ABSTRACT
Rice blast caused by Pyricularia oryzae (syn. Magnaporthe oryzae) is a disease devastating to rice. We have studied the Arabidopsis-P. oryzae pathosystem as a model system for nonhost resistance (NHR) and found that SOBIR1, but not BAK1, is a positive regulator of NHR to P. oryzae in Arabidopsis. AGB1 is also involved in NHR. However, the genetic interactions between SOBIR1, BAK1, and AGB1 are uncharacterized. In this study, we delineated the genetic interactions between SOBIR1, BAK1, and AGB1 in NHR to P. oryzae in Arabidopsis and found SOBIR1 and AGB1 independently control NHR to P. oryzae in Arabidopsis pen2-1 mutant plants. Furthermore, XLG2, but not TMM, has a positive role in penetration resistance to P. oryzae in Arabidopsis pen2-1 mutant plants. Our study characterized genetic interactions in Arabidopsis NHR.
Abbreviations: PRR: pattern recognition receptor, RLK: receptor-like kinase, RLP: receptor-like protein, BAK1: BRASSINOSTEROID INSENSITIVE 1-ASSOCIATED RECEPTOR KINASE 1, BIR1: BAK1-INTERACTING RECEPTOR-LIKE KINASE 1, SOBIR1: SUPPRESSOR OF BIR1-1-1, AGB1: ARABIDOPSIS G PROTEIN ß-SUBUNIT 1, XLG2: EXTRA-LARGE G PROTEIN 2
GRAPHICAL ABSTRACT

SOBIR1 and AGB1 independently control NHR to P. oryzae in Arabidopsis pen2-1 mutant plants.
Plants have immune systems to protect against pathogens. Plants use pattern recognition receptors (PRRs) to detect pathogen-associated molecular patterns (PAMPs). Plant PRRs consist of receptor-like kinases (RLKs) and receptor-like proteins (RLPs) that contain ligand-binding ectodomains recognizing specific PAMPs [Citation1,Citation2] . RLKs interact with co-receptors, including BRI1-ASSOCIATED RECEPTOR KINASE 1 (BAK1) and BAK1-LIKE 1 (BKK1), in a ligand-dependent manner to initiate resistance responses [Citation3]. Arabidopsis BAK1 was identified as a protein interacting with RLK BRASSINOSTEROID INSENSITIVE 1 (BRI1) and functions together with BRI1 to recognize brassinosteroids [Citation4]. BAK1 also plays a key role in plant immunity, where it functions as an RLK FLAGELLIN-SENSING 2 (FLS2) and ELONGATION FACTOR-TU RECEPTOR (EFR) co-receptor [Citation5]. Arabidopsis bak1 mutant plants display necrosis after challenge with necrotrophic pathogens [Citation6]. Furthermore, loss-of-function of BAK1 and its close homolog BKK1 leads to cell death and immunity in Arabidopsis [Citation7,Citation8]. Therefore, BAK1 controls cell death and defense responses as a negative regulator. Arabidopsis BAK1-INTERACTING RECEPTOR-LIKE KINASE 1 (BIR1) also controls cell death as a negative regulator, where a bir1-1 mutant experiences activation of cell death [Citation9]. SUPPRESSOR OF BIR1 (SOBIR1) was identified from a suppressor screen of bir1-1 [Citation9]. Mutations in SOBIR1 partially suppress the cell death phenotype observed in bir1-1 mutant plants. Furthermore, BAK1 was identified as SOBIR7 in a suppressor screen in bir1-1 and found to be required for cell death in bir1-1 mutant plants, suggesting that BAK1 functions as a positive regulator of cell death in bir1-1 mutant plants [Citation10]. Due to lacking cytoplasmic domains, RLPs are thought to depend on another co-receptor, SOBIR1, to mediate signal transmission [Citation11]. Arabidopsis SOBIR1 is involved in RLP-dependent signal transduction, including for RLP1, RLP23, RLP30, and RLP42 [Citation12–Citation16]. Recent reports indicate that BAK1 functions in RLP-mediated plant immunity [Citation1,Citation3]. Furthermore, BAK1 is recruited into a tripartite complex in a ligand-dependent manner [Citation16,Citation17]. Therefore, BAKs and SOBIR1 function as co-receptors to control RLK- or RLP-signaling networks and regulate plant immunity [Citation1,Citation3].
The Arabidopsis RLK ERECTA regulates both developmental processes and immune responses [Citation18]. ERECTA controls stomatal development through ER-like 1 (ERL1), ERL2, and RLP TOO MANY MOUTHS (TMM) interactions [Citation19,Citation20]. ERECTA functions as a positive regulator of immunity to bacteria, e.g., Ralstonia solanacearum [Citation21], fungi, e.g., Plectosphaerella cucumerina and Verticillium longisporum [Citation22,Citation23], and oomycetes, e.g., Pythium irregulare [Citation24]. A recent report also showed that ERECTA works with ERL1, ERL2, and TMM to regulate P. cucumerina resistance [Citation25].
Heterotrimeric G proteins act as convergent points for RLK-mediated signaling in plant immunity [Citation26]. In addition, mutation of ARABIDOPSIS G PROTEIN ß-SUBUNIT 1 (AGB1) suppresses the cell death in bir1-1 mutant plants and transgenic plants overexpressing SOBIR1 [Citation26]. This suggests that SOBIR1 and AGB1 are required for cell death in bir1-1 mutant plants and AGB1 functions downstream of SOBIR1 to control cell death in bir1-1 mutant plants. Furthermore, EXTRA-LARGE G PROTEIN 2 (XLG2) was also identified as SOBIR, since loss-of-function of XLG2 suppressed the cell death in bir1-1 mutant plants [Citation27]. AGB1 and XLG2 functions as a positive regulator in plant immunity [Citation26–Citation29].
Rice blast caused by Pyricularia oryzae is a disease devastating to rice. Although the resistance to P. oryzae have been studied massively [Citation30,Citation31], the mechanisms of resistance to P. oryzae in nonhosts remain poorly understood. Nonhost resistance (NHR) is a disease resistance to all genetic variants of a non-adapted pathogen species in an entire plant species [Citation32]. We have found that an Arabidopsis penetration2 (pen2) mutant experiences increased penetration into epidermal cells by P. oryzae [Citation33]. Upon inoculation onto pen2-1 mutant plants, P. oryzae conidia germinate and produce appressoria that attempt to penetrate the epidermal cells, some of which may be successful. This penetration leads to the accumulation of autofluorescent compounds in the challenged epidermal cell and a hypersensitive response-like cell death [Citation33]. PEN2 encodes an atypical myrosinase that metabolizes indolic glucosinolate during defense responses [Citation34–Citation36].
To date, we have determined that AGB1, ERECTA, and SOBIR1, but not BAK1, function as positive regulators in NHR to P. oryzae in Arabidopsis pen2-1 mutants [Citation33,Citation37–Citation39]. Based on being identified as suppressors of bir1-1, recent reports suggest that SOBIR1, BAK1, and AGB1 function in a genetic pathway to control cell death and plant immunity [Citation9,Citation10,Citation26]. AGB1 acts downstream of SOBIR1 in Arabidopsis [Citation26]. However, the genetic interactions between SOBIR1, BAK1, and AGB1 in NHR to P. oryzae in Arabidopsis remain uncharacterized. In addition, recent reports showed that AGB1 forms a functional complex with XLG2, which was also identified as SOBIR, and regulate plant immunity [Citation27–Citation29]. Furthermore, ERECTA works with RLP TMM to regulate plant immunity [Citation25] and SOBIR1 functions as an RLP co-receptor [Citation11]. From these results, XLG2 and RLP TMM might be involved in NHR to P. oryzae in Arabidopsis. However, the function of XLG2 and RLP TMM in NHR to P. oryzae in Arabidopsis remain uncharacterized. In the present study, we demonstrate SOBIR1 and AGB1 independently control NHR to P. oryzae in Arabidopsis pen2-1 mutant plants. The genetic interactions between SOBIR1 and BAK1 in the penetration resistance of pen2-1 mutant plants were determined to be complex. Furthermore, XLG2, but not RLP TMM, was found to function as a positive regulator of penetration resistance to P. oryzae in Arabidopsis pen2-1 mutant plants.
Materials and methods
Plant materials and growth conditions
The plants used in this study were Col-0 (wild-type), pen2-1 [Citation34], agb1-2 (SALK_061896 [Citation40]), bak1-4 (SALK_116202 [Citation6]), sobir1-12 (SALK_050715 [Citation14]), tmm-1 [Citation41], xlg2-1 (SALK_062645 [Citation28]), and xlg3-2 (SALK_030162 [Citation28]), which were all on the Col-0 background. These mutants were used in crosses to generate double and triple mutants as previously described [Citation33,Citation37]. Arabidopsis plants were grown on Murashige and Skoog plates under short-day conditions (9:15 L:D) at 22°C (100 μmol m−2sec−1 fluorescent illumination) in a growth room for 3 weeks. The plants were then transferred to soil and grown under short-day conditions (9:15 L:D) at 22°C (100 μmol m−2sec−1 fluorescent illumination) in a growth room for 4 weeks.
Pathogen inoculation and analysis
P. oryzae isolate Hoku 1 (race 007) was incubated on oatmeal agar media at 25°C and then the inoculum was prepared. To inoculate P. oryzae, 5-μL droplets (4 x 104 spores/mL) were applied to leaves (leaf number 8) of Arabidopsis plants at dawn (1 h before lights off). Leaves were numbered from oldest to youngest. Inoculated plants were then maintained under short-day conditions (9:15 L:D) at 22°C (100 μmol m−2sec−1 fluorescent illumination) in a growth room with saturating humidity until harvested [Citation42].
To quantify cell entry, we examined germinated fungal sporelings that developed appressoria on six leaves from six independent plants per experiment and genotype (minimum of 100 appressoria/leaf evaluated). Successful penetration of P. oryzae was detected based on the detection of autofluorescence or hyphal elongation at infection sites using fluorescence and bright-field microscopy. Cell entry for each plant genotype was quantified in three independent experiments.
Plant height analysis
Plant height was measured for 4-month-old Arabidopsis plants. At least 30 plants per genotype were measured. Plant height was measured in three independent experiments.
Statistical analysis
Data were compared using one-way analysis of variance with Tukey’s highly significant difference test. Calculations were performed on three data sets (n = 3) and differences were considered statistically significant at p < 0.05.
Results
Genetic interactions among SOBIR1, BAK1, and AGB1 in penetration resistance to P. oryzae in Arabidopsis pen2-1 mutant plants
We previously studied the genetic interactions involved in NHR to P. oryzae in Arabidopsis and found that AGB1, ERECTA, and SOBIR1, but not BAK1, are positive regulators of NHR to P. oryzae in Arabidopsis pen2-1 mutant plants [Citation33,Citation37–Citation39]. To elucidate the genetic interactions between these components controlling NHR to P. oryzae in Arabidopsis pen2-1 mutant plants, pen2-1 sobir1-12 agb1-2 and pen2-1 sobir1-12 bak1-4 triple mutants were generated and their NHR response to P. oryzae was analyzed. Analysis of P. oryzae infection of these mutants at 72 h post-inoculation (hpi) showed the entry rate into pen2-1 bak1-4 mutant plants was similar to that into pen2-1 mutant plants, while the entry rates into pen2-1 agb1-2 and pen2-1 sobir1-12 mutant plants were significantly higher than into pen2-1 mutant plants (). These results are consistent with our previous results [Citation33,Citation37,Citation39]. Furthermore, the entry rate into pen2-1 sobir1-12 agb1-2 triple mutant plants was significantly higher than into pen2-1 agb1-2 and pen2-1 sobir1-12 mutant plants and equal to the sum of the entry rates into these double mutant plants (), suggesting an additive effect between SOBIR1 and AGB1 in regulation of penetration resistance to P. oryzae in Arabidopsis pen2-1 mutant plants. As described above, the entry rate into pen2-1 bak1-4 mutant plants was similar to that into pen2-1 mutant plants, suggesting BAK1 does not play a role in penetration resistance to P. oryzae in Arabidopsis pen2-1 mutant plants. However, surprisingly, the entry rate into pen2-1 sobir1-12 bak1-4 triple mutant plants was significantly lower than that into pen2-1 sobir1-12 mutant plants and similar to that into pen2-1 bak1-4 mutant plants (), suggesting a complex genetic interaction between SOBIR1 and BAK1 in penetration resistance to P. oryzae in Arabidopsis pen2-1 mutant plants.
Figure 1. Quantitative analysis of penetration resistance to Pyricularia oryzae in Arabidopsis mutant plants.Mean frequency of P. oryzae penetration into Arabidopsis mutant plants at 72 hours post-inoculation (hpi) expressed as percentage of the total number of infection sites. Values are presented as mean ± standard error, n = 3 independent experiments. Bars with the same lowercase letters are not statistically significantly different (p > 0.05).
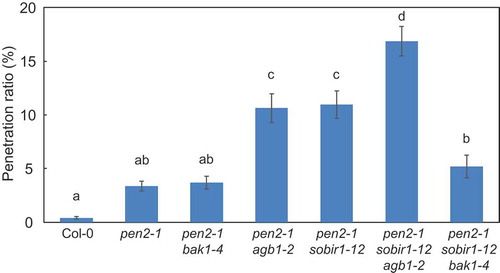
Genetic interactions among SOBIR1, BAK1, and AGB1 in post-penetration resistance to P. oryzae in Arabidopsis pen2-1 mutant plants
Next, we examined post-penetration resistance by measuring the lengths of the longest infection hyphae in the pen2-1 mutant, pen2-1 double mutants (pen2-1 bak1-4, pen2-1 agb1-2, and pen2-1 sobir1-12), and pen2-1 triple mutants (pen2-1 sobir1-12 agb1-2 and pen2-1 sobir1-12 bak1-4) at 72 hpi. Infection hyphae in pen2-1 bak1-4 and pen2-1 agb1-2 mutant plants were slightly longer than in pen2-1 mutant plants, although this difference was not significant. The infection hyphae in pen2-1 sobir1-12 mutant plants were significantly longer than those in pen2-1 mutant plants (), which is consistent with our previous results and suggests that SOBIR1 regulates post-penetration resistance in pen2-1 mutant plants [Citation33,Citation37,Citation39]. Infection hyphae in pen2-1 sobir1-12 agb1-2 mutant plants were significantly longer than those in pen2-1 agb1-2 mutant plants, slightly longer than those in pen2-1 sobir1-12 mutant plants, and equal to the sum of the lengths in these double mutant plants (), suggesting an additive effect between SOBIR1 and AGB1 in the regulation of post-penetration resistance to P. oryzae in pen2-1 mutant plants.
Figure 2. Quantitative analysis of post-penetration resistance to P. oryzae in Arabidopsis mutant plants.
Mean lengths of infection hyphae were measured at 72 hpi. Values are presented as mean ± standard error, n = 3 independent experiments. Bars with the same lowercase letters are not statistically significantly different (p > 0.05).
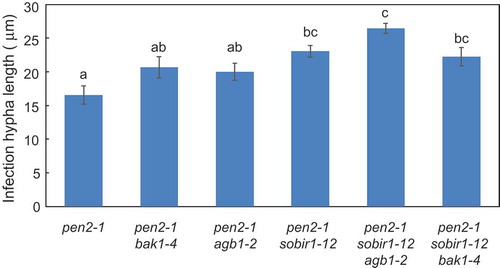
The infection hyphae in pen2-1 sobir1-12 bak1-4 mutant plants were slightly, but not significantly, longer than those in pen2-1 bak1-4 mutant plants and similar to those in pen2-1 sobir1-12 mutant plants (). In contrast to the penetration resistance of pen2-1 sobir1-12 bak1-4 mutant plants, these results suggest there is no genetic interaction between SOBIR1 and BAK1 in pen2-1 mutant plants and indicate that BAK1 does not contribute to post-penetration resistance to P. oryzae in pen2-1 sobir1-12 mutant plants.
We also examined the number of hyphal branches in these mutants. The number of hyphal branches in pen2-1 double mutants (pen2-1 bak1-4, pen2-1 agb1-2, and pen2-1 sobir1-12) was slightly higher than in pen2-1 mutant plants, although this difference was not significant (). These results are consistent with our previous results [Citation37,Citation39]. There were significantly more hyphal branches in pen2-1 sobir1-12 agb1-2 mutant plants than pen2-1 mutant plants and slightly, but not significantly, more than in pen2-1 agb1-2 and pen2-1 sobir1-12 mutant plants. The number of branches was equal to the sum of the numbers in these double mutant plants (), suggesting an additive effect between SOBIR1 and AGB1 in regulation of post-penetration resistance to P. oryzae in pen2-1 mutant plants. The number of hyphal branches in pen2-1 sobir1-12 bak1-4 mutant plants was the same as in pen2-1 mutant plants (), suggesting BAK1 does not play a role in post-penetration resistance to P. oryzae in pen2-1 sobir1-12 mutant plants.
Figure 3. Quantitative analysis of number of hyphal branches of P. oryzae in Arabidopsis mutant plants.
Number of P. oryzae hyphal branches of P. oryzae was measured at 72 hpi. Values are presented as mean ± standard error, n = 3 independent experiments. Bars with the same lowercase letters are not statistically significantly different (p > 0.05).
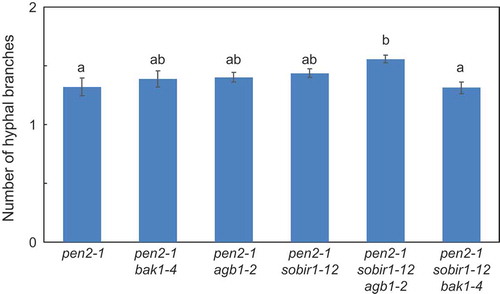
Genetic interactions among SOBIR1, BAK1, and AGB1 in regulation of Arabidopsis pen2-1 mutant plant height
AGB1, BAK1 and SOBIR1 are involved in plant growth and development [Citation4,Citation25,Citation40,Citation43]. To investigate the effect of these components on plant growth in the pen2-1 background, we examined the heights of the pen2-1 multiple mutant plants. The pen2-1 mutant plants were slightly, but not significantly, shorter than wild-type plants (Col-0). Meanwhile, pen2-1 bak1-4 and pen2-1 sobir1-12 mutant plants were of similar height to pen2-1 mutant plants (). These results suggest that BAK1 and SOBIR1 do not have roles in plant height regulation in pen2-1 mutant plants. However, pen2-1 agb1-2 mutant plants were significantly shorter than pen2-1 mutant plants (), suggesting AGB1 is a positive regulator of plant height in pen2-1 mutant plants. In fact, AGB1 functions as a positive regulator of plant height in Arabidopsis [Citation40]. The pen2-1 sobir1-12 agb1-2 mutant plants were significantly shorter than pen2-1 sobir1-12 mutant plants and of similar height to pen2-1 agb1-2 mutant plants (), suggesting SOBIR1 does not play a role in plant height regulation in pen2-1 agb1-2 mutant plants. However, the pen2-1 sobir1-12 bak1-4 plants were significantly shorter than pen2-1 sobir1-12 mutant plants and slightly shorter than pen2-1 bak1-4 plants (), indicating SOBIR1 and BAK1 had synergistic effects on plant height in the pen2-1 background.
Figure 4. Quantitative analysis of Arabidopsis mutant plant height.
Plant height was measured in 4-month-old Arabidopsis mutant plants for at least 30 plants per genotype. Values are presented as mean ± standard error, n = 3 independent experiments. Bars with the same lowercase letters are not statistically significantly different (p > 0.05).

TMM is not involved in penetration resistance to P. oryzae in pen2-1 mutant plants
Previously, we reported ERECTA is a positive regulator of NHR in Arabidopsis [Citation38]. ERECTA regulates both developmental processes and immune responses in Arabidopsis [Citation18]. It has been shown that RLP TMM is an indispensable component of the ERECTA complex in regulation during stomatal development [Citation19,Citation20]. Recently, it has been shown RLP TMM is also involved in ERECTA-mediated defense signaling against P. cucumerina [Citation25]. To determine whether TMM affects NHR to P. oryzae in Arabidopsis, a tmm-1 mutant was inoculated with P. oryzae and examined by microscopy. Analysis of the tmm-1 mutant at 72 hpi showed the entry rate into tmm-1 mutant plants was similar to that into Col-0 wild-type plants (). Next, a double mutant was produced for pen2-1 and tmm-1 to examine the role of TMM in NHR to P. oryzae in pen2-1 mutant plants. The entry rate into pen2-1 tmm-1 mutant plants was slightly, but not significantly, higher than into pen2-1 mutant plants (), suggesting TMM does not play a major role in NHR to P. oryzae in pen2-1 mutant plants.
Figure 5. Quantitative analysis of penetration resistance to P. oryzae in Arabidopsis mutant plants.
Mean frequency of P. oryzae penetration into Arabidopsis mutant plants at 72 hpi expressed as percentage of total number of infection sites. Values are presented as mean ± standard error, n = 3 independent experiments. Bars with the same lowercase letters are not statistically significantly different (p > 0.05).
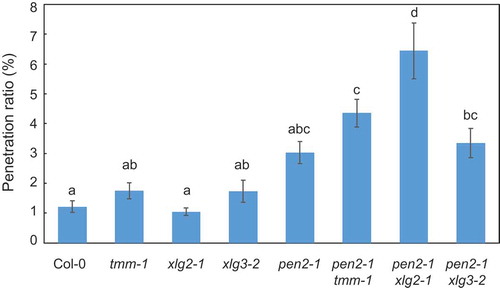
XLG2 is involved in penetration resistance to P. oryzae in pen2-1 mutant plants
Heterotrimeric G proteins function in plant immunity against diverse pathogens. XLG2 and XLG3 were recently reported to play an important role in plant immunity. XLG2 forms a functional complex with AGB1 and regulate plant immunity [Citation27–Citation29]. To determine whether XLG2 and XLG3 affect NHR to P. oryzae in Arabidopsis, xlg2-1 and xlg3-2 mutant plants were inoculated with P. oryzae and examined by microscopy. Analysis at 72 hpi showed the entry rates into the xlg mutants were similar to those for Col-0 wild-type plants (). Next, a double mutant was produced between pen2-1 and xlg mutants to examine the role of XLG2 and XLG3 in NHR to P. oryzae in pen2-1 mutant plants. The entry rate into pen2-1 xlg2-1 mutant plants was significantly higher than into pen2-1 mutant plants. However, the entry rate into pen2-1 xlg3-2 mutant plants was slightly, but not significantly, higher than into pen2-1 mutant plants (). These results suggest XLG2, but not XLG3, is involved in penetration resistance to P. oryzae in Arabidopsis pen2-1 mutant plants.
Next, we examined the role of XLG2 in post-penetration resistance by measuring the lengths of the longest infection hyphae in pen2-1 xlg2-1 mutant plants at 72 hpi. The infection hyphae in pen2-1 xlg2-1 mutant plants were slightly, although not significantly, longer than those in pen2-1 mutant plants (). We also investigated the number of hyphal branches in pen2-1 xlg2-1 mutant plants. The number of hyphal branches in pen2-1 xlg2-1 mutant plants was similar to that in pen2-1 mutant plants (), suggesting XLG2 is not involved in post-penetration resistance to P. oryzae in pen2-1 mutant plants.
Figure 6. Quantitative analysis of post-penetration resistance to P. oryzae in pen2-1 xlg2-1 mutant plants.
A, Mean lengths of infection hyphae measured at 72 hpi. Values are presented as mean ± standard error, n = 3 independent experiments. Bars with the same lowercase letters are not statistically significantly different (p > 0.05).B, Number of P. oryzae hyphal branches at 72 hpi. Values are presented as mean ± standard error, n = 3 independent experiments. Bars with the same lowercase letters are not statistically significantly different (p > 0.05).
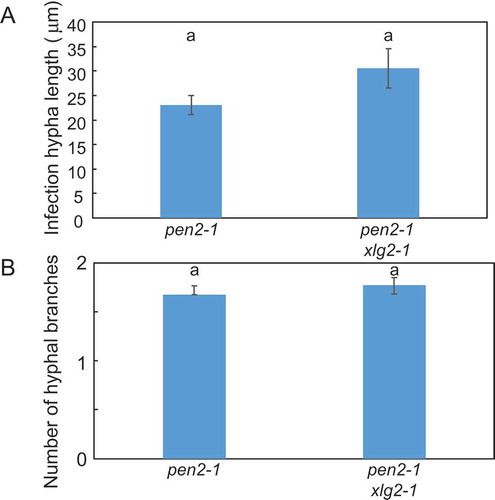
Discussion
In the present study, genetic analyses of multiple mutant combinations involving pen2-1 allowed characterization of genetic interactions between SOBIR1, BAK1, and AGB1 in NHR to P. oryzae in Arabidopsis. In addition, we showed that XLG2, but not RLP TMM, controls penetration resistance to P. oryzae in pen2-1 mutant plants.
In the pen2-1 sobir1-12 agb1-2 triple mutant plants, penetration resistance to P. oryzae was severely compromised and there was more growth of infection hyphae in penetrated epidermal cells than in the pen2-1 double mutants (pen2-1 agb1-2 and pen2-1 sobir1-12) (, , and ). These results suggest SOBIR1 and AGB1 had additive effects on penetration and post-penetration resistance to P. oryzae in pen2-1 mutant plants. Therefore, SOBIR1 and AGB1 function in parallel as positive regulators of penetration and post-penetration resistance in pen2-1 mutant plants. By contrast, in a recent report, agb1-2 was identified as a suppressor of bir1-1, a mutant that activates cell death and immunity in Arabidopsis. The cell death and immunity in bir1-1 mutant plants and transgenic plants overexpressing SOBIR1 are suppressed by an agb1 mutation [Citation26]. This suggests AGB1 controls cell death and immunity as a positive regulator and acts downstream of SOBIR1. Taken together, the genetic interactions between SOBIR1 and AGB1 that regulate NHR in pen2-1 mutant plants and cell death responses in bir1-1 mutant plants are distinct.
In this report, we found that there is a complex genetic interaction between SOBIR1 and BAK1 during penetration resistance, where BAK1 may function as a negative regulator (). By contrast, we also showed that SOBIR1 and BAK1 function synergistically as positive regulators of plant height (). Therefore, our data indicate that BAK1 could function as a negative or positive regulator in some Arabidopsis processes. A recent report showed loss-of-function of BAK1 and its close homolog BKK1 leads to cell death and immunity [Citation7,Citation8], suggesting that BAK1 controls cell death and immunity as a negative regulator. By contrast, overexpression of BAK1 induces cell death and bak1 is a suppressor of cell death in bir1-1 mutant plants [Citation10,Citation44], showing that BAK1 controls cell death as a positive regulator in Arabidopsis. Therefore, BAK1 can control plant immunity as either a negative or positive regulator in Arabidopsis. BAK1 functions as a co-receptor for PRRs [Citation3]. Therefore, PRRs, through interactions with BAK1, could regulate the reciprocal functions of BAK1. Interesting future work includes identifying these PRRs. In addition, SOBIR1, but not BAK1, was shown to function as a positive regulator in post-penetration resistance in pen2-1 mutant plants ( and ), suggesting the PRR/SOBIR1 complex, but not the PRR/BAK1 complex, regulates post-penetration resistance in pen2-1 mutant plants.
Our genetic analyses of pen2-1 multiple mutants found that AGB1 plays a major role in the regulation of plant height (). Furthermore, BAK1 and SOBIR1 have a synergistic genetic interaction and function redundantly as positive regulators of plant height in pen2-1 mutant plants (). This genetic interaction is distinct from that involved in penetration resistance in pen2-1 mutant plants, suggesting BAK1 and SOBIR1 function in different signaling complexes to regulate NHR and plant height in Arabidopsis.
RLK ERECTA regulates both developmental processes and immune responses in Arabidopsis [Citation18]. ERECTA controls stomatal development with ERL1, ERL2, and RLP TMM [Citation19,Citation20]. Recently, it was shown that ERL1, ERL2, and TMM are also involved in ERECTA-mediated defense responses against P. cucumerina [Citation25]. Previously, we reported that ERECTA functions as a positive regulator in NHR to P. oryzae in Arabidopsis pen2-1 mutant plants [Citation38]. In this study, our analyses of tmm-1 and pen2-1 tmm-1 mutant plants revealed TMM may be a slight contributor to penetration resistance to P. oryzae, but does not play a major role in pen2-1 mutant plants (). This is in contrast to resistance to P. cucumerina [Citation25], suggesting ERECTA might interact with RLPs besides TMM in NHR to P. oryzae in Arabidopsis.
Recent reports have shown AGB1 and XLG2 regulate plant immunity [Citation26–Citation29]. Previously, we reported that AGB1 functions as a positive regulator in NHR to P. oryzae in Arabidopsis as shown in [Citation33,Citation37]. In this report, we characterized the function of XLG2 in NHR to P. oryzae in Arabidopsis. Analyses of xlg2-1, xlg3-2, pen2-1 xlg2-1, and pen2-1 xlg3-2 mutant plants revealed that XLG2, but not XLG3, functions as a positive regulator in penetration resistance to P. oryzae in pen2-1 mutant plants (). We also showed that XLG2 does not play a role in post-penetration resistance to P. oryzae in Arabidopsis (). Therefore, XLG2 contributes to penetration resistance, but not post-penetration resistance, in pen2-1 mutant plants. In addition, recent reports showed that XLG2 forms a functional complex with AGB1 in Arabidopsis [Citation27–Citation29]. Taken together, XLG2 and AGB1 may interact with each other and control NHR to P. oryzae in Arabidopsis pen2-1 mutant plants. However, due to the close proximity of AGB1 and XLG2 on chromosome 4, it is difficult to make agb1-2 xlg2-1 mutant plants [Citation45]. Thus, the genetic interaction between AGB1 and XLG2 is still unknown.
The genetic and mechanistic requirements for NHR to P. oryzae in Arabidopsis should be studied in the future. These studies may eventually be used to improve plant resistance to pathogens.
Author contributions
T. Takahashi and T. Murano performed the experiments and analyzed the data. A. Ishikawa designed research and wrote the paper.
Acknowledgments
We acknowledge the Arabidopsis Biological Resource Center for providing mutants seeds. We also thank Dr. H. Koga (Ishikawa Prefectural University) for providing the P. oryzae isolate and Dr. P. Shulze-Lefert (Max Planck Institute for Plant Breeding Research) for pen2-1 seeds.
Disclosure statement
No potential conflict of interest was reported by the authors.
References
- Couto D, Zipfel C. Regulation of pattern recognition receptor signalling in plants. Nat Rev Immunol. 2016;16:537–552.
- Yu X, Feng B, He P, et al. From chaos to harmony: responses and signaling upon microbial Pattern Recognition. Annu Rev Phytopathol. 2017;55:109–137.
- Ma X, Xu G, He P, et al. SERKing coreceptors for receptors. Trends Plant Sci. 2016;21:1017–1033.
- Li J, Wen J, Lease KA, et al. BAK1, an Arabidopsis LRR receptor-like protein kinase, interacts with BRI1 and modulates brassinosteroid signaling. Cell. 2002;110:213–222.
- Chinchilla D, Zipfel C, Robatzek S, et al. A flagellin-induced complex of the receptor FLS2 and BAK1 initiates plant defence. Nature. 2007;448:497–500.
- Kemmerling B, Schwedt A, Rodriguez P, et al. The BRI1-associated kinase 1, BAK1, has a brassinolide-independent role in plant cell-death control. Curr Biol. 2007;17:1116–1122.
- He K, Gou X, Yuan T, et al. BAK1 and BKK1 regulate brassinosteroid-dependent growth and brassinosteroid-independent cell-death pathways. Curr Biol. 2007;17:1109–1115.
- Roux M, Schwessinger B, Albrecht C, et al. The Arabidopsis leucine-rich repeat receptor-like kinases BAK1/SERK3 and BKK1/SERK4 are required for innate immunity to hemibiotrophic and biotrophic pathogens. Plant Cell. 2011;23:2440–2455.
- Gao M, Wang X, Wang D, et al. Regulation of cell death and innate immunity by two receptor-like kinases in Arabidopsis. Cell Host Microbe. 2009;6:34–44.
- Liu Y, Huang X, Li M, et al. Loss-of-function of Arabidopsis receptor-like kinase BIR1 activates cell death and defense responses mediated by BAK1 and SOBIR1. New Phytol. 2016;212:637–645.
- Liebrand TW, van den Burg HA, Joosten MH. Two for all: receptor-associated kinases SOBIR1 and BAK1. Trends Plant Sci. 2014;19:123–132.
- Jehle AK, Lipschis M, Albert M, et al. The receptor-like protein ReMAX of Arabidopsis detects the microbe-associated molecular pattern eMax from Xanthomonas. Plant Cell. 2013;25:2330–2340.
- Jehle AK, Furst U, Lipschis M, et al. Perception of the novel MAMP eMax from different Xanthomonas species requires the Arabidopsis receptor-like protein ReMAX and the receptor kinase SOBIR. Plant Signal Behav. 2013;8:e27408.
- Zhang W, Fraiture M, Kolb D, et al. Arabidopsis receptor-like protein30 and receptor-like kinase suppressor of BIR1-1/EVERSHED mediate innate immunity to necrotrophic fungi. Plant Cell. 2013;25:4227–4241.
- Zhang L, Kars I, Essenstam B, et al. Fungal endopolygalacturonases are recognized as microbe-associated molecular patterns by the arabidopsis receptor-like protein RESPONSIVENESS TO BOTRYTIS POLYGALACTURONASES1. Plant Physiol. 2014;164:352–364.
- Albert I, Böhm H, Albert M, et al. An RLP23–SOBIR1–BAK1 complex mediates NLP-triggered immunity. Nature Plants. 2015;1:15140.
- Postma J, Liebrand TW, Bi G, et al. Avr4 promotes Cf-4 receptor-like protein association with the BAK1/SERK3 receptor-like kinase to initiate receptor endocytosis and plant immunity. New Phytol. 2016;210:627–642.
- van Zanten M, Snoek LB, Proveniers MC, et al. The many functions of ERECTA. Trends Plant Sci. 2009;14:214–218.
- Shpak ED, McAbee JM, Pillitteri LJ, et al. Stomatal patterning and differentiation by synergistic interactions of receptor kinases. Science. 2005;309:290–293.
- Lee JS, Hnilova M, Maes M, et al. Competitive binding of antagonistic peptides fine-tunes stomatal patterning. Nature. 2015;522:439–443.
- Godiard L, Sauviac L, Torii KU, et al. ERECTA, an LRR receptor-like kinase protein controlling development pleiotropically affects resistance to bacterial wilt. Plant J. 2003;36:353–365.
- Llorente F, Alonso-Blanco C, Sanchez-Rodriguez C, et al. ERECTA receptor-like kinase and heterotrimeric G protein from Arabidopsis are required for resistance to the necrotrophic fungus Plectosphaerella cucumerina. Plant J. 2005;43:165–180.
- Haffner E, Karlovsky P, Splivallo R, et al. ERECTA, salicylic acid, abscisic acid, and jasmonic acid modulate quantitative disease resistance of Arabidopsis thaliana to VerticilliumLongisporum. BMC Plant Biol. 2014;14:85.
- Ba A, Perez-Perez J, Perez-Perez MM, et al. ABA is an essential signal for plant resistance to pathogens affecting JA biosynthesis and the activation of defenses in Arabidopsis. Plant Cell. 2007;19:1665–1681.
- Jorda L, Sopena-Torres S, Escudero V, et al. ERECTA and BAK1 receptor like kinases interact to regulate immune responses in Arabidopsis. Front Plant Sci. 2016;7:897.
- Liu J, Ding P, Sun T, et al. Heterotrimeric G proteins serve as a converging point in plant defense signaling activated by multiple receptor-like kinases. Plant Physiol. 2013;161:2146–2158.
- Maruta N, Trusov Y, Brenya E, et al. Membrane-localized extra-large G proteins and Gbg of the heterotrimeric G proteins form functional complexes engaged in plant immunity in Arabidopsis. Plant Physiol. 2015;167:1004–1016.
- Zhu H, Gj L, Ding L, et al. Arabidopsis extra large G-protein 2 (XLG2) interacts with the Gbeta subunit of heterotrimeric G protein and functions in disease resistance. Mol Plant. 2009;2:513–525.
- Liang X, Ding P, Lian K, et al. Arabidopsis heterotrimeric G proteins regulate immunity by directly coupling to the FLS2 receptor. Elife. 2016;5:e13568.
- Koga H. Cytological Aspects of Infection by the Rice Blast Fungus Pyricularia Oryzae. Netherlands: Springer; 2001.
- Ebbole DJ. Magnaporthe as a model for understanding host-pathogen interactions. Annu Rev Phytopathol. 2007;45:437–456.
- Lipka U, Fuchs R, Lipka V. Arabidopsis non-host resistance to powdery mildews. Curr Opin Plant Biol. 2008;11:404–411.
- Maeda K, Houjyou Y, Komatsu T, et al. AGB1 and PMR5 contribute to PEN2-mediated preinvasion resistance to Magnaporthe oryzae in Arabidopsis thaliana. Mol Plant Microbe Interact. 2009;22:1331–1340.
- Lipka V, Dittgen J, Bednarek P, et al. Pre- and postinvasion defenses both contribute to nonhost resistance in Arabidopsis. Science. 2005;310:1180–1183.
- Bednarek P, Pislewska-Bednarek M, Svatos A, et al. A glucosinolate metabolism pathway in living plant cells mediates broad-spectrum antifungal defense. Science. 2009;323:101–106.
- Clay NK, Adio AM, Denoux C, et al. Glucosinolate metabolites required for an Arabidopsis innate immune response. Science. 2009;323:95–101.
- Nakao M, Nakamura R, Kita K, et al. Non-host resistance to penetration and hyphal growth of Magnaporthe oryzae in Arabidopsis. Sci Rep. 2011;1:171.
- Takahashi T, Shibuya H, Ishikawa A. ERECTA contributes to non-host resistance to Magnaporthe oryzae in Arabidopsis. Biosci Biotechnol Biochem. 2016;80:1390–1392.
- Takahashi T, Shibuya H, Ishikawa A. SOBIR1 contributes to non-host resistance to Magnaporthe oryzae in Arabidopsis. Biosci Biotechnol Biochem. 2016;80:1577–1579.
- Ullah H, Chen JG, Temple B, et al. The beta-subunit of the Arabidopsis G protein negatively regulates auxin-induced cell division and affects multiple developmental processes. Plant Cell. 2003;15:393–409.
- Nadeau JA, Sack FD. Control of stomatal distribution on the Arabidopsis leaf surface. Science. 2002;296:1697–1700.
- Yamauchi Y, Makihara M, Ishikawa A. Leaf age and time of inoculation contribute to nonhost resistance to Pyricularia oryzae in Arabidopsis thaliana. Plant Biotechnology. 2017;34:207–210.
- Leslie ME, Lewis MW, Youn JY, et al. The EVERSHED receptor-like kinase modulates floral organ shedding in Arabidopsis. Development. 2010;137:467–476.
- Dominguez-Ferreras A, Kiss-Papp M, Jehle AK, et al. An overdose of the Arabidopsis coreceptor BRASSINOSTEROID INSENSITIVE1-ASSOCIATED RECEPTOR KINASE1 or its ectodomain causes autoimmunity in a SUPPRESSOR OF BIR1-1-Dependent manner. Plant Physiol. 2015;168:1106–1121.
- Chakravorty D, Gookin TE, Milner MJ, et al. Extra-large G proteins expand the repertoire of subunits in Arabidopsis heterotrimeric G protein signaling. Plant Physiol. 2015;169:512–529.