Abstract
New application of fluorescence probe to detect apoplastic redox radicals from plant roots were sought. This probe can detect radicals selectively. Calibration curve for radicals was obtained using nitrogen monoxide as radical standard produced by NOC7. Apoplastic radicals released constitutively were quantified and the release rate was 60 μmol L−1 h−1. Oxidative burst triggered by chitin was distinguished from constitutive radical release.
Keywords:
ROS (reactive oxygen species) play important roles in the development of plant roots. For example, rboh (respiratory burst oxidase homologue), which is a NADPH oxidase homologue in plants, is highly expressed in cells and localizes ROS at sites with high elongation activity in root hairs [Citation1]. It has been reported that ROS, released into the apoplast by rboh, regulate root elongation by activating NSCC (non-selective cation channel) and promoting calcium ion inflow into cells [Citation2]. Although there are many reports on analytical methods for ROS [Citation3,4], reports on apoplastic ROS from intact plant roots are scarce. ROS is a generic term including superoxide anion radical (O2−•), hydrogen peroxide (H2O2), and hydroxyl radical (•OH), but in this report, we refer to O2−• and •OH as ROS radicals. Analyzing ROS radicals could help to understand the redox status of apoplast better than for H2O2, which passes through the plasma membrane freely. Liszkay et al. reported that ROS radicals, which were trapped by POBN (α-(4-pyridyl N-oxide)-N-tert-butylnitrone) in the presence of ethanol, were detected as carbon-centered radicals by electron spin resonance (ESR) [Citation5]. However, this method was not generally applicable because ESR instruments are not a widely available. Therefore, we sought a new analytical method for detecting apoplastic redox radicals. Fluorescent probes are a promising method for this purpose and are expected to be highly sensitive. Dihydrofluorescein, a popular prefluorescent skeleton, is widely used for ROS detection, but it is known to decompose due to photooxidation, and its photostability is low [Citation6]. Although there is a report that apoplastic redox radicals of rice root were detected using OxyBURST Green H2HFF BSA [Citation7], due to its instability, the amount of apoplastic redox radical might be obscured in the quantitative analysis. By irradiating OxyBURST Green H2HFF BSA, the background fluorescence increased about 100 times in 25 min. On the other hand, that of fluorescamine derivatized probe (1) increased only about 10 times (unpublished data). The fluorescence of this probe (1) is quenched by the intramolecular nitrone group and recovered by decomposition of the nitrone group [Citation8,9]. Among other similar fluorescent probes, this probe (1) was used due to its one step preparation reaction. This nitrone probe (1) was reported to react with oxidants and reductants, and gave peaks at different retention times [Citation10]. In the case of oxidants, the probe was oxidized, then rearranged and recovered its fluorescence (Figure (B) and ). In the case of reductants, the intramolecular nitrone group was reduced to a hydroxylamine and recovered its fluorescence (Figure (B) and ).
Figure 1. Synthetic and reaction schemes of the probe. (A) Synthetic scheme of pre-fluorescent probe (1). (B) Reaction scheme of probe (1). (1) was oxidized, then rearranged to (2) and recovered fluorescence. (1) was reduced to (3) and recovered fluorescence. In accordance with the report by Jia and colleagues, compounds (2) and (3) were characterized by LC/MS and their m/z were 395 and 473, respectively.
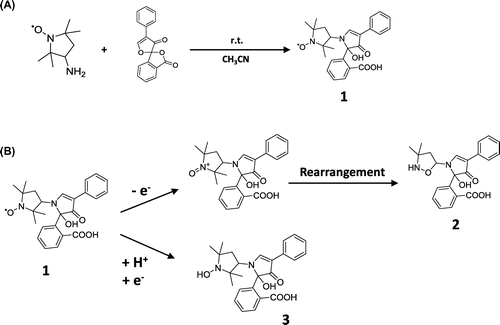
Figure 2. Chromatograms of in vitro reaction solutions using oxidants and reductants. HPLC conditions: column; TSK gel ODS 80-Ts QA (4.6 i.d. ×150 mm), mobile phase; 50 mM sodium acetate buffer (pH 4.0): methanol (2:3, v/v), flow rate; 1.0 mL/min, injection volume; 10 μL, column temperature; 30 °C. Only radical molecules were detected selectively. Reaction with oxidants; hydrogen peroxide and hydroxyl radical (A). Reaction with reductants; sodium ascorbate and glutathione (B).
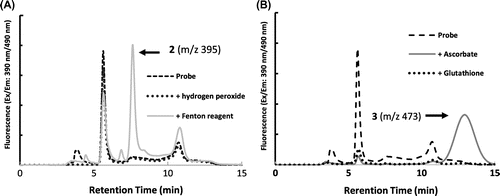
Figure 3. In vivo apoplastic radical analysis. An oxidative burst triggered by chitin was observed. About two times more radicals were released than by control samples by 60 min. Representative fluorescence chromatograms of the incubating solution with and without chitin (A). Apoplastic radical concentration after 60 min incubation, estimated using the calibration curve. Asterisk indicates a significant difference to control (*p < 0.01, n=3), analyzed by the Student’s t test (B).
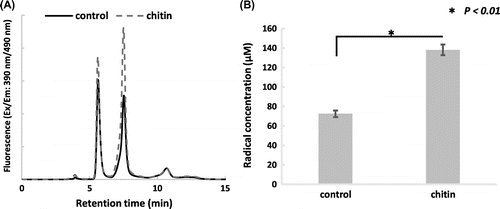
Probe (1) was synthesized from fluorescamine and 3-amino-2,2,5,5,-tetramethyl-1-pyrrolydinyloxy in accordance with the method of Li and colleagues (Figure (A)) [Citation11], and was stored in phosphate buffer (pH 7.5) at −20 °C till use. The yield of (1) was about 42%, and the purity of (1) was 82% by UV absorption at 254 nm by high-performance liquid chromatography (HPLC).
In order to confirm the reactivity of probe (1) in vitro, the oxidants and reductants were mixed with 300 µM probe (1) separately. •OH derived from Fenton’s reaction and H2O2 were used as oxidants. Sodium ascorbate (AsA) and glutathione were used as reductants. The reaction solutions were subjected to reverse phase (RP)-HPLC with fluorescence detection (excitation: 390 nm/emission: 480 nm). The assignment of each the obtained peaks on the chromatogram was determined by m/z of liquid chromatography–mass spectrometry (LC/MS) analysis after fractionation. The oxidative reaction gave a peak at 7.5 min with m/z 395 (2), on the other hand, the reductive reaction gave a peak at 13.0 min with m/z 437 (3). Furthermore, probe (1) did not react with H2O2 and glutathione, but did with •OH and AsA (Figure (A) and (B)). As a result, this probe selectively detected the redox radical molecules in the sample.
For the quantitative analysis of radicals, the nitrogen monoxide (NO) donor NOC7 was used. NOC7 supplies a certain amount of NO radicals with respect to time and its half-life is 5 min. NOC7 and 300 µM (1) were mixed in phosphate buffer (pH 7.4) and sampled 40 min later; i.e. approximately 99.6% of NOC7 had decomposed and twice as many moles of NO were generated. Then, the reaction mixture was subjected to RP-HPLC with fluorescence detection. As the result, peak (2) at 7.5 min, due to the oxidative reaction between probe (1), and this peak increased in proportion to the concentration of NO. A calibration curve was plotted using peak areas of (2) (supplemental data 1). Based on the decomposition rate of NOC7, the calibration curve contained an error in the range of 1% or less. The linear range of the calibration curve was between 5 and 100 µM.
For in vivo apoplastic redox radical analysis, seeds of soybean (Glycine max [L.] Merr.) were germinated at 25 °C for 3 days. Seedlings were grown on aerated incubation medium containing 1 mM CaCl2 for 12 h. Seedlings with a root length of 4–5 cm were used. Seedlings were soaked in incubation solution containing 1 mM of CaCl2 and 300 μM of (1) for 60 min in dark conditions. The incubation solution was subjected to the RP-HPLC with fluorescence detection. Only oxidative reaction peak (2) was detected at 7.5 min. Apoplastic ROS radical production, estimated using the calibration curve (supplemental data 1), was approximately 60–70 μmol L−1 h−1 per root.
We investigated whether the oxidative burst, in which excess ROS release outside of the cell was triggered by elicitors, can be detected by this method. Seedlings were soaked in incubation solution containing 1 mg/ml chitin for 60 min in dark conditions. In order to quantify radicals, the incubation solution was diluted twice with ultra-pure water and was subjected to RP-HPLC with fluorescence detection. The peak area of (2) was more than the control after 60 minutes’ incubation (Figure (A) and (B)). Apoplastic redox radicals triggered by the elicitor were estimated as approximately 120–150 µmol L−1 h−1 per root. These results are consistent with the previous report indicating that ROS triggered by chitin in Medicago polymorpha root increased twofold [Citation12].
In this work, a new application of quantitative analysis for apoplastic ROS radicals from plant roots was shown. This probe detected redox radicals selectively in the sample.
By this method, apoplastic redox radicals, released constitutively and from an induced oxidative burst, were distinguished quantitatively.
Author contribution
Y.I. and M.A. designed research; Y.I., M.Y. and S.K. performed research; Y.I. and M.A. wrote manuscript.
Disclosure statement
No potential conflict of interest was reported by the authors.
Funding
This work was supported by the Ministry of Education, Culture, Sports, Science and Technology, Japan [Program for the Strategic Research Foundation at Private Universities, 2014–2018].
Supplemental data
Supplemental data for this article can be accessed https://doi.org/10.1080/09168451.2017.1422108.
Supplemental_data_1.pdf
Download PDF (173.8 KB)References
- Foreman J, Demidchik V, Bothwell JHF, et al. Reactive oxygen species produced by NADPH oxidase regulate plant cell growth. Nature. 2003;422:442–446.10.1038/nature01485
- Demidchik V, Maathuis FJM. Physiological roles of nonselective cation channels in plants: from salt stress to signalling and development. New Phytol. 2007;175:387–404.10.1111/nph.2007.175.issue-3
- Halliwell B, Whiteman M. Measuring reactive species and oxidative damage in vivo and in cell culture: how should you do it and what do the results mean? British J Pharmacol. 2004;142:231–255.10.1038/sj.bjp.0705776
- Villamena FA. Reactive species detection in biology: chapter 4 Fluorescence Techunique. Amsterdam: Elsevier; 2017. p. 87–162.10.1016/B978-0-12-420017-3.00003-7
- Liszkay A, van der Zalm E, Schopfer P. Production of reactive oxygen intermediates (O2−•, H2O2, and •OH) by maize roots and their role in wall loosening and elongation growth. Plant Physiol. 2004;136:3114–3123.10.1104/pp.104.044784
- Zhao BZ, Summers FA, Mason RP. Photooxidation of Amplex red to resorufin: implications of exposing the Amplex red assay to light. Free Rad Biol Med. 2012;53:1080–1087.10.1016/j.freeradbiomed.2012.06.034
- Zhang CJ, Zhao BC, Ge WN, et al. An apoplastic H-type thioredoxin is involved in the stress response through regulation of the apoplastic reactive oxygen species in rice. Plant Physiol. 2011;157:1884–1899.10.1104/pp.111.182808
- Chen XQ, Tian XZ, Shin I, et al. Fluorescent and luminescent probes for detection of reactive oxygen and nitrogen species. Chem Soc Rev. 2011;40:4783–4783.10.1039/c1cs15037e
- You Y, Nam W. Designing photoluminescent molecular probes for singlet oxygen, hydroxyl radical, and iron-oxygen species. Chem Sci. 2014;5:4123–4135.10.1039/C4SC01637H
- Jia M, Tang Y, Lam YF, et al. Prefluorescent nitroxide probe for the highly sensitive determination of peroxyl and other radical oxidants. Anal Chem. 2009;81:8033–8040.10.1021/ac901374 m
- Li BB, Gutierrez PL, Blough NV. trace determination of hydroxyl radical in biological systems. Anal Chem. 1997;69:4295–4302.10.1021/ac970622b
- Kim SY, Sivaguru M, Stacey G. Extracellular ATP in plants. visualization, localization, and analysis of physiological significance in growth and signaling. Plant Physiol. 2006;142:984–992.10.1104/pp.106.085670