Abstract
In Caenorhabditis elgans, insulin-like peptides have significant roles in modulating larval diapause and adult lifespan via the insulin/IGF-1 signaling (IIS) pathway. Although 40 insulin-like peptides (ILPs) have been identified, it remains unknown how ILPs act as either agonists or antagonists for their sole receptor, DAF-2. Here we found 1) INS-23 functions as an antagonistic ILP to promote larval diapause through the IIS pathway like a DAF-2 antagonist, INS-18, 2) INS-23 and INS-18 have similar biochemical functions. In addition, our molecular modeling suggests that INS-23 and INS-18 have characteristic insertions in the B-domain, which are crucial for the recognition of the insulin receptor, when compared with DAF-2 agonists. These characteristic insertions in the B-domain of INS-23 and INS-18 would modulate their intermolecular interactions with the DAF-2 receptor, which may lead these molecules to act as antagonistic ligands. Our study provides new insight into the function and structure of ILPs.
The insertion of proline residues in the B-domain of INS-23 and INS-18, insulin-like peptides of C. elegans, may specify their antagonistic functions.
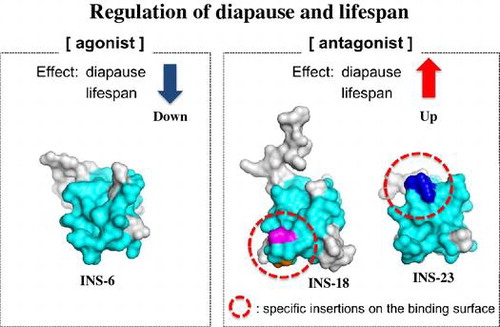
Abbreviations:
The insulin/IGF-1 signaling (IIS) pathway, which is highly conserved among the animal kingdom [Citation1], has significant roles in controlling larval diapause and adult lifespan through the sole insulin receptor-like protein DAF-2 and the forkhead box O (FOXO) transcription factor DAF-16 in Caenorhabditis elegans [Citation2–6]. To date, 40 insulin-like peptides (ILPs) have been identified as putative DAF-2 ligands, and are classified into types-α, -β, and -γ, based on the number and patterns of disulfide bonds [Citation7–9]. For example, INS-18, which is a type-γ ILP, functions as a DAF-2 antagonist to promote larval diapause and longevity [Citation10]. In contrast, INS-6, INS-7, and DAF-28, which are type-β ILPs, function as DAF-2 agonists to suppress larval diapause and/or longevity [Citation11–13]. In our previous study, we performed an RNAi screen of type-α ILPs modulating larval diapause, and found a possibility that INS-23 functions as an antagonistic ILP [Citation14]. Furthermore, Fernandes de Abre and co-workers attempted to elucidate the mechanisms by which the 40 C. elegans ILPs coordinate diverse physiological processes, such as dauer formation and longevity [Citation15]. As described above, agonistic and antagonistic ILPs modulate larval diapause and/or adult lifespan. However, it remains unknown how ILPs can act as either agonists or antagonists for their sole DAF-2 receptor to modulate the physiological functions. In this report, we demonstrate the physiological functions and expression pattern of an antagonistic ILP, INS-23, compared with those of INS-18, as well as the importance of their gene promoters for determination of their physiological function. We also discuss how INS-23 and INS-18 function as antagonists for the DAF-2 receptor, based upon their predicted tertiary structures.
Materials and methods
Nematode strains
N2 (Bristol) is the primary wild-type strain, and standard growth conditions were used [Citation16]. The following alleles were used in this study: ins-18(tm339)I, and ins-23(tm1875)III. The double mutant animals, ins-18(tm339); ins-23(tm1875), were generated by crossing. To check for deletions of mutant animals, we carried out single-worm PCR using the primers listed in Table S1. The homozygous mutants were outcrossed five times to N2 before further analysis.
Construction of plasmids
The following plasmids were created:
*ins-23 transgene (ins-23p::ins-23): A 3.0-kb genomic fragment containing the ins-23 coding region and 1.8 kb of the upstream sequence was inserted into the pGEM®-T easy vector (Promega).
*ins-18 transgene (ins-18p::ins-18) was created as described previously [Citation10].
*ins-23 promoter::venus (ins-23p::venus): A 1.8-kb promoter region upstream to a site before the start codon was inserted into the pPD_venus [Citation17] vector, using SphI and SalI sites.
*ins-18 promoter::mrfp (ins-18p::mrfp) was created as described previously [Citation10].
*ins-23p::ins-18: A 4.4-kb fragment without the ins-23 region was amplified by inverse PCR from the ins-23p::ins-23 plasmid. An additional 2.3-kb fragment containing the ins-18 region was amplified from the ins-18p::ins-18 plasmid. The amplified fragments were then ligated using a PstI site.
*ins-18p::ins-23: A 7.2-kb fragment without the ins-18 region was amplified by inverse PCR from the ins-18p::ins-18 plasmid. An additional 1.1-kb fragment containing the ins-23 region was amplified from the ins-23p::ins-23 plasmid. The amplified fragments were then ligated using the In-Fusion® HD Cloning Kit (TaKaRa).
*ins-23p::ins-23::mrfp: A 2.1-kb genomic fragment containing the ins-23 coding region and 1.8 kb of the upstream sequence was inserted into pHK_mRFP vector, using SphI and BamHI sites.
*ins-23p::ins-18::venus: A 5.4-kb fragment without the ins-23::mrfp region was amplified by inverse PCR from the ins-23p::ins-23::mrfp plasmid. An additional 3.1-kb fragment containing the ins-18::venus region was amplified from the ins-18p::ins-18::venus plasmid. The amplified fragments were then ligated using an EcoRI site.
*ins-18p::ins-18::venus was created as described previously [Citation10].
*ins-18p::ins-23::mrfp: An 8.3-kb fragment without the ins-18::venus region was amplified by inverse PCR from the ins-18p::ins-18::venus plasmid. An additional 1.1-kb fragment containing the ins-23::mrfp region was amplified from the ins-23p::ins-23::mrfp plasmid. The amplified fragments were then ligated using an EcoT22I site.
The primers are listed in Table S1. Each plasmid was verified by sequencing.
Transgenic worms
The plasmids (100 ng/μL) were injected into the gonads [Citation18] of wild-type or mutant animals, along with a transformation marker (100 ng/μL), in the following combinations: ins-23p::ins-23 + eft-3p::venus into N2, ins-18(tm339), and ins-23(tm1875); ins-18p::ins-18 + eft-3p::venus into N2 and ins-23(tm1875); ins-23p::venus + ins-18p::mrfp + pRF4 [rol-6(su1006)] into N2; ins-23p::ins-23::mrfp + ins-23p::ins-18::venus + pRF4 [rol-6(su1006)] into N2; ins-18p::ins-18::venus + ins-18p::ins-23::mrfp + pRF4 [rol-6(su1006)] into N2. The animals injected with eft-3p::venus (100 ng/μl) as a co-injection marker did not have any influence on dauer formation and adult lifespan.
Microscopy
All 3D reconstruction images were obtained using an FV10i confocal laser scanning microscope (Olympus, Japan) and FV10-AWS software (Olympus).
Dauer assay
Dauer formation was evaluated in the presence of a crude pheromone extract [Citation19] that induces larval diapause. Nematode growth media (NGM) plates containing 1% crude pheromone extract (v/v) were seeded with E. coli OP50 bacteria, and then ∼20 worms were placed on each plate. C. elegans strains were semi-synchronized by allowing gravid adults to lay eggs for 3 h at 20 °C and then the adult worms were removed from the plate. After cultivating for 72 h at 25 °C, dauers and non-dauers were identified using a microscope and counted. Dauer larvae were identified by the presence of dark pigment granules, constriction of body and pharynx, and loss of pharyngeal pumping. For the dauer formation assays, 3–4 independent plates were assayed as one trial. Multiple comparisons were carried out using one way analysis of variance (ANOVA) followed by Turkey’s HSD multiple comparison test using SPSS (IBM Statistics for Windows, version 23.0. IBM Corp, Tokyo, Japan). p <0.05 was considered to be statistically significant.
Lifespan assay
Synchronous L4-stage worms estimated as 0-day adults were incubated on NGM containing 40 μM 5-fluorodeoxyuridine (FUdR) to prevent self-fertilization. The number of surviving worms was monitored until death. The following method was used to determine whether or not a nematode was dead. One microliter of distilled water was dropped onto each worm, and the worm was observed for movement for 5 s. If the worm did not show any signs of wriggling and/or head shaking during the observation period, it was determined to be deceased. The results of the survival assays were analyzed by the Kaplan-Meier method and significance was measured by the log-rank test using the statistical analysis add-in soft for Excel, Excel Statistics 2010, (SSRI). P values were determined using log-rank statistics and values less than 0.05 were considered statistically significant. The asterisk indicates the standard using statistics.
Molecular modeling
Molecular modeling was performed with Modeller [Citation20]. Electrostatic potentials were calculated with PDB2PQR [Citation21] and APBS [Citation22]. Molecular models were drawn with PyMOL [Citation23].
Results and discussion
INS-23 promotes larval diapause through the IIS pathway
In our previous study, our RNAi screen for type-α ILPs revealed that ins-23 RNAi reduces dauer formation [Citation14]. To verify the physiological function of INS-23 in dauer formation, we measured the rate of dauer formation using ins-23(tm1875). This allele has a 1284-bp deletion and 8-bp insertion in the ins-23 coding region, indicating that ins-23(tm1875) is a loss-of-function mutant. As shown in (Figure A), in the presence of dauer pheromones, ins-23(tm1875) mutants showed a reduction in dauer formation compared with the wild-type animals. This reduction was completely rescued by the injection of the ins-23 transgene (ins-23p::ins-23). Additionally, overexpression of ins-23 promoted dauer formation. These results suggest that normal function of INS-23 promotes larval diapause.
Figure 1. INS-23 functions to promote dauer formation via DAF-16. (A and B) Relative percentages of dauer formation in each mutant animal compared with N2 are shown in the presence of dauer pheromones. Data are expressed as the mean ±S.E. #p <0.05; N.S. Not Significant. n >150.
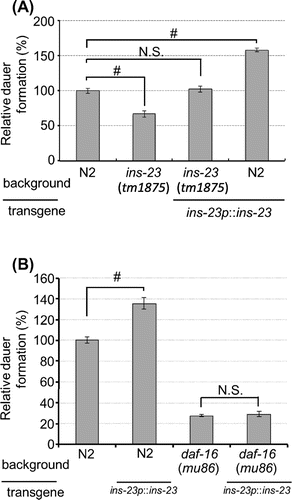
Next, we examined whether INS-23 modulates larval diapause through the IIS pathway. DAF-16, which is the sole C. elegans forkhead box O (FOXO) homolog, functions as a transcription factor, and acts in the IIS pathway that regulates dauer formation and longevity [Citation3–5] In the presence of dauer pheromones, overexpression of ins-23 promoted dauer formation in the wild-type background. In contrast, this promotion was completely suppressed in DAF-16(mu86) (Figure B). This result suggests that the modulation of larval diapause by INS-23 depends on the IIS pathway. It has been reported that INS-18, an antagonistic ILP of the DAF-2 receptor [Citation2] in the IIS pathway, functions to promote larval diapause [Citation10]. Therefore, INS-23 probably functions to promote larval diapause as a DAF-2 antagonist in the IIS pathway.
In addition to the modulation of larval diapause, the IIS pathway has an important role in regulating adult lifespan [Citation24]. To investigate whether INS-23 modulates adult lifespan, we measured adult lifespan. As shown in Figure S1, there were no significant differences observed among wild-type animals, ins-23(tm1875) mutants, or wild type animals harboring the ins-23 transgene. These results suggest that INS-23 might not contribute to the modulation of adult lifespan.
INS-23 modulates larval diapause cooperatively with INS-18
We demonstrated that INS-23 functions to promote larval diapause like INS-18. It has been reported that 1) INS-6 and DAF-28, expressed in the same neuronal cells, cooperatively modulate larval diapause [Citation13]; and 2) INS-7 and INS-35, yielded in the intestine, cooperatively modulate larval diapause [Citation14]. Thus, it seems likely that different ILPs, produced in the same cells or tissue, modulate larval diapause cooperatively. To investigate whether INS-23 modulates larval diapause cooperatively with INS-18, we first observed the expression patterns of ins-23p::VENUS and ins-18p::mRFP at the dauer arrest stage. As shown in Figure S2, these fluorescent proteins were observed in head and tail neurons. In addition, their signals were merged in these cells. Therefore, we concluded that ins-23 and ins-18 are expressed in the same neuronal cells of the head and tail.
We next assessed physiological function of INS-23 and INS-18 in larval diapause. In the presence of dauer pheromones, each single mutant showed suppression of dauer formation compared to the wild-type animals. Moreover, ins-23;ins-18 double mutants showed suppression of dauer formation, more so than either single mutant (Figure A). These results suggest that INS-23 and INS-18 cooperatively modulate larval diapause. We also demonstrated that both ins-23 and ins-18 mutants show a similar degree of suppression in dauer formation (Figure A). It is possible that INS-23 is functionally equivalent to INS-18 in the modulation of dauer formation. To assess their functions, we examined whether overexpression of one ILP could compensate for the loss of the other ILP. In the presence of dauer pheromones, the phenotype of ins-18(tm339) was fully rescued by injection of the ins-23 transgene (ins-23p::ins-23). In addition, the reciprocal experiment showed that the phenotype of ins-23(tm1875) was also rescued by injection of the ins-18 transgene (ins-18p::ins-18) (Figure B). Therefore, INS-23 and INS-18 are functionally exchangeable in the modulation of dauer formation.
INS-23 expressed by the ins-18 promoter promotes longevity
We demonstrated that INS-23 and INS-18 have a similar function in the modulation of dauer formation. In contrast, INS-23 did not contribute to the modulation of adult lifespan even though INS-18 does. Therefore, we hypothesized that their expression level and/or expression patterns were different at the adult stage. To address our hypothesis, we observed the expression patterns of ins-23p::VENUS and ins-18p::mRFP at the adult stage. Each fluorescent protein was observed in head neurons. In addition, their signals were merged. ins-23p::VENUS was also observed in the gonads. On the other hand, ins-18p::mRFP was observed in intestine (Figure S3). It has been reported that the intestinal IIS pathway plays important roles in controlling adult lifespan [Citation25]. In addition, it has been reported that the expression level of ins-23 is 5 times lower than that of ins-18 at the adult stage [Citation26]. Therefore, it is possible that low gene expression and lack of intestinal expression of ins-23 reduces its contribution to the modulation of adult lifespan.
Expression level and expression pattern of genes are generally determined by their promoter regions. Therefore, we wondered whether INS-23 expressed by the ins-18 promoter sequence could contribute to the modulation of adult lifespan. To address this question, we measured adult lifespan in wild type, wild type harboring ins-18p::ins-18, and wild type harboring ins-18p::ins-23. Wild-type animals harboring ins-18p::ins-18 exhibited an extended adult lifespan compared with the wild-type animals. Interestingly, wild-type animals harboring ins-18p::ins-23 also exhibited an extended adult lifespan. In addition, they showed a similar degree of lifespan-extension (Figure A). Therefore, INS-23 expressed by the ins-18 promoter could contribute to the modulation of adult lifespan. On the other hand, the reciprocal experiment showed that wild-type animals harboring ins-23p::ins-23 did not exhibit an extended adult lifespan. Interestingly, although ins-18p::ins-18 exhibited an extended adult lifespan, wild-type animals harboring ins-23p::ins-18 lost extension of the adult lifespan (Figure B). To confirm the expression of ins-23p::INS-18 and ins-18p::INS-23 in worms, respectively, we observed corresponding fusion proteins (ins-23p::INS-18::VENUS and ins-18p::INS-23::mRFP) at the adult stage. ins-23p::INS-18::VENUS and ins-23p::INS-23::mRFP were found in same cells. In the same way, ins-18p::INS-23::mRFP and ins-18p::INS-18::VENUS were found in same cells (Figure S4). These results suggested that ins-23p::INS-18 and ins-18p::INS-23 are expressed in the worms.
Taken together, (1) the ins-18 promoter might have sequences that are important for the modulation of adult lifespan, and (2) INS-23 and INS-18 have similar biochemical function.
INS-23 and INS-18 have characteristic insertions on their putative receptor-binding surfaces
We demonstrated that INS-23 and INS-18, driven by the ins-18 promoter, promote not only larval diapause but also longevity to the same degrees. We assumed that INS-23 and INS-18 have similar structures essential to their functions as antagonists.
To elucidate the structural similarity between INS-23 and INS-18, we first aligned amino acid sequences of INS-23, INS-18, human insulin, and INS-6, a DAF-2 agonist (opposite to INS-23 and INS-18) that structurally resembles human insulin and can activate the human insulin receptor [Citation27]. INS-23 and INS-18 have insertion sequences in the B-domain when compared with agonistic ligands, insulin, and INS-6 (Figure A). INS-18 has a three-residue insertion (PPG: blue) after the first cysteine residue of the B-domain. On the other hand, INS-23 has two proline-residue insertions (PGVP: orange and purple) after the second cysteine residue of the B-domain. The molecular models of INS-23, INS-18, and INS-6 were built (Figure B) based on the crystal structure of insulin and the sequence alignment (Figure A). For mammalian insulin and IGF-I, the amino acid residues involved in receptor binding have been identified by the ligand-receptor co-crystal structures (PDB: 3W11, 3W12, 3W13, 4OGA, 4XSS, 5KQV). Based on this information, the putative DAF-2 binding residues of INS-23, INS-18, and INS-6 are proposed and colored cyan (Figure AB). As shown in Figure B, the putative DAF-2-binding residues form a molecular surface, and both the insertion sequences of INS-23 (orange and purple) and INS-18 (blue) are located on “the putative DAF-2-binding surface”. In Figure C, the electrostatic surface potentials of INS-23, INS-18, and INS-6 are displayed. Both insertion sequences of INS-23 and INS-18 are electrostatically neutral, and partly because of these insertions, the electrostatic surface potentials of INS-23 and INS-18 are apparently different from that of INS-6. Thus, each insertion sequence of INS-23 (PxxP) and INS-18 (PPG) in the B domain modulate both the shape and the electrostatic potential of these molecules, which may lead these molecules to act as antagonistic ligands.
Figure 3. Promoter-dependent lifespan extension of INS-23 and INS-18. Survival curves of wild-type and each mutant animals are shown. (A) INS-23 and INS-18 driven by the ins-18 promoter extend lifespan. (B) INS-23 and INS-18 driven by the ins-23 promoter do not extend lifespan. Detailed data are shown in Table S3.
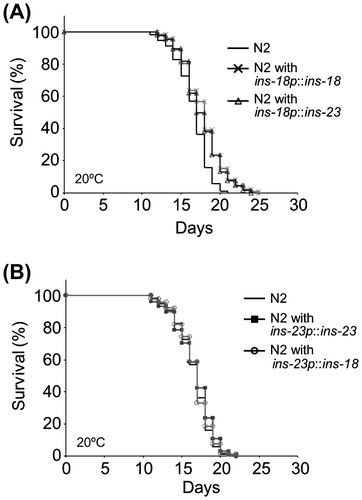
Figure 4. INS-23 and INS-18 have insertions on their putative receptor-binding surfaces. (A) Alignment of human insulin, INS-6, INS-18, and INS-23. (B) Predicted tertiary structures of INS-6, INS-18, and INS-23. The putative interaction residues with DAF-2 are colored cyan. INS-18 has a PPG insertion (blue), while INS-23 has a PxxP insertion (orange and purple). (C) Electrostatic surface potentials of INS-6, INS-18, and INS-23.
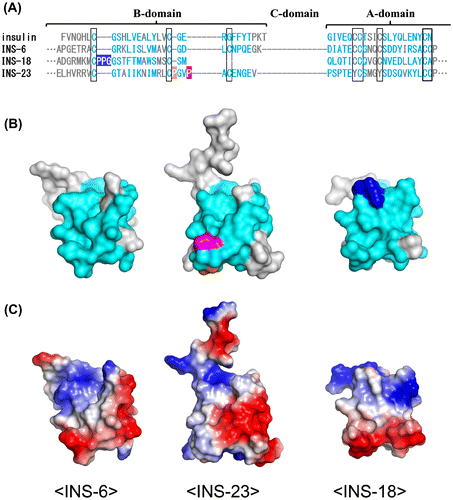
In this study, we found that INS-23 is functionally equivalent to INS-18 as an antagonist in the modulation of larval diapause and adult lifespan. In addition, INS-23 and INS-18 have insertions on the putative binding surface of the DAF-2 receptor, when compared with a DAF-2 agonist, INS-6. Although the physiological functions of ILPs have been revealed by integrated analysis [Citation15], it has not yet been revealed how ILPs could act as antagonists. Our study provides new insight into the function and structure of ILPs.
Author contributions
YM, TM, TI, TK conceived and designed the experiments. YM and TM mainly performed the experiments. KN performed molecular modeling. YM, TM, TI, KN, TK analyzed the data. YM, KN, TK co-wrote the paper. YM, TM, TI, KN, TK reviewed and edited the manuscript. TK was responsible for the overall design of the research and experiments. All authors read and approved this paper.
Disclosure statement
No potential conflict of interest was reported by the authors.
Funding
This work was supported by JSPS KAKENHI [grant number 23580150], [grant number 26292061] (to T.K.); the Platform for Drug Discovery, Informatics, and Structural Life Science (PDIS) from MEXT and AMED (to K.N.).
Supplemental data
Supplemental data for this article can be accessed [https://doi.org/10.1080/09168451.2017.1415749].
Corrected_supplemental_data.pdf
Download PDF (2.2 MB)Acknowledgement
We are grateful to Takeshi Ishihara of Kyushu University and Hiroshi Kagoshima of the National Institute of Genetics for providing us with the reporter plasmids pPD_venus and pHK_mrfp, respectively. We also thank James M. Kramer of Northwestern University for providing us with the marker plasmid pRF4. Some strains were provided by the Caenorhabditis Genetics Center funded by the National Institutes of Health National Center for Research Resources (NCRR) and the National Bioresources Project of Japan for the nematode.
References
- Fontana L, Klein S, Holloszy JO. Effects of long-term calorie restriction and endurance exercise on glucose tolerance, insulin action, and adipokine production. Age (Dordr). 2010;32:97–108.10.1007/s11357-009-9118-z
- Kimura KD, Tissenbaum HA, Liu Y, et al. daf-2, an insulin receptor-like gene that regulates longevity and diapause in Caenorhabditis elegans. Science. 1997;277:942–946.10.1126/science.277.5328.942
- Ogg S, Paradis S, Gottlieb S, et al. The Fork head transcription factor DAF-16 transduces insulin-like metabolic and longevity signals in C. elegans. Nature. 1997;389:994–999.10.1038/40194
- Henderson ST, Johnson TE. daf-16 integrates developmental and environmental inputs to mediate aging in the nematode Caenorhabditis elegans. Curr Biol. 2001;11:1975–1980.10.1016/S0960-9822(01)00594-2
- Lee RY, Hench J, Ruvkun G. Regulation of C. elegans DAF-16 and its human ortholog FKHRL1 by the DAF-2 insulin-like signaling pathway. Curr Biol. 2001;11:1950–1957.10.1016/S0960-9822(01)00595-4
- Kenyon C. A pathway that links reproductive status to lifespan in Caenorhabditis elegans. Ann N Y Acad Sci. 2010;1204:156–162.10.1111/j.1749-6632.2010.05640.x
- Pierce SB, Costa M, Wisotzkey R, et al. Regulation of DAF-2 receptor signaling by human insulin and ins-1, a member of the unusually large and diverse C. elegans insulin gene family. Genes Dev. 2001;15:672–686.10.1101/gad.867301
- Li W, Kennedy SGRuvkun G. DAF-28 encodes a C. elegans insulin superfamily member that is regulated by environmental cues and acts in the DAF-2 signaling pathway. Genes Dev. 2003;17:844–858.10.1101/gad.1066503
- Husson SJ, Mertens I, Janssen T, et al. Neuropeptidergic signaling in the nematode Caenorhabditis elegans. Prog Neurobiol. 2007;82:33–55.10.1016/j.pneurobio.2007.01.006
- Matsunaga Y, Gengyo-Ando K, Mitani S, et al. Physiological function, expression pattern, and transcriptional regulation of a Caenorhabditis elegans insulin-like peptide, INS-18. Biochem Biophys Res Commun. 2012;423:478–483.10.1016/j.bbrc.2012.05.145
- Malone EA, Inoue T, Thomas JH. Genetic analysis of the roles of DAF-28 and age-1 in regulating Caenorhabditis elegans dauer formation. Genetics. 1996;143:1193–1205.
- Murphy CT, McCarroll SA, Bargmann CI, et al. Genes that act downstream of DAF-16 to influence the lifespan of Caenorhabditis elegans. Nature. 2003;424:277–283.10.1038/nature01789
- Cornils A, Gloeck M, Chen Z, et al. Specific insulin-like peptides encode sensory information to regulate distinct developmental processes. Development. 2011;138:1183–1193.10.1242/dev.060905
- Matsunaga Y, Honda Y, Honda S, et al. Diapause is associated with a change in the polarity of secretion of insulin-like peptides. Nat Commun. 2016;7:10573.10.1038/ncomms10573
- Fernandes de Abreu DA, Caballero A, Fardel P, et al. An insulin-to-insulin regulatory network orchestrates phenotypic specificity in development and physiology. PLoS Genet. 2014;10:e1004225.10.1371/journal.pgen.1004225
- Brenner S. The genetics of Caenorhabditis elegans. Genetics. 1974;77:71–94.
- Nagai T, Ibata K, Park ES, et al. A variant of yellow fluorescent protein with fast and efficient maturation for cell-biological applications. Nat Biotechnol. 2002;20:87–90.10.1038/nbt0102-87
- Mello C, Fire A. DNA transformation. Methods Cell Biol. 1995;48:451–482.10.1016/S0091-679X(08)61399-0
- Kawano T, Kataoka N, Abe S, et al. Lifespan extending activity of substances secreted by the nematode caenorhabditis elegans that include the dauer-inducing pheromone. Biosci Biotechnol Biochem. 2005;69:2479–2481.10.1271/bbb.69.2479
- Webb B, Sali A. Comparative protein structure modeling using modeller. Curr Protoc Bioinf. 2014;47:1–32.
- Dolinsky TJ, Czodrowski P, Li H, et al. PDB2PQR: expanding and upgrading automated preparation of biomolecular structures for molecular simulations. Nucleic Acids Res. 2007;35:W522–W525.10.1093/nar/gkm276
- Baker NA, Sept D, Joseph S, et al. Electrostatics of nanosystems: application to microtubules and the ribosome. Proc Natl Acad Sci U S A. 2001;98:10037–10041.10.1073/pnas.181342398
- DeLano WL. Unraveling hot spots in binding interfaces: progress and challenges. Curr Opin Struct Biol. 2002;12:14–20.10.1016/S0959-440X(02)00283-X
- Kenyon C. Environmental factors and gene activities that influence life span. In: Riddle DL, Blumenthal T, Meyer BJ, Priess JR, editors. C. elegans II. 2nd ed. Cold Spring Harbor (NY): Cold Spring Harbor Laboratory Press. 1997.
- Libina N, Berman JR, Kenyon C. Tissue-specific activities of C. elegans DAF-16 in the regulation of lifespan. Cell. 2003;115:489–502.10.1016/S0092-8674(03)00889-4
- Baugh LR, Kurhanewicz N, Sternberg PW. Sensitive and precise quantification of insulin-like mRNA expression in caenorhabditis elegans. PLoS ONE. 2011;6:e18086.10.1371/journal.pone.0018086
- Hua QX, Nakagawa SH, Wilken J, et al. A divergent INS protein in Caenorhabditis elegans structurally resembles human insulin and activates the human insulin receptor. Genes Dev. 2003;17:826–831.10.1101/gad.1058003