the hydroxyprolines. Annu Rev Biochem. 1980;49:1005–1061.10.1146/annurev.bi.49.070180.005041
- Watanabe S, Yamada M, Ohtsu I, et al. α-Ketoglutaric semialdehyde dehydrogenase isozymes involved in metabolic pathways of D-glucarate, D-galactarate and hydroxy-L-proline: molecular and metabolic convergent evolution. J Biol Chem. 2007;282:6685–6695.10.1074/jbc.M611057200
- Watanabe S, Morimoto D, Fukumori F, et al. Identification and characterization of D-hydroxyproline dehydrogenase and Δ1-pyrroline-4-hydroxy-2-carboxylate deaminase involved in novel L-hydroxyproline metabolism of bacteria: metabolic convergent evolution. J Biol Chem. 2012;287:32674–32688.10.1074/jbc.M112.374272
- Watanabe S, Hiraoka Y, Endo S, et al. An enzymatic method to estimate the content of L-hydroxyproline. J Biotechnol. 2015;199:9–16.10.1016/j.jbiotec.2015.01.026
- MacLean AM, White CE, Fowler JE, et al. Identification of a hydroxyproline transport system in the legume endosymbiont Sinorhizobium meliloti. Mol Plant Microbe Interact. 2009;22:1116–1127.10.1094/MPMI-22-9-1116
- White CE, Gavina JM, Morton R, et al. Control of hydroxyproline catabolism in Sinorhizobium meliloti. Mol Microbiol. 2012;85:1133–1147.10.1111/j.1365-2958.2012.08164.x
- Chen S, White CE, diCenzo GC, et al. L-Hydroxyproline and D-proline catabolism in Sinorhizobium meliloti. J Bacteriol. 2016;198:1171–1181.10.1128/JB.00961-15
- Kobayashi Y, Ohtsu I, Fujimura M, et al. A mutation in dnaK causes stabilization of the heat shock sigma factor σ32, accumulation of heat shock proteins and increase in toluene-resistance in Pseudomonas putida. Environ Microbiol. 2011;13:2007–2017.10.1111/j.1462-2920.2010.02344.x
- Lowry OH, Rosebrough NJ, Farr AL, et al. Protein measurement with the folin phenol reagent. J. Biol. Chem. 1951;193:265–275.
- Hishinuma S, Yuki M, Fujimura M, et al. OxyR regulated the expression of two major catalases, KatA and KatB, along with peroxiredoxin. AhpC in Pseudomonas putida. Environ Microbiol. 2006;8:2115–2124.10.1111/emi.2006.8.issue-12
- Satomura T, Ishikura M, Koyanagi T, et al. Dye-linked D-amino acid dehydrogenase from the thermophilic bacterium Rhodothermus marinus JCM9785: characteristics and role in trans-4-hydroxy-L-proline catabolism. Appl Microbiol Biotechnol. 2015;99:4265–4275.10.1007/s00253-014-6263-9
- Tsuge H, Kawakami R, Sakuraba H, et al. Ohshima T crystal structure of a novel FAD-, FMN-, and ATP-containing L-proline dehydrogenase complex from Pyrococcus horikoshii. J Biol Chem. 2005;280:31045–31049.10.1074/jbc.C500234200
- Kawakami R, Sakuraba H, Ohshima T. Gene and primary structures of dye-linked L-proline dehydrogenase from the hyperthermophilic archaeon Thermococcus profundus show the presence of a novel heterotetrameric amino acid dehydrogenase complex. Extremophiles. 2004;8:99–108.10.1007/s00792-003-0368-x
- Satomura T, Zhang XD, Hara Y, et al. Characterization of a novel dye-linked L-proline dehydrogenase from an aerobic hyperthermophilic archaeon, Pyrobaculum calidifontis. Appl Microbiol Biotechnol. 2011;89:1075–1082.
- Sakuraba H, Satomura T, Kawakami R, et al. Crystal structure of novel dye-linked L-proline dehydrogenase from hyperthermophilic archaeon Aeropyrum pernix. J Biol Chem. 2012;287:20070–20080.10.1074/jbc.M111.319038