Abstract
Plants coordinate the timing of flower opening with pollen and gynoecium maturation to achieve successful pollination. However, little is known about how the coordination is executed. We found that flower bud development was paused immediately before flower opening in a jasmonic acid (JA)-insensitive tomato mutant, jai1-1. Phytohormone measurement and RNA analysis in flower buds revealed that newly synthesised JA peaked at two days before flower opening and the expression of a transcription factor gene SlMYB21 delayed in jai1-1. Buds of transgenic tomato plants expressing an artificial repressor, AtMYB24-SRDX, which was expected to impede the function of SlMYB21, aborted flower opening and resembled those of jai1-1. Furthermore, the AtMYB24-SRDX plants produced abnormal pollen grains deficient in germination and pistils that did not support pollen tube elongation. We concluded that JA facilitates the expression of SlMYB21, which coordinates flower opening, pollen maturation, and gynoecium function in tomato.
Jasmonic acid stimulates SlMYB21 expression, which is required for coordinated flower opening and fertility in male and female organs in tomato.
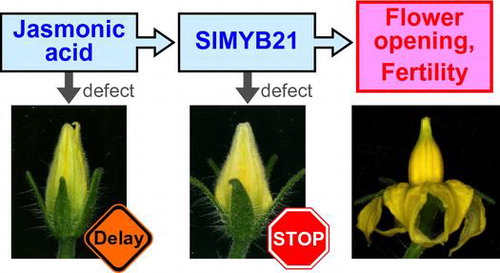
The flower is the most important reproductive organ in angiosperms. The flowers release mature pollen grains from anthers, attract pollinators by color and scent, attach the pollen grains to the pollinators, and accept pollen grains at stigmas. Therefore, coordination of flower opening with pollen maturation and gynoecium maturation is important for successful pollination and subsequent fertilization. Flower opening is regulated by both the internal environment such as pollen and ovule development and circadian rhythms and the external environment such as light, temperature, and humidity [Citation1,2]. Moreover, many phytohormones, such as auxin, gibberellin (GA), abscisic acid (ABA), ethylene, and jasmonic acid (JA), have been reported to be involved in the regulation of flower opening with relation to both internal and external cues [Citation1].
Involvement of JA in the regulation of flower opening was initially reported in the analysis of a JA-deficient mutant, defective in anther dehiscence1 (dad1), in Arabidopsis [Citation3]. The dad1 flower buds developed normally until the end of the middle stage, which corresponded to two days before flower opening in the wild type (WT). In the later stage, however, the development of the mutant flower buds was retarded in comparison to WT until flower opening, and as a consequence, more unopened buds were clustered in the inflorescence of dad1 [Citation3,4]. The dad1 flowers also showed delayed anther dehiscence and pollen grain infertility, and all three defects including delayed flower opening were rescued by exogenous application of JA [Citation3]. Similar phenotypes appeared in other JA biosynthesis mutants such as allene oxide synthase, oxophytodienoate reductase3 (opr3), double mutants lipoxygenase3 lipoxygenase4 [Citation5–8], and a JA-insensitive mutant, coronatine insensitive1 (coi1) [Citation9]. COI1 is a JA receptor essential for all the JA responses including defense and stress responses in Arabidopsis [Citation10]. COI1 is an F-box protein that forms a complex with a jasmonate ZIM-domain protein, and the complex specifically binds to jasmonoyl-L-isoleucine (JA-Ile). Hence, JA-Ile is thought to be an active form of JA [Citation11,12]. These observations indicate that JA is an activator of flower opening, anther dehiscence, and pollen development in Arabidopsis.
Regulation of flower opening by JA was also reported in other plants. In Brassica rapa, flower opening was suppressed in the transformants expressing an antisense gene of BrDAD1, which is the putative ortholog of Arabidopsis DAD1, and the phenotype was restored by application of JA [Citation13]. In wild tobacco, Nicotiana attenuata, a series of transgenic plants in which JA biosynthetic genes such as ALLENE OXIDE CYCLASE (AOC), or a JA perception gene, COI1, were silenced showed delayed flower opening or incomplete corolla expansion phenotypes [Citation14]. In rice, opening of florets was induced by treatment of methyl jasmonate, and the floret opening as well as anther dehiscence was impaired in the dysfunctional mutants of OsJAR1, which encodes an enzyme forming JA-Ile [Citation15,16]. Enhanced flower opening by methyl jasmonate treatment was also observed in Brassica napus [Citation17].
Along with the defects in flower opening, JA-related mutants often affect the development of floral organs. Rice mutant extra glume 1 (eg1), which is a mutation in a homolog of DAD1, changed floral organ identity and number [Citation18]. Tomato mutant jasmonic-acid insensitive1 (jai1), of which the causal gene is SlCOI1, is a unique ortholog of Arabidopsis COI1 and showed reduced pollen viability and female sterility [Citation19,20]. Dobritzsch et al. proposed that the reduced pollen fertility in jai1 is attributed to the premature tapetum degradation and premature anther dehiscence in the absence of normal JA signaling [Citation21]. These observations revealed that JA is not only a common regulator of flower opening in many plants but also involved in the development of floral organs including male and female gametophytes in a species-dependent manner. However, their mechanisms are largely unknown.
A transcriptome analysis comparing JA-treated and not-treated stamens of Arabidopsis opr3 mutants revealed that two R2R3-type MYB transcription factors, AtMYB21 and AtMYB24, were strongly induced by JA [Citation22]. T-DNA insertion mutants of atmyb21 exhibited delayed flower opening, shorter anther filaments, delayed anther dehiscence, and reduced male fertility, resembling the phenotype of JA-deficient mutants [Citation22]. Another transcriptome analysis identified three genes AtMYB21, AtMYB24, and AtMYB57 as GA-dependent genes in flower buds [Citation23]. All atmyb21 phenotypes were enhanced in atmyb21 atmyb24 double mutants and were further exacerbated in atmyb21 atmyb24 atmyb57 triple mutants, suggesting overlapping functions of these three R2R3-MYB genes [Citation22,24]. The authors concluded that GA acts through JA to control expression of the R2R3-MYB genes and promote stamen filament elongation [Citation23].
Involvement of putative orthologs of AtMYB21/24/57 in flower opening has also been reported in other plants. Transgenic torenia plants expressing the Pro35S:AtMYB24-SRDX gene, which consisted of the cauliflower mosaic virus 35S promoter (Pro35S) and the AtMYB24 coding sequence fused to the sequence of an EAR motif repression domain (SRDX), exhibited a deficiency in flower opening [Citation25]. The EAR motif was originally identified as a transcription repression domain of ETHYLENE-RESPONSIVE ELEMENT-BINDING FACTOR in Arabidopsis and has the ability to change transcription activators to repressors when it is fused to the activators [Citation26,27]. In petunia and Nicotiana attenuata, suppression of the EMISSION OF BENZENOIDS II (EOBII) gene, a close homolog of AtMYB21/24, by RNA interference caused a failure of flower opening [Citation28]. These findings indicate that the ortholog(s) of AtMYB21/24/57 regulate flower opening in diverse plant species. However, the relationship between JA signaling and regulation of AtMYB21/24/57 ortholog(s) has not yet been elucidated in plants other than Arabidopsis.
In this study, we found that tomato plants having jai1-1, a strong allele of jai1 mutation, showed delayed flower opening. Phytohormone measurement and transcript analysis revealed that JA stimulated the expression of the SlMYB21 gene, a unique ortholog of AtMYB21/24/57 in tomato. We showed that transgenic tomato expressing a chimeric transcription repressor for SlMYB21 exhibited a more exacerbate phenotype than jai1-1 particularly in male and female sterility. We will discuss the involvement of JA signaling and SlMYB21 in the regulation of flower opening and floral organ development in tomato.
Materials and methods
Plant materials and growth conditions
Tomato cultivar Micro-Tom (Solanum lycopersicum L. cv. Micro-Tom) was used unless otherwise indicated. jai1-1, a deletion allele of the SlCOI1 gene (Solyc05g052620) in a Micro-Tom background, was described previously [Citation19]. Due to the strong sterility of jai1-1 homozygotes, jai1-1 was maintained as a heterozygous line, and homozygous jai1-1 plants were identified by a PCR-based genotyping [Citation19]. We established a WT line having a homozygous unmutated SlCOI1 allele from the progeny of a jai1-1 heterozygote and named it “MT-GH.” MT-GH was used as the WT control in most experiments in this study. For RNA sequencing (RNA-seq), a line of Micro-Tom (MT-J, TOMJPF00001) obtained from the National Bio-Resource Project (http://tomato.nbrp.jp/indexEn.html) was used. Another cultivar of tomato (cv. Momotaro 8, Takii, Japan) was also used for some experiments. Seeds were sown on pre-fertilized soil (Nippi No. 1, Nippon Hiryo, Japan) and grown under fluorescent white light (170 μmol/s/m2) of 16 h light and 8 h darkness at 25 °C.
RNA preparation and gene expression analysis
A whole flower bud or the floral organs of a bud were harvested and immediately frozen in liquid nitrogen. Total RNA was extracted using an RNeasy Plant Mini Kit (QIAGEN, www.qiagen.com). Extracted RNA was reverse transcribed with ReverTra Ace qPCR RT Master Mix with gDNA Remover (Toyobo, www.toyobo-global.com). qRT-PCR was performed using the StepOne Real-Time PCR system (Thermo Fisher Scientific, www.thermofisher.com) and THUNDERBIRD SYBR qPCR Mix (Toyobo). RNA yields and reverse transcription efficiencies were normalized by the amount of DNAJ (a subset of tomato DnaJ/Hsp40 homologs comprising Solyc04g009770, Solyc05g055160, and Solyc11g006460) mRNA or SAND (Solyc03g115810) mRNA, as described in the literature [Citation29]. The primers used in qRT-PCR are listed in Supplemental Table S1.
RNA-seq analysis was carried out as described previously [Citation30]. Reference cDNA sequences were extracted from a whole genome sequence database (version SL2.40 of Solanum lycopersicum cv. Heinz 1706) by use of ITAG2.3 gene prediction information (Sol Genomics Network, solgenomics.net).
Measurement of phytohormones
Each flower bud was separately harvested from an inflorescence and immediately frozen in liquid nitrogen. Extraction and purification of phytohormones were performed as described previously [Citation31]. Quantification of phytohormone species excepting JA-Ile were extracted and measured as described previously [Citation31,32]. For JA-Ile quantification, stable isotope-labeled JA-Ile ([2H2]N-[(-)-Jasmonyl]-isoleucine, OlChemim, www.olchemim.cz/) was used as the internal standard. Detection was performed by ultra-high performance liquid chromatography (UHPLC)-electrospray interface (ESI) and quadrupole-orbitrap mass spectrometer (UHPLC/Q-Exactive™; Thermo Fisher Scientific) with an AQUITY UPLC HSS T3 column (2.1 × 100 mm; 1.8 μm, Waters).
Construction of plasmids and transgenic plants
To construct the plasmid pBCKK-Pro35S:AtMYB24:SRDX expressing the AtMYB24-SRDX gene under the control of the cauliflower mosaic virus 35S promoter, the Pro35S:MYB24-SRDX fragment was transferred from a plasmid 35S:MYB24-SRDX/pEntry, which is a previously reported entry plasmid constructed on p35SSRDXG vector [Citation33], to the pBCKK binary vector [Citation34] by a Gateway LR reaction (Thermo Fisher Scientific). Transformation of tomato plants was performed as described previously [Citation35].
Microscopy
An SZX12 stereo microscope (Olympus, www.olympus-global.com/en/) was used for observation of the morphologies of flower buds and flowers. To observe pollen tube growth in vivo, manually pollinated pistils were harvested 24 h after pollination, fixed in ethanol:acetic acid (3:1 v/v) for 24 h, and treated for 12 h with 5 N NaOH. After being rinsed three times in water, the pistils were stained in 0.1 % aniline blue dye solution in 0.1 M K-phosphate buffer (pH 11) for 1 to 4 h. The pistils were mounted on a slide with 80% (v/v) glycerol solution and observed by a fluorescence microscope BX60 (Olympus) under UV light excitation (Filter set WU, excitation 330–385 nm, emission > 420 nm). To visualize the pollen nuclei, pollen grains in dehisced anthers were suspended in 1 μg/ml 4′,6-diamidino-2-phenylindole (DAPI) solution in 1 × PBS (130 mM NaCl, 5.1 mM Na2HPO4, 1.6 mM KH2PO4) by brief vortexing. The pollen suspension was mounted on a slide and observed by fluorescence microscopy with UV excitation. To observe the female gametophytes, ovules were collected from newly opening flowers, fixed in ethanol:acetic acid (9:1 v/v) solution for 2 h, and cleared in a modified Hoyer’s solution (7.5 g gum arabic, 100 g chloral hydrate, 5 ml glycerol, 30 ml H2O). Specimens were observed by differential interphase contrast microscopy.
Results
Delayed flower opening in a JA-insensitive mutant
In our growth condition, most of the WT Micro-Tom plants produced nine flowers in the primary inflorescence. Figure (A) shows a typical primary inflorescence in which the first (a) and second (b) flowers have opened and the next three buds (c–e) have petals longer than the sepals (hereafter called the petal-emerged phase). The other four buds (f–i) did not show their petals (Figure (A)). We observed the daily developmental process of each flower bud in the inflorescence and found that one flower newly opened every day in basal to apical order, and once-opened flowers continued to open for two days before senescence. The duration of the petal-emerged phase (including the partially opened bud) was three days on average (Figure (D), (F)).
Figure 1. Delayed flower opening in JA-insensitive jai1-1 mutants.
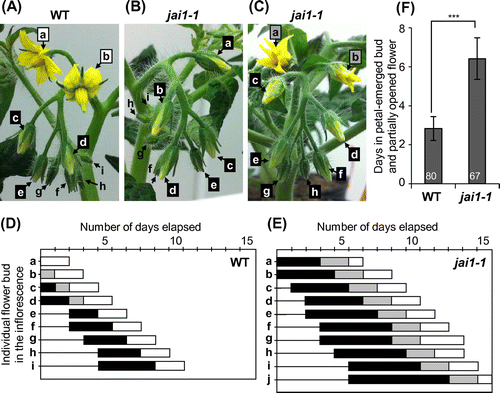
To analyze the roles of JA on the bud development and flower opening in tomato, we observed a JA-insensitive tomato mutant, jai1-1, which is a deletion allele of jai1 lacking a whole exon 2 and exon 3 of the SlCOI1 gene [Citation19,20]. Figure (B) shows a primary inflorescence of jai1-1 at the same developmental stage as that of the WT inflorescence shown in Figure (A). The size and shape of the four youngest flower buds (f–i) and the next three petal-emerged buds (c–e) of jai1-1 were similar to those of the corresponding buds in WT. However, the two largest buds (a and b) in jai1-1 had not yet opened, whereas the counterparts in WT had fully opened (Figure (A), B). Figure (C) shows another inflorescence of jai1-1 when the first two flowers (a and b) were partly (but not yet fully) opened. All the other buds showed the petals. Continuous observation of the inflorescence revealed that most of the jai1-1 flower buds required four to five days for passing though the petal-emerged phase and an additional two days for the partially opened phase before complete opening (Figure (E), (F)). These results indicated that the jai1-1 flower buds paused the development for three days in comparison to WT before opening. Hence, we concluded that JA has an important role in facilitating flower opening in tomato.
Levels of phytohormones in developing WT and jai1-1 flower buds
The above observations prompted us to examine whether JA and other phytohormones are biosynthesized and accumulated in tomato flower buds prior to flower opening and whether the levels might be different between in WT and jai1-1 flower buds. To describe the flower development of Micro-Tom, we used the bud age in days. We defined the age of the flower bud that attained 4 mm in length as a “day-1 bud” and larger flower buds on the following days as a “day-X bud” (Figure (A)). Typically, the day-2 bud became longer than 5 mm but its calyx was still closed. On day 3, the calyx opened and petals could be observed. On day 4, petals became longer than the sepals. On day 5, petals enlarged, whereas petal color remained pale green. On day 6, petal color changed to light yellow. On day 7, petals began to elongate outward, which meant initiation of flower opening. On day 8, petals elongated completely and bent outward. The flower fully opened, and this morphology continued to the end of day 9, except petals partially closed during the night. Then, flower began to close on day 10 and was senesced on day 11. The size and morphology of jai1-1 flower buds were equivalent to those in WT until day 5, but the development was delayed thereafter (Figure (B)). The day-6 bud of jai1-1 was indistinguishable from that on day 5. Petals began to turn yellow on day 7, but they were still tinged with green and never opened on day 8. The bud finally began to open on day 9 and was fully open on day 10. Petals closed on day 12 (Figure (B)). Consistent with the observation shown in Figure , bud development in jai1-1 was retarded for two to three days at the petal-emerged phase, and subsequent flower opening and closing proceeded two days later than in WT.
Figure 2. Development of WT and jai1-1 flower buds toward opening and changes of phytohormone levels in the flower buds. The levels of nine phytohormones in the same bud sample were simultaneously measured.
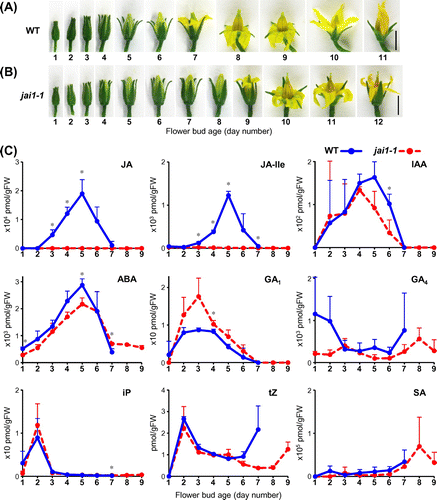
We performed simultaneous measurement of phytohormones from the day-1 bud to flower opening. Three flower buds were harvested for each stage (day 1 to day 7 for WT and day 1 to day 9 for jai1-1), and an individual bud was separately used for hormone extraction and measurement. It is an important advantage of this method that the levels of various hormones in the same samples can be compared directly. First, we analyzed JA and its biologically active form, JA-Ile, in WT. Both molecules began to increase on day 3, reached a sharp peak on day 5, at which petal growth was nearly completed but the color had not yet turned yellow, and decreased to the basal level when the flower began opening on day 7 (Figure (A), (C)). In contrast, accumulation of JA and JA-Ile in jai1-1 was hardly detectable throughout flower bud development (Figure (B), (C)), which confirmed a previously reported result [Citation21]. These results suggested that the JA and JA-Ile accumulated in the day-5 buds is important for facilitating the subsequent flower opening.
Similar peak formation around day 5 was observed for the levels of indole-3-acetic acid (IAA) and abscisic acid (ABA), whereas gibberellin A1 (GA1) reached the peak slightly earlier at day 3 (Figure (C)). However, there were only small differences between WT and jai1-1. Two cytokinins, N6-(Δ2-isopentenyl) adenine (iP), and trans-zeatin (tZ), temporary increased in the day-2 flower buds of both WT and jai1-1 (Figure (C)). Intriguingly, tZ increased in the day-7 bud in WT and day-9 bud in jai1-1, both of which corresponded to opening flowers. Although the difference on day 7 was not statistically significant due to large deviations in WT, all three measured values for WT were larger than those for jai1-1. Hence, it indicated that the level of tZ might be causally related to flower opening.
Salicylic acid (SA) increased gradually during bud development but not differentially between WT and jai1-1, suggesting no relation to flower opening (Figure (C)). GA4 was possibly accumulated in young flower buds and the opening flower in WT, though the reliability of this finding is low due to large deviations among samples (Figure (C)). In summary, simultaneous phytohormone measurement revealed that the levels of JA, JA-Ile, and tZ were affected by jai1-1 mutation during flower bud development. In the following experiments, we focused on the expression of biosynthesis and downstream genes of JA.
Expression of JA-related genes during flower bud development in WT and jai1-1
Among genes encoding JA biosynthetic enzymes, putative orthologs of Arabidopsis AOC (hereafter AtAOC) and OPR3 (AtOPR3) have been identified in tomato [Citation36,37], and we refer to them as SlAOC (Solyc02g085730) and SlOPR3 (Solyc07g007870), respectively. The expression levels of SlAOC and SlOPR3 were measured by quantitative reverse transcription PCR (qRT-PCR). In WT, the SlAOC expression was low until day 2, rapidly increased to the highest level at day 3, and gradually decreased by flower opening at day 7 (Figure (A)). SlOPR3 was moderately expressed throughout development with a broad peak around day 4 (Figure (B)). It was characteristic that these peaks slightly preceded (by one to two days) the accumulation of JA and JA-Ile. In contrast, the level of SlAOC expression in jai1-1 was kept low throughout bud development and was significantly lower than that in WT during day 1 to day 6 (Figure (A)). SlOPR3 was expressed in the mutant at a level comparable to WT, but the peak was unclear (Figure (B)). The reduced expression of JA biosynthetic genes, especially of SlAOC, was consistent with the deficient accumulation of JA and JA-Ile in the mutant.
Figure 3. Changes of gene expression in WT and jai1-1 flower buds toward opening.
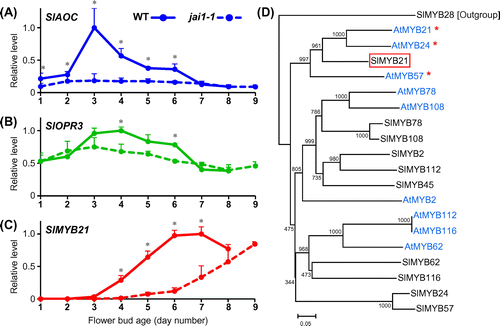
The weak correlation between SlOPR3 expression and JA accumulation prompted us to examine whether AtOPR3 homologs other than SlOPR3 in the tomato genome contribute to JA synthesis in developing flower buds. A BLAST search revealed six genes as being similar to SlOPR3 in the tomato genome (Supplemental Figure S1A) but no genes homologous to SlAOC. We analyzed an RNA-seq data-set consisting of a series of developing WT flower buds at days 1, 2, 3, 5, 6, and 7 and found that SlOPR3 as well as SlAOC was highly expressed in unopened flower buds with a peak at day 3, whereas the expression levels of most other SlOPR3 homologs in tomato, excepting Solyc10g086220, were much lower than that of SlOPR3 (Supplemental Figure S1, Supplemental Table S2). Solyc10g086220 showed moderate expression throughout bud development, but it has been shown that this gene, designated LeOPR1 or SlOPR1, is not involved in JA synthesis [Citation38]. Therefore, we concluded that SlOPR3 is predominantly involved in JA synthesis in developing flower buds and the contribution of other SlOPR3 homologs is small, if any.
In Arabidopsis, three R2R3-MYB genes, AtMYB21, AtMYB24, and AtMYB57, regulate flower opening and stamen elongation downstream of the JA signaling pathway [Citation22,23,39]. To identify the orthologs of these genes in tomato, we performed a similarity analysis of a subset of tomato and Arabidopsis R2R3-MYB genes [Citation40]. We found that only SlMYB21 (Solyc02g067760) belonged to the same clade as AtMYB21/24/57, suggesting that SlMYB21 is a unique ortholog of AtMYB21/24/57 (Figure (D)). The expression of SlMYB21 was first detected in the day-3 bud, in which JA and JA-Ile accumulation began, and reached the maximum level on day 6, which was one day later than the peaks of JA and JA-Ile accumulation (Figures (A), (C), (C)). The expression profile was consistent with the data of RNA-seq analysis (Supplemental Figure S1D). The RNA-seq analysis also revealed that the expression level of SlMYB21 in developing flower buds was much higher than those of the other nine genes shown in Figure (D) (Supplemental Figure S1D, Supplemental Table S2). In jai1-1 buds, the first detection of SlMYB21 expression was delayed to day 5, which was two days later than the timing in WT (Figure (C)). The expression increased gradually and finally reached the maximum level on day 9, which was comparable to the WT level at day 6 (Figure (C)). The results suggested that the expression of SlMYB21 does not require, but is facilitated by, activated JA signaling in flower buds. The delayed flower opening in jai1-1 was coincident with the delayed expression of the SlMYB21 gene.
Suppression of SlMYB21 mimics the delayed flower opening phenotype of jai1-1
In order to test whether SlMYB21 is involved in flower opening in tomato, we constructed transformants of Micro-Tom containing the Pro35S:AtMYB24-SRDX gene, which expressed a chimeric repressor protein, AtMYB24-SRDX, under the control of the cauliflower mosaic virus 35S promoter [Citation33]. Because AtMYB24 is one of the closest homologs of SlMYB21 (Figure (F)), the expression of Pro35S:AtMYB24-SRDX is expected to repress the transcription of SlMYB21 target genes in tomato. Among independently obtained 12 transformants redifferentiated from transgenic calli, six plants showed a remarkable phenotype. Flower buds of the transformants showed severely retarded opening or never opened until senescence (Figure (A), (B)). This phenotype resembles those of transgenic torenia plants containing the same gene [Citation25] as well as of Arabidopsis atmyb21 atmyb24 double mutants of strong alleles and atmyb21 atmyb24 atmyb57 triple mutants [Citation22,23]. Taken together with the uniqueness of SlMYB21 in the clade of AtMYB21/24/57 (Figure (D)), we concluded that AtMYB24-SRDX acts as a repressor of SlMYB21 in tomato.
Figure 4. Bud and flower development in AtMYB24-SRDX plants.
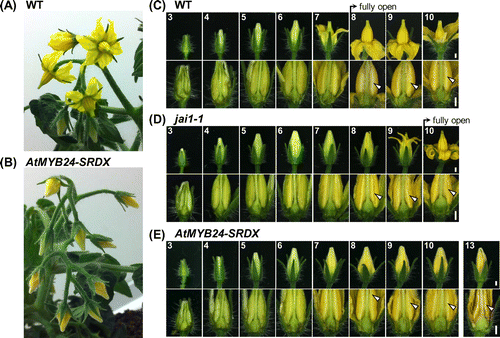
We compared the bud and flower development of the AtMYB24-SRDX transformants to that of WT and jai1-1 in the period from day 3 to day 10 (Figure (C)–(E)). Until day 5, the bud morphologies of all three lines, in particular the size and color of organs, were indistinguishable. The first difference was observed at day 6, when the WT petals began to turn yellow. Similar to jai1-1, the color of AtMYB24-SRDX petals remained pale green at this stage (Figure (C)–(E)). Flower buds of AtMYB24-SRDX plants were severely delayed in flower opening, and in extreme cases they never opened even when petals began to senesce on day 13 (Figure (E)). Nevertheless, observation of the inner organs revealed that the timings of anther color change (on the day 7) and anther dehiscence (on the day 8) were not delayed in either jai1-1 or AtMYB24-SRDX plants (Figure (C)–(E)). These observations indicated that the SlMYB21 gene regulates the timing of flower opening but not of anther dehiscence in tomato.
Deficient male and female fertility in AtMYB24-SRDX flowers
Flowers of AtMYB24-SRDX plants occasionally bore fruits but they contained few seeds (Figure (A)), suggesting that SlMYB21 plays an important role in male and/or female organs. A similar phenotype is observed in jai1-1 (Figure (A)), and the sterility has been attributed to the deficiency in male and female organs, though the detailed mechanism has not been elucidated [Citation19,20]. qRT-PCR analysis revealed that the SlMYB21 gene was expressed in all floral organs of Micro-Tom flower buds immediately before flower opening (day 6), and the levels in petals, anthers, filaments, and styles were more than two times higher than that in sepals (Figure (B)). Thus, we attempted to examine how SlMYB21 contributes to the fertility. We used transgenic AtMYB24-SRDX plants generated in the background of commercial cultivar Momotaro 8, of which the large organ size made dissection easier. Two out of 17 independent transformants of this cultivar showed the obvious opening-deficient phenotype in flowers (Supplemental Figure S2) and were used for further analysis.
Figure 5. Abnormal male and female fertility in AtMYB24-SRDX flowers.
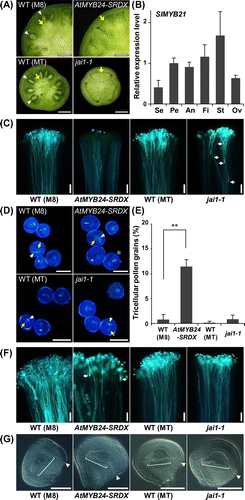
We manually pollinated AtMYB24-SRDX pollen grains onto the WT stigma and tested pollen tube elongation by aniline blue staining. In contrast to the control experiment, in which the WT pollen elongated many pollen tubes, a few pollen tubes were observed in a style when AtMYB24-SRDX pollen was pollinated (Figure (C)). The result that only a small number of pollen grains remained on the stigma indicated that most of the pollinated pollen grains dropped out during the process of aniline blue staining, presumably because they did not anchor to the stigma by their germinated pollen tubes. Defective pollen tube elongation was also observed for jai1-1 pollen grains. In this case, many pollen grains germinated but aborted their tube growth in the styles (Figure (C)). A morphological abnormality was also found in AtMYB24-SRDX pollen grains. Fully developed pollen grains in tomato contain two nuclei, a large vegetative nucleus and a small or elongated generative cell nucleus. But DAPI staining of pollen grains collected from dehisced anthers revealed that the generative nuclei in a considerable proportion (more than 10%) of AtMYB24-SRDX pollen grains had already divided into two putative sperm cell nuclei (Figure (D), (E)). Such generative cell division in ungerminated pollen grains was rarely observed in WT and jai1-1 pollen grains (Figure (D), (E)), even though the flowers had senesced. Therefore, we concluded that SlMYB21 is required for the development and function of pollen grains.
To evaluate the female fertility of AtMYB24-SRDX flowers, we pollinated WT pollen grains onto the AtMYB24-SRDX stigma and observed the pollen tube elongation. Most pollen grains germinated, but the pollen tubes frequently terminated their growth in the stigma or in the style, suggesting that SlMYB21 is required for stigmas and styles to facilitate pollen tube elongation (Figure (F)). Pistils of jai1-1 as well as WT did not show such defects. The failure of pollen tube elongation might be attributed to the developmental defects of ovules. However, we could not find any abnormalities among the ovules of AtMYB24-SRDX, jai1-1, and WT plants. In conclusion, SlMYB21 is necessary for the functional development of both male and female organs.
Discussion
In our growth conditions, Micro-Tom plants open a new flower every day in their primary inflorescences. This means that an inflorescence meristem of a primary shoot produces one flower bud every day, so that the inflorescence consists of a series of flower buds the ages of which differ by one day from the neighbors. We found that jai1-1 flower buds develop normally until day 5 of bud age, but subsequently delay the growth and require two to three extra days for opening. This phenotype resembles those of Arabidopsis JA-deficient and JA-insensitive mutants [Citation3–9]. In WT plants, JA and JA-Ile peak on day 5. After day 5, the developmental delay of jai1-1 buds appears, suggesting that activation of JA signaling is required to trigger the process of flower opening. The accumulated JA and JA-Ile levels in WT flower buds were higher than those in wounded tomato leaves [Citation41–43], suggesting that strong JA signaling is required for the flower opening. An RNA measurement showed that the level of SlAOC mRNA was temporally increased in the day-3 buds, which preceded the peak of JA accumulation by two days. The induction of SlAOC is dependent on the function of SlCOI1, the causal gene of jai1-1, which accounts for the failure of JA accumulation in jai1-1 buds. Another JA biosynthetic gene, SlOPR3, was moderately expressed throughout bud development but was also weakly upregulated in an SlCOI1-dependent manner. Hence, it seems likely that the activation of a positive feedback loop of JA synthesis is necessary for the promotion of flower opening. Activated JA signaling accelerates the expression of SlMYB21, the expression of which coincides with flower opening. Furthermore, strong repression of SlMYB21 caused a failure of flower opening in tomato, as reported in other plants such as torenia and petunia. These indicate that JA facilitates flower opening through the activation of SlMYB21 expression.
With respect to the development of stamens in jai1-1 flower buds, anther color change and anther dehiscence normally occurred, whereas petal elongation was delayed. This differential development in jai1-1 was previously explained as premature anther dehiscence and premature pollen release [Citation21]. However, based on our morphology and gene expression analyses, we proposed that anther wall development was normal but petal expansion was delayed in jai1-1.
Simultaneous phytohormone measurement revealed that GA1 peaked at day 3 in parallel with the expression of SlAOC. In Arabidopsis, GA is required for the induction of JA biosynthetic genes such as DAD1, and JA controls the expression of AtMYB21/24/57 genes and promotes stamen filament elongation [Citation23]. Auxin was also accumulated simultaneously with or slightly earlier than JA in the day-4 to day-5 flower buds. Arabidopsis AUXIN RESPONSE FACTOR6 (ARF6) and ARF8 are required for the activation of DAD1 expression [Citation4], and also AtMYB21/24 expression, by both JA-dependent and independent pathways [Citation39]. Our results suggested the existence of a similar mechanism in tomato. We also found a temporal accumulation of cytokinins and abscisic acid during flower bud development. In particular, an increased tZ level in parallel with flower opening is characteristic, though their biological meanings have not yet been explained.
Observation of AtMYB24-SRDX flowers revealed that SlMYB21 is not only required for flower opening but also plays important roles in male and female organ development. However, the phenotypic characteristics of AtMYB24-SRDX tomato were not identical to those of atmyb21 atmyb24 double mutants in Arabidopsis. In addition to the lack of petal expansion leading to unopened flowers, the atmyb21 atmyb24 double mutants with a combination of knockout alleles showed short stamen filaments, undehisced anthers, and reduced pollen fertility, but female fertility was normal [Citation22,39]. In contrast, the AtMYB24-SRDX tomato showed abnormal pollen development, impaired pollen tube elongation, and deficient stigma/style function, but anther dehiscence was normal. The effect on filament elongation cannot be evaluated due to innate short filaments in tomato. It is suggested that the functions of SlMYB21 and AtMYB21/24 have varied in the course of morphological diversification between tomato and Arabidopsis. This difference partly explains the phenotypic difference between Arabidopsis coi1 and tomato jai1. The former shows a failure of filament elongation and anther dehiscence, which results in the production of dysfunctional pollen grains [Citation9]. In contrast, the latter shows no defect in anther dehiscence but shows abnormal male and female fertility [Citation19,20]. We frequently found aborted pollen tube elongation of jai1-1 pollen grains, which is consistent with their reduced viability reported previously [Citation19]; however, we have not found any reason for female sterility.
We also found that there were considerable differences between jai1-1 mutants and AtMYB24-SRDX plants in the phenotypes of male and female organs. Pollen grains of AtMYB24-SRDX plants hardly germinated on the stigma, whereas jai1-1 pollen grains germinated but aborted pollen tube elongation. Precocious division of generative cells in pollen grains frequently observed in AtMYB24-SRDX plants occurs only rarely in jai1-1 pollen grains. Furthermore, styles of AtMYB24-SRDX plants inhibit pollen tube elongation, whereas jai1-1 styles do not show such a defect. These observations indicate that the SlMYB21 gene is required for the functional development of both male and female organs after the opening of flowers. Relatively weak defects in jai1-1 mutants in comparison to AtMYB24-SRDX plants might be explained by retarded but increased expression of SlMYB21 genes in jai1-1 flowers.
In summary, JA synthesised in developing flower buds accelerates the expression of the SlMYB21 gene, which coordinately induces petal elongation and functional development of male and female organs in tomato. JA might determine the timing of flower opening by integrating the signals of other hormones such as GA and auxin. Although the role of JA in inducing the expression of AtMYB21/24 or SlMYB21 genes is common between Arabidopsis and tomato, the functions of these MYB genes have diverged between the two species.
Accession number
FASTQ files of the RNA-seq reads are available with the accession number DRA006324.
Author contributions
T.N., T.S., T.H., H.S., and S.I. designed and coordinated the experiments. T.N., T.S., Y.T., and R.I. carried out the experiments and analyzed the results. T.N. and S.I. wrote the manuscript. All the authors have read and approved the final manuscript.
Disclosure statement
No potential conflict of interest was reported by the authors.
Funding
This work was supported by the Cabinet Office, Government of Japan, under the SIP Program “Technologies for creating next-generation agriculture, forestry and fisheries” (funding agency: Bio-oriented Technology Research Advancement Institution, NARO, Japan) to S.I., H.S. and T.S.; Japan Science and Technology Agency, under the ERATO Higashiyama Live-Holonics Project [grant number JPMJER1004] to T.H. and T.S.
Supplemental data
Supplemental data for this article can be accessed https://doi.org/10.1080/09168451.2017.1422107
SuppleTable_S2.xlsx
Download MS Excel (1.8 MB)SuppleTable_S1.pdf
Download PDF (7.6 KB)SupplementalFigures.pdf
Download PDF (477.6 KB)Acknowledgements
We thank Ms Tomomi Shinagawa and Mr Naoki Takahashi (Nagoya University) for her/his skilled technical assistance, Dr Shunsuke Imanishi (National Agriculture and Food Research Organization) and Professor Mikio Nakazono (Nagoya University) for helpful discussion, Professor Kenji Matsui (Yamaguchi University) for technical advice, Professor Gregg A. Howe (Michigan State University) for providing the jai1-1 seeds, and Dr Nobutaka Mitsuda (National Institute of Advanced Industrial Science and Technology) for providing 35S:MYB24-SRDX/pEntry and pBCKK plasmids. Micro-Tom seeds (TOMJPF00001) were provided by University of Tsukuba, Gene Research Center, through the National Bio-Resource Project of the AMED, Japan. Homology alignment was performed with Clustal W provided at the DDBJ website.
References
- van Doorn WG, Kamdee C. Flower opening and closure: an update. J Exp Bot. 2014;65:5749–5757.10.1093/jxb/eru327
- van Doorn WG, van Meeteren U. Flower opening and closure: a review. J Exp Bot. 2003;54:1801–1812.10.1093/jxb/erg213
- Ishiguro S, Kawai-Oda A, Ueda J, et al. The DEFECTIVE IN ANTHER DEHISCIENCE1 gene encodes a novel phospholipase A1 catalyzing the initial step of jasmonic acid biosynthesis, which synchronizes pollen maturation, anther dehiscence, and flower opening in Arabidopsis. Plant Cell. 2001;13:2191–2209.10.1105/tpc.13.10.2191
- Tabata R, Ikezaki M, Fujibe T, et al. Arabidopsis AUXIN RESPONSE FACTOR6 and 8 regulate jasmonic acid biosynthesis and floral organ development via repression of class 1 KNOX genes. Plant Cell Physiol. 2010;51:164–175.10.1093/pcp/pcp176
- Park JH, Halitschke R, Kim HB, et al. A knock-out mutation in allene oxide synthase results in male sterility and defective wound signal transduction in Arabidopsis due to a block in jasmonic acid biosynthesis. Plant J. 2002;31:1–12.10.1046/j.1365-313X.2002.01328.x
- Sanders PM, Lee PY, Biesgen C, et al. The Arabidopsis DELAYED DEHISCENCE1 gene encodes an enzyme in the jasmonic acid synthesis pathway. Plant Cell. 2000;12:1041–1061.10.1105/tpc.12.7.1041
- Stintzi A, Browse J. The Arabidopsis male-sterile mutant, opr3, lacks the 12-oxophytodienoic acid reductase required for jasmonate synthesis. Proc Natl Acad Sci. 2000;97:10625–10630.10.1073/pnas.190264497
- Caldelari D, Wang GG, Farmer EE, et al. Arabidopsis lox3 lox4 double mutants are male sterile and defective in global proliferative arrest. Plant Mol Biol. 2011;75:25–33.10.1007/s11103-010-9701-9
- Feys B, Benedetti CE, Penfold CN, et al. Arabidopsis mutants selected for resistance to the phytotoxin coronatine are male sterile, insensitive to methyl jasmonate, and resistant to a bacterial pathogen. Plant Cell. 1994;6:751–759.10.1105/tpc.6.5.751
- Xie DX, Feys BF, James S, et al. COI1: An Arabidopsis gene required for jasmonate-regulated defense and fertility. Science. 1998;280:1091–1094.10.1126/science.280.5366.1091
- Sheard LB, Tan X, Mao HB, et al. Jasmonate perception by inositol-phosphate-potentiated COI1-JAZ co-receptor. Nature. 2010;468:400–405.10.1038/nature09430
- Yan J, Zhang C, Gu M, et al. The Arabidopsis CORONATINE INSENSITIVE1 protein is a jasmonate receptor. Plant Cell. 2009;21:2220–2236.10.1105/tpc.109.065730
- Hatakeyama K, Ishiguro S, Okada K, et al. Antisense inhibition of a nuclear gene, BrDAD1, in Brassica causes male sterility that is restorable with jasmonic acid treatment. Mol Breed. 2003;11:325–336.10.1023/A:1023429700668
- Stitz M, Hartl M, Baldwin IT, et al. Jasmonoyl-L-isoleucine coordinates metabolic networks required for anthesis and floral attractant emission in wild tobacco (Nicotiana attenuata). Plant Cell. 2014;26:3964–3983.10.1105/tpc.114.128165
- Xiao YG, Chen Y, Charnikhova T, et al. OsJAR1 is required for JA-regulated floret opening and anther dehiscence in rice. Plant Mol Biol. 2014;86:19–33.10.1007/s11103-014-0212-y
- Zeng XC, Zhou X, Zhang W, et al. Opening of rice floret in rapid response to methyl jasmonate. J Plant Growth Regul. 1999;18:153–158.10.1007/PL00007063
- Pak HS, Guo Y, Chen MX, et al. The effect of exogenous methyl jasmonate on the flowering time, floral organ morphology, and transcript levels of a group of genes implicated in the development of oilseed rape flowers (Brassica napus L.). Planta. 2009;231:79–91.10.1007/s00425-009-1029-9
- Cai Q, Yuan Z, Chen M, et al. Jasmonic acid regulates spikelet development in rice. Nat Commun. 2014;5:3476.
- Li L, Zhao Y, McCaig BC, et al. The tomato homolog of CORONATINE-INSENSITIVE1 is required for the maternal control of seed maturation, jasmonate-signaled defense responses, and glandular trichome development. Plant Cell. 2004;16:126–143.10.1105/tpc.017954
- Li L, Li CY, Howe GA. Genetic analysis of wound signaling in tomato. Evidence for a dual role of jasmonic acid in defense and female fertility. Plant Physiol. 2001;127:1414–1417.10.1104/pp.010705
- Dobritzsch S, Weyhe M, Schubert R, et al. Dissection of jasmonate functions in tomato stamen development by transcriptome and metabolome analyses. BMC Biol. 2015;13:28.10.1186/s12915-015-0135-3
- Mandaokar A, Thines B, Shin B, et al. Transcriptional regulators of stamen development in Arabidopsis identified by transcriptional profiling. Plant J. 2006;46:984–1008.10.1111/tpj.2006.46.issue-6
- Cheng H, Song SS, Xiao LT, et al. Gibberellin acts through jasmonate to control the expression of MYB21, MYB24, and MYB57 to promote stamen filament growth in Arabidopsis. PLoS Genet. 2009;5:e1000440.10.1371/journal.pgen.1000440
- Chen X, Huang H, Qi TC, et al. New perspective of the bHLH-MYB complex in jasmonate-regulated plant fertility in arabidopsis. Plant Signal Behav. 2016;11:e1135280.10.1080/15592324.2015.1135280
- Sasaki K, Yamaguchi H, Narumi T, et al. Utilization of a floral organ-expressing AP1 promoter for generation of new floral traits in Torenia fournieri Lind. Plant Biotechnol. 2011;28:181–188.10.5511/plantbiotechnology.11.0124b
- Hiratsu K, Matsui K, Koyama T, et al. Dominant repression of target genes by chimeric repressors that include the EAR motif, a repression domain, in Arabidopsis. Plant J. 2003;34:733–739.10.1046/j.1365-313X.2003.01759.x
- Mitsuda N, Matsui K, Ikeda M, et al. CRES-T, an effective gene silencing system utilizing chimeric repressors. Methods Mol Biol. 2011;754:87–105.10.1007/978-1-61779-154-3
- Colquhoun TA, Schwieterman ML, Wedde AE, et al. EOBII controls flower opening by functioning as a general transcriptomic switch. Plant Physiol. 2011;156:974–984.10.1104/pp.111.176248
- Expósito-Rodríguez M, Borges AA, Borges-Pérez A, et al. Selection of internal control genes for quantitative real-time RT-PCR studies during tomato development process. BMC Plant Biol. 2008;8:131.10.1186/1471-2229-8-131
- Notaguchi M, Higashiyama T, Suzuki T. Identification of mRNAs that move over long distances using an RNA-Seq analysis of Arabidopsis/Nicotiana benthamiana heterografts. Plant Cell Physiol. 2015;56:311–321.10.1093/pcp/pcu210
- Kojima M, Kamada-Nobusada T, Komatsu H, et al. Highly sensitive and high-throughput analysis of plant hormones using MS-probe modification and liquid chromatography-tandem mass spectrometry: an application for hormone profiling in Oryza sativa. Plant Cell Physiol. 2009;50:1201–1214.10.1093/pcp/pcp057
- Ikeuchi M, Iwase A, Rymen B, et al. Wounding triggers callus formation via dynamic hormonal and transcriptional changes. Plant Physiol. 2017;175:1158–1174.10.1104/pp.17.01035
- Shikata M, Narumi T, Yamaguchi H, et al. Efficient production of novel floral traits in torenia by collective transformation with chimeric repressors of Arabidopsis transcription factors. Plant Biotechnol. 2011;28:189–199.10.5511/plantbiotechnology.10.1216a
- Fujita Y, Fujita M, Satoh R, et al. AREB1 is a transcription activator of novel ABRE-dependent ABA signaling that enhances drought stress tolerance in Arabidopsis. Plant Cell. 2005;17:3470–3488.10.1105/tpc.105.035659
- Sun HJ, Uchii S, Watanabe S, et al. A highly efficient transformation protocol for Micro-Tom, a model cultivar for tomato functional genomics. Plant Cell Physiol. 2006;47:426–431.10.1093/pcp/pci251
- Scalschi L, Sanmartín M, Camañes G, et al. Silencing of OPR3 in tomato reveals the role of OPDA in callose deposition during the activation of defense responses against Botrytis cinerea. Plant J. 2015;81:304–315.10.1111/tpj.2015.81.issue-2
- Ziegler J, Stenzel I, Hause B, et al. Molecular cloning of allene oxide cyclase – the enzyme establishing the stereochemistry of octadecanoids and jasmonates. J Biol Chem. 2000;275:19132–19138.10.1074/jbc.M002133200
- Strassner J, Schaller F, Frick UB, et al. Characterization and cDNA-microarray expression analysis of 12-oxophytodienoate reductases reveals differential roles for octadecanoid biosynthesis in the local versus the systemic wound response. Plant J. 2002;32:585–601.10.1046/j.1365-313X.2002.01449.x
- Reeves PH, Ellis CM, Ploense SE, et al. A regulatory network for coordinated flower maturation. PLoS Genet. 2012;8:e1002506.10.1371/journal.pgen.1002506
- Zhao P, Li Q, Li J, et al. Genome-wide identification and characterization of R2R3MYB family in Solanum lycopersicum. Mol Genet Genomics. 2014;289:1183–1207.10.1007/s00438-014-0879-4
- Miersch O, Neumerkel J, Dippe M, et al. Hydroxylated jasmonates are commonly occurring metabolites of jasmonic acid and contribute to a partial switch-off in jasmonate signaling. New Phytol. 2008;177:114–127.
- Suza WP, Rowe ML, Hamberg M, et al. A tomato enzyme synthesizes (+)-7-iso-jasmonoyl-l-isoleucine in wounded leaves. Planta. 2010;231:717–728.10.1007/s00425-009-1080-6
- Li C, Schilmiller AL, Liu G, et al. Role of beta-oxidation in jasmonate biosynthesis and systemic wound signaling in tomato. Plant Cell. 2005;17:971–986.10.1105/tpc.104.029108