Abstract
Cowpea seed β-vignin, a vicilin-like globulin, proved to exert various health favourable effects, including blood cholesterol reduction in animal models. The need of a simple scalable enrichment procedure for further studies for tailored applications of this seed protein is crucial. A chromatography-independent fractionation method allowing to obtain a protein preparation with a high degree of homogeneity was used. Further purification was pursued to deep the molecular characterisation of β-vignin. The results showed: (i) differing glycosylation patterns of the two constituent polypeptides, in agreement with amino acid sequence features; (ii) the seed accumulation of a gene product never identified before; (iii) metal binding capacity of native protein, a property observed only in few other legume seed vicilins.
Using a shorted protocol, -vignin with a high degree of purity was obtain. A glycolylated gene product, never identified before, and its metal binding capacity were identified.
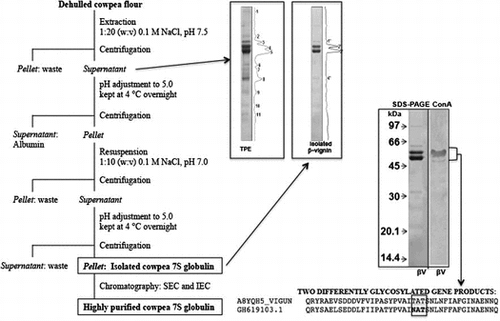
The nutritional relevance of grain legumes primarily relies upon their high contents of protein, which vary from 200 to 300 g/kg in peas and beans up to 380–400 g/kg in soybean and lupin. Legume proteins are gaining interest as ingredients in food systems too, especially in the industrialized countries, where a growth in per capita consumption of grains has been observed [Citation1]. According to Alonso et al. [Citation2], a number of desirable features of legume proteins, which affect both the quality and behaviour of proteins in food systems during processing, manufacturing, storage and preparation, are required for their optimal food utilisation. In turn, the search for novel seed protein sources stimulates research studies aiming at the characterisation and improved isolation of proteins from under-utilized legumes. Furthermore, health-promoting biological activities and regulatory functions in human body have been related to several protein components of plant origin in recent years. Plant peptides of different sizes and even intact proteins were shown to exert various beneficial effects in humans, notably in bone and cardiovascular health, inflammation, cancer, weight control, insulin sensitivity and immune-cell activity [Citation3].
Cowpea (Vigna unguiculata, L. Walp) seeds are among the most relevant Vigna species for human food. Cowpea bean is cultivated and primarily consumed as a food in tropical and subtropical zones of the world, including Africa, Asia and Latin America [Citation4] and particularly in North Eastern Brazil [Citation5]. Despite the rich protein content of cowpea (210 g to 340 g/kg) and a high concentration of calcium, potassium, iron, zinc and copper [Citation5], as well as a number of positive agronomic features, including drought resistance and adaptation to poor soils, Vigna seeds are not widely consumed yet. The incomplete appreciation of the molecular, technological, nutritional and nutraceutical properties of Vigna seed components is one of the reasons of this limitation [Citation6].
As for most legume seeds, the main protein fraction of cowpea seeds is represented by globulins which accounts for 51 to 72% of total seed proteins. The most represented protein type is β-vignin, which belongs to the 7S globulin or vicilin-like family [Citation7–10]. The 7S globulins of Vigna species show 62% amino acid sequence identity with soybean β-conglycinin α’ subunit, which proved to be effective in lowering blood cholesterol and triglycerides levels in humans and animals [Citation11–14]. Indeed, recent results by our research groups have shown a specific hypocholesterolaemic effect of rat-administered oral doses of β-vignin isolated from cowpea [Citation15]. Other authors observed that the hydrolysis product of total protein extract from cowpea favourably affects lipid metabolism, through the inhibition of cholesterol synthesis [Citation16].
The study of cowpea β-vignin molecular properties has already been approached by few authors [Citation8,17]. The techno-functional results are reported by Horax et al. [Citation18] and Zhang et al. [Citation19]. However, improved or simplified protocols for protein fractionation, as well as the full characterisation and evaluation of the nutritional and nutraceutical properties of this protein are still scarcely investigated. In this work, we describe a simplified procedure, which allowed the preparation of highly purified β-vignin from cowpea seed. Moreover, its further molecular characterisation led us to identify novel molecular features such as the glycosylation pattern and metal binding capacity.
Material and methods
Plant material and chemicals
Cowpea (V. unguiculata, L. Walp) seeds were kindly provided by Dr. Rogério Faria Vieira of the Centro Tecnológico da Zona da Mata da Empresa de Pesquisa Agropecuária de Minas Gerais (Viçosa, MG, Brazil). All chemicals were from Sigma-Aldrich® (Milan, Italy), if not otherwise stated.
Structural homology by bioinformatic analyses
The available sequences of cowpea β-vignin were obtained from NCBI and UniProtKB. The following entries were used: NCBI Blast: AM905848; UniProtKB: A8YQH5_VIGUN for 7S-cowpea. All sequences are classified as “fragment”. The sequences of 7S-adzuki globulin by UniProtKB: A4PI98_PHAAN; soybean β-conglycinin subunit was that of the α′ subunit UniProtKB: P11827_SOYBN and the sequence of lupin 7S globulin was UniProtKB: Q53HY0_LUPAL. The sequences were aligned by Clustal O and Lalign at https://www.expasy.org; prediction of N-glycosylation was carried out by Net-N-Glyc 1.0 Server at http://www.cbs.dtu.dk/services/NetNGlyc/; Protein Parameters at https://www.expasy.org was used to calculate theoretical pI and molecular mass of the polypeptide chains.
Extraction and isolation of raw β-vignin from cowpea seeds
Cowpea seeds were first imbibed in distilled water for 10 h. Subsequently, the hull was removed manually. The seeds were then placed in an oven with air circulation at 40 °C for 12 h. Then, the seeds were milled to a flour and sieved through a 60 mesh nylon filter. The flour obtained was not defatted.
The whole procedure is schematically shown in Figure . The procedure consisted in the extraction of the flour with 0.1 M NaCl solution in the ratio 1/20 (w/v), adjusted to pH 7.5 with 2 M NaOH. Stirring was applied at room temperature for 1 h. The suspension was then filtered through a nylon filter as above and then centrifuged at 10,000 g for 40 min at the same temperature. From this step on, all procedures were carried out at 4 °C. The supernatant was diluted 1/1 with distilled water; pH was adjusted to 5.0 with 2 M HCl and the solution was kept overnight at 4 °C. A further centrifugation step as above allowed obtaining a soluble fraction (albumins) and a pellet. The pellet was suspended in water 1:20 w(initial weight)/v and stirred at 4 °C for 10 min. Then, the pH was adjusted to 5.0 and the suspension centrifuged as above. The new pellet was dissolved in 0.1 M NaCl, 1:10 w(initial weight)/v at pH 7.0 and stirred at 4 °C for 20 min. After further centrifugation as above, the pellet was discarded and the supernatant was diluted 1/1 v/v with distilled water; the pH was adjusted to 5.0 and the solution was kept at 4 °C overnight. The latter centrifugation step allowed recovering a pellet mainly consisting of β-vignin. The pellet was dissolved in 0.2 M NaCl, at pH 7.0, and dialyzed against distilled water prior to freeze-drying.
Protein concentration
Protein concentration was determined by the method of Lowry et al. [Citation20], using bovine serum albumin (BSA) as a reference.
Chromatographic steps of cowpea β-vignin extensive purification
Aliquots of freeze dried β-vignin were solubilised in 50 mM potassium phosphate buffer, pH 7.5, containing 0.5 M NaCl and 0.01% NaN3, and fractionated by size exclusion on Sepharose CL-6B column (1.0 cm × 100 cm), previously equilibrated with the above described buffer [Citation17]. The flow rate of the column was kept at 5.8 mL/min. The protein eluted from the column was monitored by measuring absorbance at 280 nm. The main fraction was collected, dialyzed against distilled water, and then lyophilized. The chromatographed β-vignin was dissolved in 50 mM Tris-HCl buffer, pH 7.5 and fractionated by ion exchange chromatography on Mono-Q column (5.0 cm × 0.5 cm). A NaCl concentration gradient from 0.05 to 0.5 M lasting forty minutes, with flow rate of 1 mL/min, was applied. To wash the column, 1.0 M NaCl solution containing 0.01% NaN3 was used.
Estimation of β-vignin apparent molecular weight
The apparent molecular mass of the 7S globulin from cowpea was estimated by Size Exclusion Chromatography (SEC) on a Sephadex G-200 column (50 cm × 2.5 cm), previously equilibrated in 10 mM potassium phosphate buffer, pH 7.5, and containing 0.5 M NaCl and eluted at a flow rate of 5.8 mL/tube at room temperature. Protein applied was at a concentration of 1 mg/mL. The elution of proteins was monitored by absorbance at 280 nm. The following standard proteins were used for column calibration (see Figure ): ferritin (440 kDa), myosin (240 kDa), galactosidase (116 kDa), BSA (66 kDa), ovalbumin (45 kDa) and cytochrome C (12.4 kDa) (Sigma-Aldrich®, Milan, Italy). Adzuki bean vicilin was included for comparison.
SDS–PAGE
One-dimensional gel electrophoresis in the presence of 2% 2-mercaptoethanol was performed on 12% polyacrylamide gels, using a Mini Protean II cell (Bio-Rad, Hercules, CA, USA) according to Laemmli [Citation21]. Marker proteins of known molecular weight were used (GE Healthcare®, Milan, Italy). Gels were stained with Coomassie Brilliant Blue (R-250), and analyzed by densitometry scanning with the Alpha Imager 6.0 software (Alpha Innotech®, San Leandro, CA, USA).
N-terminal amino acid sequencing
After SDS–PAGE, gels were transferred to PVDF membranes (Bio-Rad, Milan, Italy) by blotting on a Trans-blot Electrophoretic Transfer Cell (Bio-Rad, Milan, Italy), according to Matsudaira [Citation22]. Selected protein bands were excised and submitted to direct N-terminal amino acid sequencing. Automated Edman degradation was performed on a pulsed-liquid sequencer equipped with a PTH analyzer (Procise model 491 Applied Biosystems, Foster City, CA).
Detection of N-glycosylated polypeptides by ConA revelation
Proteins separated by SDS–PAGE were electrophoretically transferred to a nitrocellulose membrane, using a mini Trans-blot Transfer Cell (BioRad®). The blotted membranes were used to detect glycol-polypeptides by ConA/peroxidase method [Citation23].
Inductively coupled plasma/mass spectrometry (ICP-MS) analysis for globulin metal content
ICP-MS analysis (Bruker AURORA® M90 ICP-MS) was performed according to Mermet and Poussel [Citation24]. Briefly, the samples (about 0.2 g) were digested by a microwave digestor system (Anton Paar Multiwave-Eco) in Teflon tubes filled with 10 mL of 65% HNO3 by applying a two-step power ramp (Step 1: 200 W in 10 min, kept for 5 min; Step 2: 650 W in10 min, kept for 15 min). In the mineralized sample, the concentrations of Mg, Ca, Ni and Zn were measured. Typical analysis interferences were removed by using CRI (Collision-Reaction-Interface) with an H2 flow of 65 mL/min through the skimmer cone.
Immobilized metal affinity chromatography (IMAC)
Immobilized metal affinity chromatography separation of the cowpea total protein extract, as well as three other seed sources, namely adzuki bean, soybean and lupin for comparison, was carried out on a NiNTA-agarose (Qiagen®, Milan, Italy) column (1.8 cm × 5.0 cm). Total protein extracts (TPEs) were prepared by suspending 2 g of each flour in 50 mM Tris-HCl buffer, pH 7.5 in the presence of 0.5 M NaCl in the ratio 1:20 w/v. The slurries were stirred at room temperature for 30 min. After centrifugation at 10,000 g for 30 min, aliquots (2 mL) of the supernatants were applied to the NiNTA-agarose column equilibrated with 50 mM Tris–HCl buffer, pH 7.5. The resin was thoroughly washed with the same buffer to elute the unbound material. Elution of the bound material was carried out with the same buffer containing 0.1 M imidazole.
Results and discussion
In the present work, a convenient isolation procedure of cowpea main protein fraction, i.e. the β-vignin, is described (Figure ). The protocol did not make use of any chromatographic step, thus allowing easy upgrading to large-scale protein preparations. This procedure can be useful for protein massive uses, including nutritional and nutraceutical studies, which may require animal or human testing, or direct exploitation in targeted food formulations. Approximately 8 g of β-vignin were recovered from 100 g cowpea flour. The protein content of the freeze dried powder was 92% by the Kjeldahl method (N × 6.25). The isolated β-vignin exhibited a high degree of homogeneity, as assessed by SDS–PAGE (Figure ). The two major bands corresponded to the β-vignin major polypeptides of 60 and 56 kDa, typical of the vicilin family polypeptides [Citation9,10,25–27]. N-terminal amino acid sequencing of these bands gave the sequences IVHR and IVHRXHQESQEE respectively, thus confirming their identity (see Figure 1SM, supplementary material, for the protein sequence).
Figure 2. SDS–PAGE analysis under reducing conditions and densitometric scans with the band profiles of cowpea protein samples. TPE: total protein extracts; βV: enriched β-vignin; M: marker proteins; Con A: Blotted membranes reacted with ConA for N-glycosylated polypeptide detection.
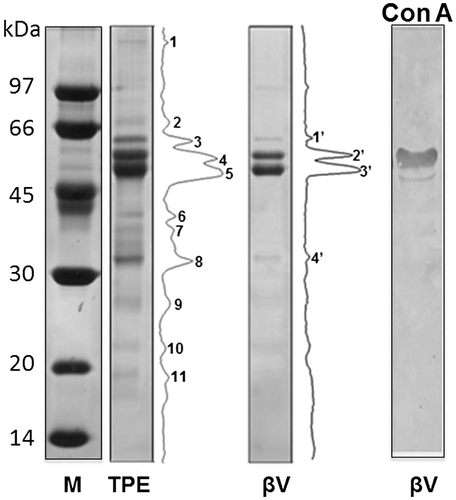
Densitometric analysis of cowpea total protein extract (TPE) evidenced 11 main peaks, whereas the β-vignin isolated according to the proposed protocol had only two major and two minor peaks (Figure ). The percentage of β-vignin main subunits in the TPE was approximately 60% of the total protein, while in the enriched β-vignin fraction the respective subunits summed up to 92% of total detected bands. The 11S globulin bands are barely visible in the TPE separation (bands at 30 and 20 kDa for the acidic and basic subunits, respectively), thus suggesting that the 11S globulin present in the cowpea used in this study was not a major component. This finding is in contrast with previous results by Freitas et al. [Citation8], who indicated an almost equivalent amount of 11S globulin to the 7S one. This may depend on the intrinsic variability of protein composition in seeds, which could be attributed to cultivar differences, as well as agronomic conditions, related to the sulphur availability, as previously suggested [Citation28].
In order to improve the protein homogeneity for further molecular characterization, size exclusion chromatography (SEC) and ion exchange chromatography (IEC) were performed, as described under Methods. The unique peak present in IEC confirmed the remarkable charge homogeneity of the β-vignin (Figure , panel b). Analytical SEC separation on Sephadex G200 showed a symmetric peak (Figure , panel a) that proved to have a native apparent molecular weight of 133,800 ± 12,200 (Figure 2SM). This finding indicates that the purified β-vignin conserved the oligomeric status of the vicilin family [Citation29]. Some large sized protein material likely represents a greater assembly of the vicilin-like subunits (Figure , panel a).
Figure 3. Chromatographic separation of enriched cowpea β-vignin. (a) SEC separation; (b) IEC separation (dotted line: NaCl concentration).
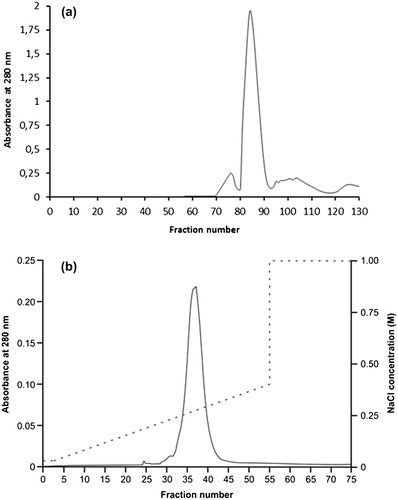
Next, we explored two never described molecular properties of β-vignin, i.e. the glycosylation pattern and the metal ion binding capacity. These properties may have relevant roles in protein structural stabilization, protein-protein interactions, antibody recognition, metal transport and other cellular events, such as signaling processes and defense mechanisms.
The presence of N-acetylglucosamine-linked oligosaccharides was estimated by reaction with the lectin concanavalin A (ConA). Figure shows the Western blotting of β-vignin polypeptides and the results of the reaction with ConA. Only one of the two main bands was significantly reactive. The faster migrating band did not react with ConA. These results allowed concluding that the two cowpea bands differ for their glycosylation status, being the larger polypeptide glycosylated and the smaller one non-glycosylated. This is likely one of the reasons of the molecular weight differences of the two polypeptide chains. As a matter of facts, the analysis of the unique available cowpea sequence (Uniprot A8YQH5_VIGUN) did not show (Figure 1SM, underlined residues) any N-glycosylation consensus motif (NXS/T). However, a recently identified cowpea gene expressed in seeds (NCBI-EST GH619103.1), with sequence highly similar to sequence A8YQH5_VIGUN, shows the glycosylation consensus motif NAT (Figure 1SM, residues in bold). In the same position, the sequence A8YQH5_VIGUN display the residues TAT (residues 442–444). This glycosylation site is conserved in other vicilin proteins such as those from adzuki, soybean and lupin. Therefore, the peculiar glycosylation pattern of β-vignin suggests the identification of a new gene product of cowpea β-vignin.
To check the metal interaction capacity of β-vignin, IMAC was performed on a NiNTA matrix. NiNTA-bound protein fractions of different legume species TPEs are compared by SDS–PAGE (Figure ). The electrophoretic profiles of cowpea sample fully matched those shown in Figure . Sequencing of the first four N-terminal amino acids (IVHR) confirmed the identity of the protein. Therefore, β-vignin was capable of binding metal, a property that has never been described before. A similar result was obtained with the 7S globulins of adzuki bean and soybean. Conversely, the bound material from lupin seed migrated as the γ-conglutin large and small subunits, which does not belong to the vicilin family [Citation30]. Computational prediction tools (available on-line at http://metaldetector.dsi.unifi.it and at http://raptorx.uchicago.edu) were unable to unequivocally identify the molecular determinants at the basis of the binding capacities of these 7S globulins (not shown).
Figure 4. SDSPAGE comparative analysis under reducing conditions of protein fractions bound to NiNTA matrix and eluted with imidazole from TPEs of cowpea (Cp), adzuki bean (Ad), soybean (Sb) and lupin (Lp).
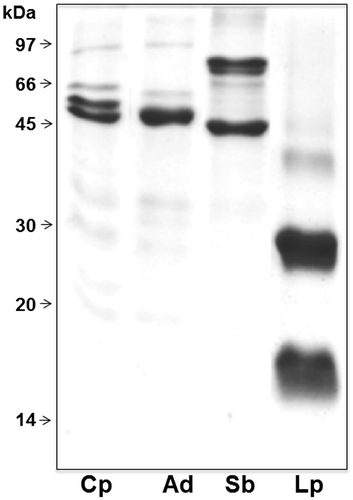
To further test the capacity of β-vignin to bind divalent metal ions, the content of calcium, zinc, magnesium and nickel was measured by ICP-MS. The results are reported in Table . The molar ratios of each metal for β-vignin monomer were 0.25, 0.09, 0.07 and 0.05, respectively. Only for 7S globulin adzuki beans was proved a metal ion-binding capacity, namely two calcium ions per subunit (PDB: 2EA7). Since the amount of calcium detected in our study for adzuki beans globulin was lower than that reported previously [Citation31], it cannot be excluded that the purification treatments have caused the partial loss of the bound calcium ions. This would indicate a quite weak ion binding capacity of the tested 7S globulins.
Table 1. Molar ratios of selected metal ions to cowpea, adzuki bean and lupin 7S globulins.Table Footnotea
Conclusions
The chromatography-independent fractionation procedure used in the present work allowed to obtain a protein preparation with a high degree of homogeneity, suitable for industrial applications. This can open the way for functional and nutritional investigations and tailored uses in value-added nutraceutical formulations.
The present data on the molecular features of β-vignin increase the set of information available for this protein. Our molecular characterisation allowed the identification of a glycosylated β-vignin version, never isolated before. The apparent M.W. of the native protein was consistent with the widely acknowledged trimeric structure of all vicilin-like proteins. Intriguingly, the trimer shows some metal binding capacity, as revealed by IMAC and IC-MS analyses, a property which is shared with only few other legume vicilins.
Author Contributions
AC, JC, MD, AS conceived and designed the experiments. AC, JC, ESF, FS, performed the experiments. AC, EMC, JC, AD, MD, ESF, CM, VAN, AS, analyzed the data. JC, MD, ESF, AS wrote the manuscript.
Disclosure statement
No potential conflict of interest was reported by the authors.
Supplemental data
Supplemental data for this article can be accessed 10.1080/09168451.2017.1419855.
Acknowledgments
We are indebted with Prof. Armando Negri for N-terminal amino acid sequencing and with Giorgio Lucchini for ICP-MS analyses.
References
- FAOSTAT. Available from: www.fao.org/faostat/
- Alonso BO, Rovir RF, Vegas CA, et al. Papel de las leguminosas en la alimentación actual. Actividad Dietética. 2010;14:72–76.10.1016/S1138-0322(10)70014-6
- Carbonaro M, Maselli P, Nucara A. Structural aspects of legume proteins and nutraceutical properties. Food Res Int. 2015;76:19–30.10.1016/j.foodres.2014.11.007
- Phillips RD, McWatters KH, Chinnan MS, et al. Utilization of cowpeas for human food. Field Crop Res. 2003;82:193–213.10.1016/S0378-4290(03)00038-8
- Maia FMM, Oliveira JTA, Matos MRT, et al. Proximate composition, amino acid content and haemagglutinating and trypsin-inhibiting activities of some Brazilian Vigna unguiculata (L) Walp cultivars. J Sci Food Agr. 2000;80:453–458.10.1002/(ISSN)1097-0010
- Grusak MA. Legumes. 2nd ed. In: Caballero B, Allen L, Prentice A, editors. Encyclopedia of human nutrition. New York (NY): Elsevier Academic Press; 2005. p. 120–126.10.1016/B0-12-226694-3/00191-X
- Murray DR, Mackenzie KF, Vairinhos F, et al. Electrophoretic studies of the seed proteins of cowpea, Vigna unguiculata (L.). Walp Z Pflanzenphysiol. 1983;109:363–370.10.1016/S0044-328X(83)80118-4
- Freitas RL, Teixeira AR, Ferreira RB. Characterization of the proteins from Vigna unguiculata seeds. J Agric Food Chem. 2004;52:1682–1687.10.1021/jf0300588
- Carbonaro M, Grant G, Cappelloni M. Heat-induced denaturation impairs digestibility of legume (Phaseolus vulgaris L. and Vicia faba L.) 7S and 11S globulins in the small intestine of rat. J Sci Food Agr. 2005;85:65–72.10.1002/(ISSN)1097-0010
- CARBONARO M. 7S globulins from Phaseolus vulgaris L.: impact of structural aspects on the nutritional quality. Biosci Biotechnol Biochem. 2006;70:2620–2626.10.1271/bbb.60203
- Duranti M, Lovati MR, Dani V, et al. The α′-subunit from soybean 7S globulin lowers plasma lipids and up-regulates liver VLDL receptors in rats fed a hypercholesterolemic diet. J Nutr. 2004;134:1334–1339.
- Consonni A, Lovati MR, Manzoni C, et al. Cloning, yeast expression, purification and biological activity of a truncated form of the soybean 7S globulin alpha’ subunit involved in Hep G2 cell cholesterol homeostasis. J Nutr Biochem. 2010;21:887–891.10.1016/j.jnutbio.2009.07.003
- Ferreira ES, Silva MA, Demonte A, et al. β-Conglycinin (7S) and glycinin (11S) exert a hypocholesterolemic effect comparable to that of fenofibrate in rats fed a high-cholesterol diet. J Funct Foods. 2010;2:275–283.10.1016/j.jff.2010.11.001
- Ferreira ES, Silva MA, Demonte A, et al. β-conglycinin combined with fenofibrate or rosuvastatin have exerted distinct hypocholesterolemic effects in rats. Lipids Health Dis. 2012;11:11–18.10.1186/1476-511X-11-11
- Ferreira ES, Amaral ALS, Demonte A, et al. Hypocholesterolaemic effect of rat-administered oral doses of the isolated 7S globulins from cowpeas and adzuki beans. J Nutr Sci. 2015;4(e7):1–9.
- Marques MR, Freitas RAMS, Carlos CC, et al. Peptides from cowpea present antioxidant activity, inhibit cholesterol synthesis and its solubilisation into micelles. Food Chem. 2015;168:288–293.10.1016/j.foodchem.2014.07.049
- Neves VA, Pereira DD, Shoshima AHR, et al. Características da solubilidade proteica e isolamento da globulina principal de caupí (Vigna unguiculata L. Walp.) cultivar BR-14 Mulato. Alim Nutr. 2003;14:47–55.
- Horax R, Hettiarachchy NS, Chen P, et al. Preparation and characterization of protein isolate from cowpea (Vigna unguiculata L. Walp.). J Food Sci. 2004;69:114–118.
- Zhang ML, Gao JL, Yang HX. Functional properties of 7s globulin extracted from cowpea vicilins. Cereal Chem. 2009;86:261–266.10.1094/CCHEM-86-3-0261
- Lowry OH, Rosebrough NJ, Farr AL. Protein measurement with the Folin phenol reagent. J Biol Chem. 1951;193:265–275.
- Laemmli UK. Cleavage of structural proteins during assembly of the head of bacteriophage T4. Nature. 1970;227:680–685.10.1038/227680a0
- Matsudaira P. Sequence of picomole quantities of proteins electroblotted onto polyvinylidene difluoride membranes. J Biol Chem. 1987;262:10035–10037.
- Faye L, Chrispeels MJ. Characterization of N-linked oligosaccharides by affinoblotting with concanavalin A-peroxidase and treatment of the blots with glycosidases. Anal Biochem. 1985;149:218–224.10.1016/0003-2697(85)90498-1
- Mermet JM, Poussel E. ICP emission spectrometers: analytical figures of merit. Appl Spectrosc. 1995;49:12A–18A.10.1366/0003702953965588
- Chen THH, Gusta LV, Tjahjadi C, et al. Electrophoretic characterization of adzuki bean (Vigna angularis) seed proteins. J Agr Food Chem. 1984;32:396–399.10.1021/jf00122a053
- Mendoza EMT, Adachi M, Bernardo AEN, et al. Mungbean [Vigna radiata (L.) Wilczek] globulins: purification and characterization. J Agr Food Chem. 2001;49:1552–1558.10.1021/jf001041 h
- Sathe SK, Venkatachalam M. Fractionation and biochemical characterization of moth bean (Vigna aconitifolia L.) proteins. Food Sci Technol. 2007;40:600–610.
- Weber H, Borisjuk L, Wobus U. Molecular physiology of legume seed development. Annu Rev Plant Biol. 2005;56:253–279.10.1146/annurev.arplant.56.032604.144201
- Deshpande SS, Damodaran S. Conformational characteristics of legume 7S globulins as revealed by circular dichroic, derivative U.V. absorption and fluorescence techniques. Int J Pept Protein Res. 1990;35:25–34.
- Scarafoni A, Ronchi A, Duranti M. γ-Conglutin, the Lupinus albus XEGIP-like protein, whose expression is elicited by chitosan, lacks of the typical inhibitory activity against GH12 endo-glucanases. Phytochem. 2010;71:141–148.
- Fukuda T, Maruyama N, Salleh MR, Mikami B, Utsumi S. Characterization and crystallography of recombinant 7S globulins of Adzuki bean and structure−function relationships with 7S globulins of various crops. J Agric Food Chem. 2008;56:4145–4153.10.1021/jf072667b