Abstract
Branched-chain α-keto acid dehydrogenase (BCKDH) kinase (BDK) suppresses the branched-chain amino acid (BCAA) catabolism by inactivation of the BCKDH complex. The muscle-specific BDK-deficient (BDK-mKO) mice showed accelerated BCAA oxidation in muscle and decreased endurance capacity after training (Xu et al. PLoS One. 12 (2017) e0180989). We here report that BCAA supplementation overcompensated endurance capacity in BDK-mKO mice after training.
Leucine, isoleucine and valine (BCAAs) are essential amino acids for mammals. In the catabolism of BCAAs, the first two steps are common to the three BCAAs. The first step is reversible transamination of BCAAs to form branched chain α-keto acids (BCKAs) by BCAA aminotransferase (BCAT). In the second step, BCKAs are converted to CoA-esters by BCKA dehydrogenase (BCKDH) complex. Because the second step is an irreversible reaction, it is believed to be the rate-limiting step in BCAA catabolism. The BCKDH complex is regulated by a phosphorylation-dephosphorylation cycle. In this cycle, BCKDH kinase (BDK) is responsible for inactivation of the complex by phosphorylation [Citation1,2], and BCKDH phosphatase is responsible for activation of the complex by dephosphorylation [Citation3,4]. The activity of the complex is quite low in rat and mouse skeletal muscle but extremely high in liver [Citation5,6]. BDK activity is inversely correlated with that of the BCKDH complex. Therefore, BDK is the responsible regulator for oxidation of BCAAs in skeletal muscle.
In our previous study [Citation7], we generated muscle-specific BDK deficient mice (BDK-mKO mice) which exhibited high BCKDH complex activity in muscle, resulting in promotion of BCAA oxidation and subsequent low BCAA concentrations in muscle and plasma. We found that endurance capacity enhancement by treadmill training for 2 weeks was significantly less in BDK-mKO mice than in control mice [Citation8], and glycogen and acetyl-CoA in skeletal muscle levels were lower in BDK-mKO mice than in control mice. These results suggested that acceleration of BCAA catabolism in skeletal muscle induced BCAA deficiency and perturbation of energy metabolism in tissues. However, in the previous study we could not conclude whether or not BCAA deficiency might be responsible for the low endurance capacity of BDK-mKO mice. Therefore, in the present study we examined the effect of BCAA supplementation during training on the endurance capacity and muscle glycogen content of BDK-mKO mice after training.
We generated BDK-mKO mice using the Cre-loxP system [Citation9], as described previously [Citation7]. These mice were housed at 23 ± 1 °C with lights on from 08:00 to 20:00 h. At 4 weeks of age, male mice were weaned and housed individually with free access to water and food (standard rodent chow CE-2, CLEA Japan, Tokyo).From 8 weeks of age, male control and BDK-mKO mice (n = 7 per group) were fed ad libitum an experimental diet (20 kcal% from protein, 70 kcal% from carbohydrate and 10 kcal% from fat; D12450B, Research Diets, Inc., New Brunswick, NJ, USA) until the end of the experiment. BCAAs were supplemented as 3% BCAAs in drinking water from 8 weeks of age until the mice were sacrificed. The BCAA composition ratio was 2:1:1 = leucine:isoleucine:valine. At the end of their 12th week (4 weeks’ supplementation of drinking water with 3% BCAAs), the endurance capacities of all the mice were assessed by measuring the distance ran until exhaustion using a motor-driven treadmill (Natsume, Tokyo, Japan) with a 10% grade. The running speed of the test was initially 15 m/min for 4 min, then increased at a rate of 1 m/min every 4 min. Then, all the mice were trained for 2 weeks (until 14 weeks of age) by running on the treadmill with a 10% grade for 60 min/day at a speed of 15 m/min during the first week and 18 m/min the following week, 5 days/week. The endurance capacities of all of the mice were assessed again using the above method 2 days after the last treadmill training session. Two days after the last capacity examination, the mice were killed by cervical dislocation after 8 hours’ fasting, then the skeletal muscles (soleus, gastrocnemius and plantaris muscles) of the left hind-limb were rapidly removed, freeze-clamped at liquid nitrogen temperature, and stored for glycogen and acetyl-CoA analysis. Blood was collected from the posterior vena cava and/or heart to prepare plasma, and then heart, liver, kidney, and epididymal adipose tissues were removed, freeze-clamped at liquid nitrogen temperature, and stored at –80 °C until analysis. The procedures described above are the same as those reported previously [Citation8], except that all of the animals ingested the BCAA drinking water during the experiment.
Muscle glycogen in the hind-limb muscle was determined by the phenol-sulfuric acid method, as reported previously [Citation10]. The amount of acetyl-CoA in the hind-limb muscle was measured with a PicoProbe Acetyl CoA Assay Kit (ab87546; Abcam, Cambridge, United Kingdom).Plasma samples were mixed with 3% (w/v) sulfosalicylic acid to give a final concentration of 1.5% and then centrifuged at 15000×g at 4 °C for 15 min to remove the precipitated proteins. The amino acid concentrations in the supernatants obtained were analyzed using an automatic amino acid analyzer (JLC-500/V; JEOL, Tokyo, Japan).
Data are expressed as means ± SEM. The data for the control and BDK-mKO mice were compared using a two-tailed Student’s t-test. A probability of less than 0.05 was considered to be statistically significant.
We previously reported that BDK-mKO had no effect on the growth rate or tissue weights of mice [Citation7]. In the present study, all the mice were given water supplemented with BCAAs during the experiment, and there was no difference in the body weight, or weights of skeletal muscle (soleus, gastrocnemius and plantaris muscles of left hind-limb), heart, liver, and epididymal adipose tissues between the control and BDK-mKO mice (Table ). The intake of BCAAs (the sum of BCAAs from the diet and water) was greater in the BDK-mKO mice than in the control mice (2.40 ± 0.03 g/week vs. 2.17 ± 0.07 g/week, respectively). These results indicate that BCAA supplementation did not affect the growth rate or tissue weights of the BDK-mKO mice.
Table 1. Body and tissue weights of the control and BDK-mKO mice with BCAA supplementation.
The distance ran to exhaustion in the present study was not different between the control and BDK-mKO mice before exercise training (1016 ± 129 m vs. 872 ± 113) (Figure ). After exercise training, the distance ran to exhaustion was increased in both the control and BDK-mKO mice (1826 ± 88 m and 2118 ± 71 m, respectively) and was significantly higher in the BDK-mKO mice than in the control mice (Figure ), indicating that BCAA supplementation was more effective in enhancing endurance capacity in BDK-mKO mice than in control mice. It should be noted that the distances ran due to training tended to be higher in the BDK-mKO mice with BCAA supplementation than that without BCAA supplementation (1528 ± 51 m) in the previous study, although the distances ran by the control mice after training were comparable with or without BCAA supplementation. These results suggest that an adequate supply of BCAAs is important in the training-enhanced endurance capacity of BDK-mKO mice, and that enhanced BCAA catabolism in muscle tissues is not a negative factor for endurance capacity.
Figure 1. Endurance capacity of control and BDK-mKO mice. The distance ran to exhaustion before and after 2 weeks’ training with BCAA supplementation. *Significant difference vs. control mice after training (p < 0.05).
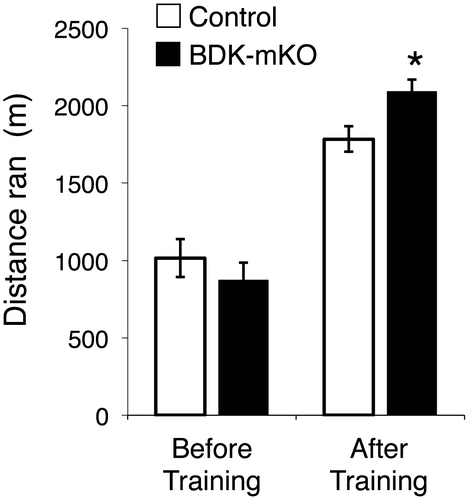
In the present study, the plasma leucine concentration in the BDK-mKO mice was ~16% lower than in the control mice, whereas the plasma concentrations of isoleucine and valine were not significantly different between the two groups (Table ). The BCAA concentrations of BDK-mKO mice in the present study were much improved by BCAA supplementation, given that the concentrations in the previous study without BCAA supplementation were ~50% that of the control mice [Citation8].
Table 2. Plasma amino acid concentrations of the control and BDK-mKO mice with BCAA supplementation.
In the present study, the plasma concentrations of alanine, arginine, asparagine, aspartate, glutamate, threonine, tryptophan, and tyrosine were significantly higher in the BDK-mKO mice than in the control mice (Table ). Of these amino acids, alanine, asparagine, aspartate, and glutamate may be related to BCAA transamination [Citation8]. Since the plasma concentrations of these amino acids in the previous study without BCAA supplementation were not significantly different between the control and BDK-mKO mice, the transamination of BCAAs in BDK-mKO mice was promoted in the present study by BCAA supplementation. The reason for the increases in the concentrations of the other amino acids (arginine, threonine, tryptophan, and tyrosine) remains unclear.
We showed in the previous study [Citation8] that muscle BCAA catabolism was promoted by BDK-mKO, which is associated with low glycogen content (1.8 ± 0.2 and 2.4 ± 0.2 mg/g tissue for BDK-mKO and control mice, respectively) and perturbation of energy metabolism in the skeletal muscle of BDK-mKO mice without acute exercise. A decreased acetyl-CoA level (less than 50% compared to control mice) in the skeletal muscle of the BDK-mKO mice was observed, indicative of perturbed energy metabolism [Citation8]. Therefore, we measured glycogen and acetyl-CoA in hind-limb skeletal muscle in the present study and found that the levels of both were the same in the BDK-mKO and control mice (glycogen: 2.2 ± 0.2 and 2.2 ± 0.3 mg/g tissue, respectively; acetyl-CoA: 3.3 ± 0.5 and 3.0 ± 0.4 nmol/g tissue, respectively). Since the glycogen content in the control mice was the same in the present and previous studies, it was not affected by BCAA supplementation. Therefore, we conclude that these parameters in the BDK-mKO mice were recovered by BCAA supplementation.
In this report, the endurance performance of BDK-mKO mice was higher than that of control mice after training. The plasma concentrations of BCAAs, muscle glycogen and acetyl-CoA in the BDK-mKO mice were almost recovered to the levels of the control mice. These results suggest that accelerated BCAA catabolism in muscle tissues may not be a negative factor for endurance performance, but rather may enhance endurance capacity when sufficient BCAAs are provided. Although it is unclear whether the findings obtained in the present study are applicable to humans, results to date clearly demonstrate that endurance exercise promotes BCAA oxidation in association with activation of muscle BCKDH complex in experimental animals and humans [Citation6,11,12]. Therefore, BCAA supplementation may be an aid for improving endurance performance.
Author contributions
MX, YS conceived and designed the experiments. The experiments were performed by MX, YK, DS. The data were analysed by MX, YK, YS. The article was written by MX, YS.
Funding
This work was supported by the JSPS KAKENHI [grant number 17H03817 from Grant-in-Aid for Scientific Research (B) (to YS), and No. 17H01965 from Grant-in-Aid for Scientific Research (B) (to YK)].
Disclosure statement
No potential conflict of interest was reported by the authors.
References
- Shimomura Y, Nanaumi N, Suzuki M, et al. Purification and partial characterization of branched-chain alpha-keto acid dehydrogenase kinase from rat liver and rat heart. Arch Biochem Biophys. 1990;283:293–299.10.1016/0003-9861(90)90645-F
- Popov KM, Zhao Y, Shimomura Y, et al. Branched-chain alpha-keto acid dehydrogenase kinase-molecular-cloning, expression, and sequence similarity with histidine protein-kinases. J Biol Chem. 1992;267:13127–13130.
- Joshi M, Jeoung NH, Popov KM, et al. Identification of a novel PP2C-type mitochondrial phosphatase. Biochem Bioph Res Co. 2007;356:38–44.10.1016/j.bbrc.2007.02.108
- Lu G, Sun HP, She PX, et al. Protein phosphatase 2Cm is a critical regulator of branched-chain amino acid catabolism in mice and cultured cells. J Clin Invest. 2009;119:1678–1687.10.1172/JCI38151
- Shimomura Y, Fujii H, Suzuki M, et al. Brancheded-chain alpha-keto acid dehydrogenase complex in rat skeletal muscle regulation of the activity and gene expression by nutrition and physical exercise. J Nutr. 1995;125:S1762–S1765.
- Shimomura Y, Honda T, Shiraki M, et al. Branched-chain amino acid catabolism in exercise and liver disease. J Nutr. 2006;136:250S–253S.10.1093/jn/136.1.250S
- Ishikawa T, Kitaura, Y, Kadota Y, et al. Muscle-specific deletion of BDK amplifies loss of myofibrillar protein during protein undernutrition, Scientific Reports. 2017; 7.
- Xu MJ, Kitaura Y, Ishikawa T, et al. Endurance performance and energy metabolism during exercise in mice with a muscle-specific defect in the control of branched-chain amino acid catabolism. PLoS ONE. 2017;11:e0180989.10.1371/journal.pone.0180989
- Bruning JC, Michael MD, Winnay JN, et al. A muscle-specific insulin receptor knockout exhibits features of the metabolic syndrome of NIDDM without altering glucose tolerance. Mol Cell. 1998;2:559–569.10.1016/S1097-2765(00)80155-0
- Lo S, Russell JC, Taylor AW. Determination of glycogen in small tissue sample. J Appl Psychol. 1970;28:234–236.
- Shimomura Y, Suzuki T, Saitoh S, et al. Activation of branched-chain alpha-keto acid dehydrogenase complex by exercise: effect of high-fat diet intake. J Appl Physiol. 1990;68:161–165.10.1152/jappl.1990.68.1.161
- vanHall G, MacLean DA, Saltin B, et al. Mechanisms of activation of muscle branched-chain alpha-keto acid dehydrogenase during exercise in man. J Physiol. 1996;494:899–905.10.1113/jphysiol.1996.sp021542