ABSTRACT
(2-Nitroethyl)benzene, methyl 4-methoxybenzoate and 4-methoxybenzaldehyde have been known as major scent components in flowers of the Japanese loquat Eriobotrya japonica [Rosales: rosaceae], together with 13 related benzenoids, including Z- and E-2-phenylacetaldoxime and benzyl alcohol. The scents air-trapped from a flowering panicle during 24 h incubation with d8-L-phenylalanine were composed of 15 deuterium labeled compounds {d6-styrene, d5-benzaldehyde, d7-2-phenylacetaldehyde, methyl d5-benzoate, d7 −2-phenylethanol, d7-2-phenylacetonitrile, d4-1,4-dimethoxybenzene, d7-Z-2-phenylacetaldoxime, d4-4-methoxybenzaldehyde, d7-E-2-phenylacetaldoxime, d4-4-methoxybenzyl alcohol, d7-(2-nitroethyl)benzene, methyl d4-4-methoxybenzoate, methyl d6-cinnamate and ethyl d4-4-methoxybenzoate}. On the other hand, hexane extracts of the flower petal incubate with a mixture of d5-Z- and d5-E-2-phenylacetaldoxime after 24 h indicated generation of six d5-labeld components {d5-benzaldehyde, d5-benzyl alcohol, d5-2-phenylacetaldehyde, methyl d5-benzoate, d5-2-phenylethanol, and d5-(2-nitroethyl)benzene}. By comparing those results, (2-nitroethyl)benzene was concluded as a product directly generated from a mixture of Z- and E-2-phenylacetaldoxime together with six minor benzenoids, while two major compounds (4-methoxybenzaldehyde and methyl 4-methoxybenzoate) together with three minors from L-phenylalanine, presumably via L-tyrosine. The other two minor components were derived from L-phenylalanine.
Graphical Abstract

Generation of (2-Nitroethyl)benzene and Related Benzenoids from L-Phenylalanine; Flower Scents of the Japanese Loquat Eriobotrya japonica [Rosales: Rosaceae]
The Japanese loquat Eriobotrya japonica [Rosales: Rosaceae], a fruit tree of evergreen, blooms in white with five petals during winter from December to March. The type of this flower is classified as panicle; each flower bud opens one by one randomly among each branch (consisting of 5–10 branches) with a conical form and stays in bloom at least for 4 days. Characteristic sweet flavors emitted from the flower were air-trapped using a solid phase adsorbent. The adsorbed flower volatiles, after eluted with hexane, were analyzed by a gas chromatography coupled with a mass spectrometry (GC/MS) analysis. The following 15 aromatic compounds (benzenoids) have been recovered; styrene (1), benzaldehyde (2), 2-phenylacetaldehyde (4), methyl benzoate (5), 2-phenylethanol (6), 2-phenylacetonitrile (7), 1,4-dimethoxybenzene (8), (Z)-2-phenylacetaldoxime (9), 4-methoxybenzaldehyde (10), (E)-2-phenylacetaldoxime (11), 4-methoxybenzyl alcohol (12), (2-nitroethyl)benzene (13), methyl 4-methoxybenzoate (14), methyl cinnamate (15) and ethyl 4-methoxybenzoate (16), along with five terpenoids, as reported [Citation1]. Among them, (2-nitroethyl)benzene (13, 32.7%), 4-methoxybenzaldehyde (10, 27.3%) and methyl 4-methoxybenzoate (14, 24.6%) has been the major components. Presence of 13 as a flower scent is the first finding in Japanese plants [Citation1], while 13 has been known as the essential oil component from Brazilian and African plants [Citation2–Citation4]. Natural distribution of NO2-containing molecule seems to be not common, but it has actually been distributed widely as a minor component in many flower scents from orchids and others [Citation5–Citation9]. The occurrence of 13 has also been known in millipedes, as a minor component of their defense allomone in Eutrichodesmus elegans (Miyosi) and E. armatus (Miyosi) [Polydesmida: Haplodesmidae][Citation10].
Biosynthesis of 13 has been reported in a plant as an intermediate to produce benzylglucosinolate from L-phenylalanine via 2-phenylacetaldoxime [Citation11]. In the recent biosynthetic study of the flower volatiles from the Japanese loquat Eriobotrya japonica, however, the presence of 13 has been lost [Citation12] together with seven benzenoids (1, 5, 7, 8, 11, 15 and 16), with unknown reason. On the other hand, zingerone and phenethyl benzoate have not been detected in our results [Citation1].
Actual generations of 10, 13 and 14 as major components from the Japanese Loquat flowers were reconfirmed this time together with 13 minor benzenoids {1 – 9, 11, 12, 15 and 16, including benzyl alcohol (3)}, not only in trapped volatiles by Mono Trap RCC18 during administration of d8-L-phenylalanine but also in conventional hexane extracts of petal incubates with d5-9 & −11. By comparing both results, biosynthetic pathways of flower scent volatiles were elucidated.
Materials and methods
Flower
Flowering shoots of the Japanese loquat Eriobotrya japonica [Rosales: Rosaceae] was obtained from garden in Kyoto Japan, in winter 2012–2013, and kept for a while in a cold room (ca. 4 °C) with water after covering with polyethylene bag (23 cm x 34 cm, 0.02 mm in film thickness, Yazaki Kako Corporation, Japan) to avoid desiccation during storage.
Incubation with d8-L-phenylalanine and collection of volatiles by mono trap RCC18
The following two flowering panicles (shoots), after removal of leaves, were selected for collection of flower scents as indicated in ; one (right; old flower, OF) was a panicle with more than ten flowers in their full-bloom, and the other (left; new flower, NF) was a panicle with three flowers in their full-bloom. Both flower shoots were separately immersed to a glass vial (27 mm i.d. and 55 mm in height), where d8-L-phenylalanine (50 mg, Cambridge Isotope Laboratories, Inc.) dissolved in water (2 mL) had preliminary been introduced. After hanging a clean Mono Trap RCC18 block (tube-like solid phase adsorbent, 5 mm in length, 3 mm o.d. and 1.5 mm i.d., GL Science Inc., Japan) by a wire (ɸ 0.28 mm, stainless steel), the shoot was each loosely covered by a clean polyethylene bag, not only to avoid dilution of volatiles to the atmosphere, but also to facilitate exposure to the adsorbent, and volatiles were air-trapped at 24°C as reported [Citation1].
Figure 1. Photograph of panicle incubations {left: new flower (NF), right: old flower (OF)} with d8-L-phenylalanine administered as aqueous solution in vials. Arrow indicates a mono trap RCC18 hanged with steal wire. After setting the trap to both shoots, both flowers were covered by polyethylene bags to collect flower scent volatiles.
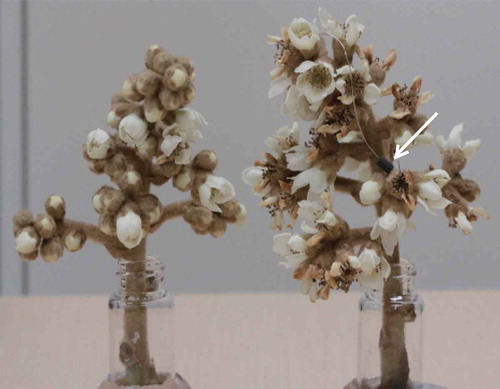
Adsorbents were changed twice, each after 24 h of exposure; the first 24 h period (p1) and the latter 24 h (p2) for both flower shoots (NF and OF). Air-trapped volatiles were each recovered by dipping the adsorbent in hexane (50 μL) for three minutes, using a micro tube (Agilent, flat-bottomed micro-insert, 31 mm in length, ɸ 5.6 mm) carefully to avoid contamination due to handling, and then the hexane extracts (1 μL) of the volatiles was analyzed by GC/MS. The composition (%) of volatiles was assessed by RIC, and contents (%d) of d-labeled isomers by SIC, as mentioned later.
The solid phase adsorbent block was preliminary washed with hexane using a Soxhlet extractor, and stored in hexane before use to avoid contamination during handling.
Incubation of petals from a whole flower with d5-9 & −11 (incubation 1)
All five petals from a freshly opened flower were removed, and transferred as a group into a glass vial (1.5 mL volume, 11.7 mm o.d. x 32 mm in length). To the petals, d5-9 & −11{7.12 μg, a mixture of d5-9 (56%) and d5-11 (44%) in 50 μL water} was added as the substrate, and incubated at r.t overnight after sealed with a plastic cap. Hexane (250 μL) was added to the petal incubate, and then the hexane layer (4 μL) after three minutes was subjected to GC/MS analysis. Contents (%d) of d-labeled isomers were assessed by SIC, as mentioned later.
Incubation method for flower petals with d5–9 & −11 (incubation 2)
All ten petals that were removed from two freshly opened flowers were each transferred into flat-bottomed glass tubes [31 mm in length, 5.5 mm o.d., containing water (5 μL)] by a fine forceps, and each lower tip of petals was made touch the water at the bottom of tube. Five tubes as a group were then placed separately in two glass vials (1.5 mL volume, 11.7 mm o.d. x 32 mm in length) and sealed with a plastic cap to prevent desiccation.
The following two sets of conditions were prepared for each vials (five tubes, each); one (Incubation 2–1, Intact petal) was incubated, as it is, after applying d5-9 & −11 {0.1 μg, a mixture of d5-9 (56%) and −11(44%) in 11 μL water} to each tubes at r.t. overnight. The other set (Incubation 2–2, Heat denatured petal) in the sealed vial with cap was heated in a water bath (at 90 °C) by dipping for 1 h, in order to denature enzyme(s) preliminary. After cooled to r.t., the substrate, d5-9 & −11 (0.1 μg, in 11 μL water), was added as well, and incubated overnight. After incubation, hexane (50 μL) was added to each tube, and the hexane extract (4 μL) was subjected to GC/MS analysis after 3 minutes. The composition (%) of volatiles in Incubation 2–1 was assessed by RIC as representative among petal incubations, and contents (%d) of d-labeled isomers in Incubation 2–1 and 2–2 by SIC, as mentioned later.
Running condition of GC/MS system
7890A GC system (Agilent Technologies Inc.) was used, as reported previously, by coupling with a 5975C Inert XL EI/CI MSD with a triple axis mass selective detector operated at 70 eV, using an HP-5MS capillary column (0.25 mm i.d. × 29 m, 0.25 μm film thickness; Agilent Technologies Inc.)[Citation13]. Helium was used as the carrier gas at a flow rate of 1.00 mL/min, with a split-less mode at a temperature programmed to change from 60°C (2 min) to 290°C at a rate of 10°C/min. This temperature was maintained for 5 minutes. GC and GC-MS data were processed using Chemstation (Hewlett Packard Co.), with reference to an MS database (Wiley275 library; Hewlett Packard Co.).
Relative compositions (%) of gas chromatogram were assessed by integration of reconstructed ion chromatogram (RIC). In cases of non-resolved peaks composed of a compound and its d-labeled isomer(s), their compositions (%d) of d-labeled isomer in each peaks were assessed by selected ion chromatogram (SIC), using base ions of each corresponding components as monitors. Typical examples were shown later in and .
Chemicals
α,β,β,2,3,4,5,6-d8-L-phenylalanine was obtained from Cambridge Isotope Laboratories, Inc. A thermo-dynamic equilibrium mixture of d5-9 &-11 was prepared starting from 2,3,4,5,6-d5-bromobenzene (Cambridge Isotope Laboratories, Inc.). Ethylene oxide {generated from 2-chloroethanol (24.8 g) with KOH (31 g) and dissolved in ether (75 mL)} was added carefully to the Grignard reagent prepared from 2,3,4,5,6-d5-bromobenzene (10 g) and magnesium (1.8 g) to give d5-6 (9.0 g). The purified d5-6 (1.1 g) by a SiO2 column was then oxidized to d5-aldehyde (d5-4) by pyridinium chlorochromate (2.2 g) in methylene chloride (60 mL). After filtrated on a Florisil layer and concentrated in vacuo, the product that contained d5-2 and other unknown by-products (contaminated reason; remains obscure), was purified on a SiO2 column (Hex: EtOAc, 5:1) to obtain pure d5-2-phenylacetaldehyde (d5-4). The aldehyde (d5-4) after concentrated in vacuo was subsequently mixed with 50% aqueous hydroxylamine (15 mL), and the resulting oily solid after extraction with ethyl acetate was again chromatographed on a SiO2 column to obtain pure d5-9 & −11{152 mg, 13.7% yield (from d5-6), mp 99.5–100.5°C (after re-crystallized in hexane), an equilibrium mixture of d5-9 (56%, > 99.28%d) and d5-11 (44%, > 99.29%d)}. The mixture (d5-9 & −11) was dissolved in water as a 1,000 ppm solution and stored at 4°C before use.
A non-labeled mixture of 9 (23%) and 11 (77%) were prepared by mixing 2-phenylacetaldehyde {4, generated from 2-phenylacetaldehyde dimethylacetal (available commercially from Nacalai tesque Co. Ltd. Kyoto, Japan) by acid hydrolysis} with 50% aq. hydroxylamine. The resulting crystalline mass was separated by filtration, and re-crystallized in hexane.
(2-Nitroethyl)benzene (13) was prepared from 1-bromo-2-phenylethane and sodium nitrite by refluxing in acetone, after leading 1-bromo-2-phenylethane to 1-iodo-2-phenylethane. Structure of 13 was confirmed by GC/MS, comparing with that of millipede Thelodesmus armatus [Polydesmida: Haplodesmidae][Citation10,Citation14]. The compound 4 was prepared from 2-phenylacetaldehyde dimethylacetal as mentioned above. The compound (5) was prepared by dissolving benzoic acid in methanol and by refluxing overnight with catalytic amount of conc. H2SO4. Methyl 4-methoxybenzoate (14) was converted to 4-methoxybenzyl alcohol (12) by LiAlH4 reduction in dry ether. The compound (14) was also converted to ethyl 4-methoxybenzoate (16) by refluxing in ethanol with catalytic amount of NaOH. All the other compounds (1, 2, 3, 6, 7, 8, 10, 14, 15) were obtained commercially, and their tRs and mass spectra were measured as hexane solution (1000 ppm).
Results
Detection and identification of flower scent volatiles by GC/MS after incubation of d8-L-phenylalanine and d5-9 & −11
Mass spectra of the natural components from flower scents and all d-labeled isomers, not only in air-trapped volatiles derived from d8-L-phenylalanine incubation but also in hexane extracts of d5-9 & −11 incubates, are summarized in . All compounds were identified by comparison with those of authentic standards as indicated, and structures of all d-labeled isomers were elucidated by their mass spectra. Those d-labeled isomers indicated shorter tRs (ca. 0.02 – 0.06 min) than those of natural components characteristically, and similar mass fragmentation patterns with difference due to numbers of deuterium present in each fragments.
Table 1. Gas chromatographic and mass spectral data of flower scent compounds from Japanese loquat Eriobotrya japonica after incubation by d8-L-phenylalamnine and by a mixture of d5-Z- and -E-2-phenylacetaldoxime.
Numbers of deuterium atoms in each molecule varied from four to seven among components after d8-L-phenylalanine incubation (mentioned later and ), as follows; d7-compounds (4, 6, 7, 9, 11 and 13), d6-compounds (1 and 15), d5-compounds (2, 3 and 5) and d4-compounds (8, 10, 12, 14 and 16). When flower petals were incubated with d5-9 & −11, those d7-labeled four compounds (4, 6, 7 and 13, by d8-L-phenylalanine incubation) were recovered as d5-compounds (mentioned later), indicating biogenesis via 9 & 11 from L-phenylalanine.
Table 2. d-Labeled content (%) of flower scents from Loquat after incubation with d8-L-phenylalanine.
The base ion (m/z 110) of d7-13 was the same to the M+ ion (and the base ion) of d6-styrene (d6-1)(). This fact means that the base ion of d7-13 is derived after elimination of one deuterium from the molecule as neutral DNO2. All the other compounds after administrations of d8-L-phenylalanine and of d5-9 & −11 () indicated the same m/z differences of each molecular ions and also of each base ions between natural components and each its d-labeled isomers.
D8-L-phenylalanine administration to flowering panicle
A typical GC profile (, with SIC) of air-trapped volatiles during administration of d8-L-phenylalanine (OF-p2, mentioned later) was mainly composed of three peaks (10, 13 and 14) together with 10 minors (1, 2, 4 – 8, 12, 15 and 16), and the profile was the same as reported [Citation1], though peaks (3, 9 and 11) were apparently not observed due to contamination and too small amounts. Administration results are summarized in , as follows; 1) M+ and the base ions for SIC, and incorporated numbers of deuterium atoms. 2) peak area (%) of scent components in NF-p1 (new flower during 0 – 24 h) as representative (results were simiar to those reported [Citation1]), and 3) d-labeled isomer contents (%d) from new (NF) and old flowers (OF) during two periods {0 – 24 h (p1), and 24 – 48 h (p2)} with content (%d) ratios (p2/p1).
The d6-1 content in NF-p1 was 45.5%d, while that increased to 77.3%d in NF-p2. In OF the shift from p1 to p2 was not clear (from 15.0%d to 15.5%d). Content of d5-2 changed from 37.6%d in NF-p1 to 12.8%d in NF-p2, while its value was shifted from 21.4%d in OF-p1 to 10.0%d in OF-p2. Content of d7- 4 changed from 24.4%d in NF-p1 to 19.9%d in NF-p2, and shifted from 14.7%d in OF-p1 to 6.4%d in OF-p2.
Likewise, d5-5 changed from 34.9%d in NF-p1 to 12.4%d in NF-p2 and from 32.1%d in OF-p1 to 11.8%d in OF-p2. Content of d7-6 changed from 33.2%d in NF-p1 to 17.8%d in NF-p2 and from 28.3%d in OF-p1 to 10.9%d in OF-p2. Content of d7-7 shifted from 54.2%d (the largest incorporation rate among all components) in NF-p1 to 11.0%d in NF-p2, and from 27.5%d (the fifth largest value after 32.1%d of d5-5) in OF-p1 to 5.1%d (the lowest rate among all components) in OF-p2. Content of d4-8 maintained almost the same level during p1 and p2 periods, from 27.5%d to 25.1%d in NF and from 17.4%d to 17.7%d in OF. The similar tendency was observed on d4-14; from 28.0%d to 24.8%d in NF and from 29.4%d to 28.4%d in OF.
Non-labeled (Z)- & (E)-2-phenylacetaldoxime (9 & 11) were each detected as a set of the same ions (m/z 91, 117 and 135) at tR 10.14 min and 10.44 min, respectively, whereas corresponding regions to d7-9 & −11 at tR 10.08 min and 10.38 min did not indicated reliable abundances of ion set (m/z 98, 124 and 142, not indicated in ). As a result, percentages of d7-9 & −11 were not obtained.
Content of d4-10 changed from 36.3%d in NF-p1 to 15.4%d in NF-p2 and from 20.0%d in OF-p1 to 8.1%d in OF-p2. Content of d4 −12 was almost the same during p1 and p2 periods in NF (19.1%d and 20.7%d), while in OF, it decreased from 25.1%d in p1 to 14.1%d in p2. Content of d7-13 changed from 44.1%d in NF-p1 to 10.0%d in NF-p2 and from 22.5%d in OF-p1 to 7.1%d in OF-p2. Content of d6-15 changed from 31.5%d in NF-p1 to 5.2%d in NF-p2 and from 39.5%d in OF-p1 to 12.6%d in OF-p2. Content of d4-16 changed from 34.9%d in NF-p1 to 30.0%d in NF-p2, and from 29.1%d in OF-p1 to 36.4%d in OF-p2.
The following eight compounds (5, 6, 7, 8, 10, 13, 14 and 15) indicated the similar 24 h period ratios (p2/p1) between NF and OF, and seemed to be constantly observable components. Among them, 10 (27.3%), 13 (32.7%) and 14 (24.6%) were major scent components as indicated in , occupied 84.6% of the total volatiles in NF. On the other hand, three compounds (1, 4 and 12) decreased and two (2 and 16) increased.
The following mono-substituted benzenoids [1 (45.5%d), 7 (54.2%d) and 13 (44.1%d)] in NF-p1 demonstrated larger labeled rates than the following di-substituted benzenoids [8 (27.5%d), 10 (36.3%d), 12 (19.1%d), 14 (28.0%d) and 16 (34.9%d)] in the same period. In the subsequent 24 h period (p2), 7 and 13 decreased to 11.0%d and 10.0 %d, while 8 (25.1%d), 12 (20.7%d), 14 (24.8%d and 16 (30.0%d) maintained similar level, except the case of 1 (increased to 77.3%d) and 10 (decreased to 15.4%d). These facts might be contributing to control flavor composition along with flower ages.
D5-9 & −11 incubation using a whole flower petals (incubation 1)
Results are summarized in (Incubation 1) and (with SIC). Total 25 peaks were observed in hexane extract from flower petals after incubation with d5-9 & −11, including hydrocarbons (n-C21, n-C23, n-C25 and n-C29), and alkanals {presumably C22-CHO and C24-CHO, both showed similar mass spectrum pattern to n-C17-CHO (Library ID 91%)}. The following nine compounds looked over-dosed incubation, and resulted more than 89%d of d-labeled isomers; 2 (84.5%d), 3 (94.1%d), 4 (98.8%d), 5 (89.1%d), 6 (99.6%d), 7 (98.6%d), d5-9 (100%d), d5-11(100%d) and 13 (96.2%d), including administered d5-9 & −11. On the other hand, no trace of d-labeled isomers was detected at all in other seven benzenoids (1, 8, 10, 12, 14, 15 and 16, corresponded to 51.8% of total benzenoids contents) and components extracted by hexane (hydrocarbons and alkanals, tR 19.78 min – 26.20 min).
Table 3. Major components of hexane extracts after incubation of flower petal by d5-9 & −11.
As a result, it is concluded that there is no evidence of conversion that took place from 9 & 11 to L-phenylalanine, and that all compounds observed (2, 3, 4, 5, 6, 7, 9, 11 and 13) were derived only from administered 9 & 11.
D5-9 & −11 incubation with a petal (incubation 2–1)
As shown in – Incubation 2 (2–1) and , total nine compounds (2, 3, 4, 5, 6, 7, 9, 11 and 13) contained d-labeled isomers, including administered d5-9 &-11 (2.3% & 2.1%) and non-assessable 7 (due to overlapped impurity). (2-Nitroethyl)benzene (13) was the most abundant component (36.1%) among nine benzenoids and 17.4% among all peak responses of the hexane extracts, and 2-phenylethanol {6, 7.7% (3.7%), intensity ca. 1/5 of 13} was the second major product. The other four (2, 3, 4, and 5) were all trace {less than 0.1% (0.1%)}, detectable barely enough by SIC (, ).
On the other hand, d-isomer contents of the following 8 compounds {total peak area 48.2% (23.2%), including d5-9 & −11} were assessed within a moderate range (13.7%d – 42.0%d) {-Incubation 2 (2–1)} to compare each other; 2 (17.8%d), 3 (13.7%d), 4 (41.0%d), 5 (15.6%d), 6 (42.0%d) and 13 (41.6%d). Among them, compounds (4, 6 and 13) showed each more than 40.0%d of deuterium up-take and possessed C6 + C2 structural moiety in their molecule. Peak area of the three compounds occupied 43.8% of benzenoid products, indicative of active productivity. Whereas, compounds (2, 3 and 5) indicated deuterium up-take each lower than 18.0%d, and all were trace components (less than 0.1%) with C6 + C1 moiety (after cleavage of one carbon from C6 + C2 structure). Then, these three compounds seemed to be produced by a minor pathway of biogenesis.
Like the case of Incubation 1 (mentioned above), seven benzenoids (1, 8, 10, 12, 14, 15 and 16, corresponded to 51.8% of total benzenoids contents) did not contain d-labeled isomers () and the fact was also true in the present Incubation 2–1, indicating that d5-9 & −11 were not function as their precursor. Styrene (1) biosynthesis was actually not observed in the present incubation (), while d6-1 was obtained from d8-L-phenylalanine incubation as described before. It was no wander because 1 has been known as a decarboxylation product from 15 [Citation15]. Dehydration from 9 & 11 to 7 seemed to be suppressed by low availability of 9 & 11 {total 4.4% (2.1%) as mentioned above}, and 7 looked to be consumed rapidly to 2, 3 and 5 (all trace, < 0.1%d).
D5-9 & −11 incubation with a heat denatured petal (incubation 2–2)
Incubation of d5-9 & −11 with heat denatured petals demonstrated puzzling results (). Production ability of 5 and 13 was completely lost, then, it was sure that these two were produced by heat sensitive process such as enzyme reaction from 9 & 11. While all the other compounds (2, 3, 4, 6 and 7) each contained large amounts of corresponding d-labeled isomer (contents more than 88.7%d, each), and seemed to be produced anyhow. There might be two possibilities, by thermo-stable enzyme(s) or by chemical degradation, but also due to incomplete inactivation by the present heat treatment. Prominent d-labeled isomer content of 4 (95.5%d) and those of subsequently generated 6 (94.0%d) were almost the same to those of d5-9 & −11 (94.8%d & 95.7%d), indicative of degradation (no isotope dilution effect) or no natural compounds present in the incubated condition due to heat treatment. Generation of 7 (94.8%d) was also understandable as non-enzymatic dehydration from 9 & 11, but that of 2 (89.4%d) and subsequent reduction to 3 (88.7%d) remains obscure, together with reduction to 6 from 4.
Discussion
Matsuo, Kirkland and Underhill (1972) have demonstrated by radioactive 14C tracer experiments that 9 & 11 are precursors of 13, using shoots of Tropaeolum majus L [Geraniales: Tropaeolaceae][Citation11]. Then, biosynthesis of 13, a major flower fragrance of the Japanese loquat, was examined applying d8-labeled-L-phenylalanine and d5-9 & −11 as precursors. Both precursors were successfully incorporated into the plant, simply by dipping shoots or petals into the aqueous substrate solution. Results were, unexpectedly informative, to elucidate biogenetic pathways not only of 13 but also of all benzenoids (total 16 compounds) detectable as flower fragrances in the Japanese loquat, as summarized in .
Figure 4. A proposed pathway of (2-nitroethyl)benzene and other related benzenoids detected in loquat flower scents, derived from L-phenylalanine by way of Z- and E-2-phenylacetaldoxime. Compounds in blanket were not detected in the present methods. Broad open arrows indicate reactions to major components. Solid line: reaction pathway(s) reported or conceivable, doted line: suggestive pathway, including non-enzymic step(s) partly.

It was demonstrated that there were three pathways (routes A, B and C) from phenylalanine to explain all sixteen benzenoids (1 – 16) in the flowers, as follows ( and ); route A) from d8-L-phenylalanine to reach d4-compounds {8 (peak area < 0. 1%), 10 (27.3%), 12 (0.9%), 14 (24.6%) and 16 (0.1%)} by way of putative steps (L-tyrosine, 4-hydroxy- and 4-mthoxybenzoic acid, not detected by solid phase absorption nor by hexane extraction). Although a part of step(s) from d8-L-phenylalanine to reach 14 has been proposed [Citation12], involvement of L-tyrosine have not been mentioned as the product of L-phenylalanine hydroxylation, possibly because of no enzyme(s) seemed to be known in plants. Route B) was from d8-L-phenylalanine to d6-compounds {1 (0.2%) via 15 (7.2%)}, possessing one extra deuterium atom outside of benzene ring. The compound 1 is known as the de-carboxylation product of 15 [Citation15]. Route-C) was from d8-L-phenylalanine to d7-compounds [a mixture of 9 (0.2%) and 11 (0.6%)], which were then separated to three groups of d7-compounds {C-1: after oxidation to 13 (32.7%), C-2: degradation to 4 (0.1%) and subsequent reduction to 6 (3.4%), and C-3: dehydration to 7 (1.0%)}. Because 7 has been known as a precursor of mandelonitrile biosynthesis in polydesmid millipedes [Citation16], and also presence of hydroxynitrile lyase has been known in the Japanese loquat [Citation17], involvement of mandelonitrile (not detected) might be reasonably explainable for occurrences of 2 (1.6%, by degradation of mandelonitrile), 3 (< 0.01%, reduced from 2) and 5 {0.2%, by multi-step(s) product}; not only from benzoic acid (not detected) after oxidation of 2, but also by β-oxidation of 15 (not listed in )}.
On the other hand, the following seven compounds (1, 8, 10, 12, 14, 15 and 16) were not produced by administration of 9 & 11. The fact means that no reaction takes place from 9 & 11 to L-phenylalanine. As a result, all 16 benzenoid components in flower scents of the Japanese loquat Eriobotrya japonica, not only the major components (10, 13 and 14), but also other minors (1 – 9, 11, 12, 15 and 16), were demonstrated as products derived solely from L-phenylalanine.
As mentioned in the previous study [Citation1], compound(s) with nitro group(s), such as 13, is generously uncommon as the natural product, except essential oil component from several plants. The compound (13) is known as the essential oil components in Ocotea pretiosa [Laurales: Lauraceae][Citation4] and Aniba canelilla [Laurales: Lauraceae][Citation18] emanating a powerful sweet-floral, warm-spicy odor reminiscent of cinnamon, and it is also widely distributed as a constituent of many flowers (e.g. Madagascar jasmine Stephanotis floribunda [Gentianales: Asclepiadaceae][Citation5], bitter orange Citrus x aurantium [Sapindales: Rutaceae] [Citation6], and many species of orchids [Citation7], the Colombian ylang-ylang Cananga odorata, [Magnoliales: Annonaceae] [Citation8], and tomato Solanum lycopersicum [Solanales: Solanaceae] fruits [Citation9].
Among them, its extremely high content is known in the following two plants; the Brazilian plant A. canelilla [Citation18], and the Nigerian spicy medicinal plant Dennettia tripetala G. Baker [Magnoliales: Annonaceae][Citation2 and Citation19]. In the Brazilian plant, the compound (13) is present in substantial quantities – up to more than 70% of the essential oil from the bark [Citation18]. The leaf oil from D. tripetala is composed of the compound (13, 53.7%), methyl eugenol (22.8%), and linalool (15.7%) together with other 45 components (7.3%)[Citation2]. The compound 13 (over 70%) is, furthermore, reported as its leaf and bark oil from the same plant [Citation19].
Two Japanese polydesmid millipedes, Eucondylodesmus elegans Miyosi (Polydesmida: Haplodesmidae) and E. armatus Miyosi (Polydesmida: Haplodesmidae) also possess 13 as a minor compound, presumably as the precursor of the major defense components (1Z)- and (1E)-2-nitroethenylbenzenes [Citation10]. Biosynthesis of α,β,1,2,3,4,5-d7-(1E)-2-nitroethenylbenzene has been confirmed by a primitive feeding experiment using contaminated diet with d8-labelled L-phenylalanine [Citation20].
Among 325 species of flower scent studied (23 families, 16 orders) (), 13 is distributed in 198 species (ca. 61%), composed of 192 species {among which 159 species (ca. 69%), belong to Orchidaceae [Asparagales] together with 7 and 9 & 11, of four species only with 7, and of 2 species that only possess 13 [Citation21]. On the other hand, 7 is distributed independently in total 126 species (ca.39%) (among which 73 species were Orchidaceae) and one species contained 7 and 9 & 11. There is no species known to possess 9 & 11 only, suggestive of its less stable nature than 7 and 13. Then, 9 & 11 seems to be accompanied with 7 and(or) 13, and to be the biogenic precursor of them.
Table 4. Distribution of (2-nitroethyl)benzene (Nitro), 2-phenylacetaldoximes (Oxime) and 2-phenylacetonitrile (Nitrile) in the plant kingdom as components of flower scents (El-Sayed AM 2017).
The present flowers, 9 & 11 seemed to be utilized in three ways, not only to produce 13 or 7, but also to both compounds at a time. The oxidation reaction from 7 to mandelonitrile has been elucidated as the function of a P-450 (CYP3201B1) in millipedes [Citation16], and then the oxidation process from 9 & 11 to 13 in the present flower scent and two cases of Haplodesmid millipedes [Citation10] might be similarly conceivable as the function of a putative P-450 enzyme.
As the natural product, distributions of nitroalkanes are known, such as 1-nitro-2-methylpropane and 1-nitro-3-methylbutane of tomato S. lycopersicum fruits flavors [Citation22]. Based on our results, those compounds might be P-450 oxidation products from isoleucine and leucine, by way of 2-methylbutanaloxime and 3-methylbutanaloxime, respectively. Similar generation of isovaleraldoxime, isovaleronitrile and 3-methyl-1-nitrobutane from L-leucine by herbivory have recently been reported in the common evening primrose Oenothera biennis [Myrtales: Onagraceae] that stimulate foraging behavior of the predatory blue shield bug Zicrona caerulea [Heteroptera: Pentatomidae][Citation23].
4-Methoxybenzaldehyde (10, p-anisaldehyde, 27.3%, the second major component) is widely distributed as one of the common floral scents identified in Angraecum [Asparagales: Orchidaceae], Cypripedium [Asparagales: Orchidaceae], Fragaria [Rosales: Rosaceae], Hypecoum [Ranunculales: Papaveraceae], Nymphaea (Nymphaeales: Nymphaeaceae), Ophrys [Asparagales: Orchidaceae], and Syringa (Lamiales: Oleaceae) [Citation24], and known as a potent attractant for pollinator such as adults of the varied carpet beetle, Anthrenus verbasci [Coleoptera: Dermestidae] irrespective of sex. The combined attractant preparation with the sex pheromone demonstrates twice the sum of catches baited with each single attractant [Citation25]. Methyl 4-methoxybenzoate (14, methyl p-anisate, 24.6%, the third major component) is also distributed as common floral scents in Asparagales: Orchidaceae, Ericales: Ericaceae, Primulales: Theophrastaceae, Ranunculales: Fumariaceae, and Rosales: Rosaceae [Citation21].
In conclusion (), all of 16 benzenoids detectable in the flower scents of the Japanese loquat Eriobotrya japonica were demonstrated as L-phenylalanine origin. (2-Nitroethyl)benzene (13, one of the three major components) and six minors (2 – 7) were products from L-phenylalanine via (Z)- and (E)-2-phenylacetaldoximes (routes C-1, C-2 and C-3), while 4-methoxybenzaldehyde (10) and methyl 4-methoxybenzoate (14), together with 3 minors (8, 12, and 16) were derived from L-phenylalanine after 4-hydroxylation (route A). The two other minors (1 and 15) were from L-phenylalanine (route B).
Author contribution
YK conceived and designed research, conducted experiments and analyzed data. YK and YA wrote the manuscript. All authors read and approved the manuscript.
Acknowledgments
We would like to thank Dr. Kimiyasu Isobe of our project for his critical reading of this manuscript.
Disclosure statement
No potential conflict of interest was reported by the authors.
Additional information
Funding
References
- Kuwahara Y, Ichiki Y, Morita M, et al (2-Nitroethyl)benzene: a major flower scent from the Japanese loquat Eriobotrya japonica [Rosales: rosaceae]. Biosci Biotech Biochem. 2014;78:1320–1323.
- Adeoti SB, Ayedoun MA, Leciercq PA. Essential oil of Denettia tripetala leaves from Benin. J Essent Oil Res. 2000;12:412–414.
- Ajaiyeoba EO, Ekundaya O, Okogun JI, et al Antifungal agents: syntheses and antifungal activities of 2-phenylnitroethane; a component of Dennettia tripetala fruits and nitro vinyl derivatives. J Pharm Res Develop. 1999;4:47–52.
- Naranjo P, Kijjoa A, Giesbrecht AM, et al Ocotea quixos, American cinnamon. J Ethnopharmacol. 1981;4:233–236.
- Pott MB, Pichersky E, Piechulla B. Characterization of an acyltransferase capable of synthesizing benzyl benzoate and other volatile esters in flowers and damaged leaves of Clarkia breweri. J Plant Physiol. 2002;159:925–934.
- Kaiser R, Lamparsky D. Constituants azotes en trace de quelques absolues de fleurs et leurs headspaces correspondants. In: Grasse F, editor. Proceedings of 8th International Congrress of Essential Oils; (Cannes, 1980); Fedarom, Grasse, France 1982. p. 287–294.
- Kaiser R. The scents of orchids, olfactory and chemical investigations. Amsterdam: Elsevier;1993. p. 259. ISBN10:0444898417/ISBN 13: 9780444898418.
- Stashenko EE, Prada NQ, Martínez JR. HRGC/FID/NPD and HRGC/MSD study of Colombian Ylang-Ylang (Cananga odorata) oils obtained by different extraction techniques. J High Resol Chromatogr. 1996;19:353–358.
- Rg B, Teranishi R, Lc L, et al Quantitative and sensory studies on tomato paste volatiles. J Agric Food Chem. 1990;36:336–340.
- Kuwahara Y, Tanabe T, Asano Y. Changes in defense allomone compositions of Eutrichodesmus legans and Eutrichodesmus armatus (Polydesmida: haplodesmidae)during different stages of their life cycles. Appl Entomol Zool. 2017;52:305–312.
- Matsuo M, Kirkland DF, Underhill EW. 1-Nitro-2-phenylethane, a possible intermediate in the biosynthesis of benzylglucosinolate. Phytochem. 1972;11:697–701.
- Koeduka T, Kajiyama M, Suzuki H, et al Benzenoid biosynthesis in the flowers of Eriobotrya japonica: molecular cloning and functional characterization of p-methoxybenzoic acid carboxyl methyltransferase. Planta. 2016;244:725–736.
- Kuwahara Y, Shimizu N, Tanabe T. Release of hydrogen cyanide via a post-secretion Schotten-Baumann reaction in defensive fluids of polydesmoid millipedes. J Chem Ecol. 2011;37:232–238.
- Kuwahara Y, Mori N, Sakuma M, et al (1Z)- and (1E)-2-Nitroethenylbenzenes, and 2-nitroethylbenzene as natural products in defense secretions of a millipede Thelodesmus armatus Miyosi (Polydesmida: pyrgodesmidae). Jpn J Environ Entomol Zool. 2003;14:149–155.
- McKenna R, Nielsen DR. Styrene biosynthesis from glucose by engineered E. coli. Metab Eng. 2011;13:544–554.
- Yamaguchi T, Kuwahara Y, Asano Y. A novel cytochrome P450, CYP3201B1, is involved in (R)-mandelonitrile biosynthesis in a cyanogenic millipede. FEBS Open Bio. 2017. DOI:10.1002/2211-5463.12170
- Ueatrongchit T, Kayo A, Komeda H, et al Purification and characterization of a novel R-hydroxynitrile lyase from Eriobotrya japonica (Loquat). Biosci Biotech Biochem. 2008;72:1513–1522.
- Vilegas JH, Lanças FM, Vilegas W. Composition of the volatile compounds from Aniba canelilla (HBK) Mez. extracted by CO2 in the supercritical state. Rev bras farmacogn. 1998;7:13–19.
- Gbolade AA, Arcoraci T, D’Arrigo M, et al Essential oils of Dennettia tripetala Bak. f. stem bark and leaf. Constituents and biological activities. Planta Med. 2009;75(09):PI32.
- Kuwahara Y, Ômura H, Tanabe T. 2-Nitroethenylbenzene as natural products in millipede defense secretions. Naturwissenschaften. 2002;89:308–310.
- El-Sayed AM. (2017). The pherobase: database of pheromones and semiochemicals. Available from: http://www.pherobase.com
- Buttery RG. Quantitative and sensory aspects of flavor of tomato and other vegetables and fruits. In: Acree T, Teranishi R, editor. Flavor Science: sensible principles and techniques,”. Washington, DC, USA: ACS; 1993. p. 259–286.
- Noge K, Tamogami S. Isovaleronitrile co-induced with its precursor, L-leusine, by herbivory in the common evening primrose stimulates foraging behavior of the predatory blue shield bug. Biosci Biotech Biochem. 2018;82:395–406.
- Knudsen JT, Tollsten L, Bergstrom LG. Floral scents—a checklist of volatile compounds isolated by head-space techniques. Phytochemistry. 1993;33:253–280.
- Imai T, Maekawa M, Tsuchiya S. Attractiveness of p-anisaldehyde to the varied carpet beetle, Anthrenus verbasci (L.)(Coleoptera: dermestidae). Appl Entomol Zool. 2002;37:505–508.