ABSTRACT
Benign prostatic hyperplasia (BPH) is commonly observed in men > 50 years worldwide. Phytotherapy is one of the many treatment options. Sorghum (Sorghum bicolor L.) contains various health-improving phytochemicals with antioxidant and inhibitory activities on cell proliferation, both in vitro and in vivo. To confirm the effects of Donganme sorghum ethyl-acetate extract (DSEE) on BPH, we induced BPH in Spragye–Dawley rats using exogenous testosterone. We measured prostate weight, examined prostrates histopathologically, and analyzed mRNAs associated with male hormones and proteins associated with cell proliferation in the prostate. DSEE inhibited weight gain of the prostate; decreased mRNA expressions of androgen receptor and 5α-reductase II; and improved histopathological symptoms, the protein-expressed ratio of Bax/Bcl-2, and the oxidative status of BPH induced by testosterone in SD rats. Therefore, DSEE may have potential as a preventive or therapeutic agent against BPH.
Graphical Abstract
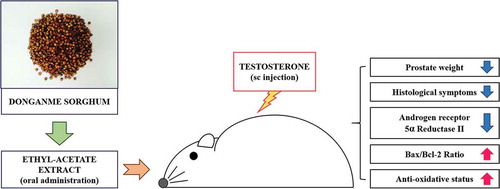
Ameliorative effects of Donganme sorghum ethyl-acetate extract on prostate-weight gain, histological symptoms, mRNA and protein expressions, and anti-oxidative status in SD rats treated with testosterone.
Benign prostatic hyperplasia (BPH) is a histological diagnosis associated with the non-malignant growth of the prostate gland [Citation1]. It is associated with lower urinary tract symptoms, including prostate enlargement and bladder outlet obstruction. BPH gradually increases in an age-dependent manner and is the most common condition affecting men > 50 years of age [Citation2,Citation3]. Although the risk factors and mechanism for the development of BPH remain unclear, its progress is thought to be associated with obesity; low levels of physical activity; and dietary factors, such as a high intake of fat, meat, and dairy products or the low consumptions of vegetables and fruits [Citation4–Citation6]. Only for patients with mild symptoms, the management and treatment of BPH involves watchful waiting and/or lifestyle modifications, including reduction of body weight and increase in exercise [Citation7]. Depending on the level of BPH, medications, such as alpha blockers, 5α-reductase inhibitors, and phosphodiesterase-5 inhibitors, or prostatectomy, may be used [Citation8]. However, in some European countries, phytotherapy using plant extracts is the most commonly recommended initial treatment for men with BPH. A merit of phytotherapy is that it generally has only mild side effects with long-term administration [Citation9]. Epidemiological studies have reported significantly reduced symptoms related to the use of herbal medicines, such as extracts of saw palmetto, nettle, pygeum, rye grass pollen, and others, for BPH [Citation10,Citation11].
Androgen and androgen receptor (AR) signaling play important roles in the development of BPH by promoting the growth of prostate epithelial and stromal cells and enhancing epithelial–mesenchymal transition [Citation12,Citation13]. In patients with BPH, the serum concentra-tion of dihydrotestosterone, a potent agonist to AR, increases, whereas the inhibitors of 5α-reductase II, which convert testosterone to dihydrotestosterone, significantly improve the symptoms of BPH by reducing the size of the prostate [Citation13,Citation14]. It has also been suggested that inflammation and inflammatory mediators are associated with the progression of BPH and that the inflammation may result from damage to the prostate tissue by oxidative stress caused by reactive oxygen species (ROS) [Citation15].
Sorghum (Sorghum bicolor L.) is the fifth most important cereal crop in the world and is a rich source of health-improving phytochemicals, such as phenolic acids, condensed tannins, and phytosterols [Citation16]. Sorghum extract inhibits the Jak/STAT and/or PI3K/Akt pathways and has suppressive and pro-apoptotic effects on tumor growth in the colon, breast, and prostate [Citation17–Citation19]. Sorghum also has outstanding antioxidant properties [Citation16,Citation20].
In this study, we evaluated the preventive or ameliorative potential of Donganme sorghum ethyl-acetate extract (DSEE) on BPH in a testosterone-induced BPH rat model.
Materials and methods
Preparation of DSEE
The preparation and content analysis of DSEE followed the method described previously [Citation19]. Briefly, whole Donganme sorghum was ground, sieved, and extracted with 70% (v/v) ethanol, which was then filtered and concentrated. The concentrate was defatted with n-hexane, and the defatted aqueous layer was extracted three times with ethyl-acetate. The ethyl-acetate layer was then evaporated and freeze-dried.
Ethics statement
Mice were maintained in accordance with the policies of the Chungbuk National University Institutional Animal Care and Use Committee (IACUC). The present study has been approved by the Chungbuk National University IACUC (CBNUA-935–16-01)
Animals
Male Sprague–Dawley (SD) rats aged 7 weeks were purchased from Orientbio Inc. (Seongnam, Korea) and acclimated for 1 week. They were housed in an environmentally controlled animal room at 19°C–23°C and 50% ± 10% relative humidity, with a 12-h light/dark cycle. All rats were provided with a commercial rodent pellet diet and sterile water ad libitum throughout the experimental period.
Experimental design
After acclimatization, animals were randomly assigned into five groups, eight animals per group, as follows: (i) normal control group, (ii) BPH control group, (iii) BPH + DSEE 50 mg/kg group, (iv) BPH + DSEE 100 mg/kg group, and (v) BPH + DSEE 150 mg/kg group. The animals in the BPH groups were subcutaneously injected with 3 mg/kg testosterone (Tokyo Chemical Industry Co., Ltd., Tokyo, Japan) dissolved in olive oil (Sigma-Aldrich Co., St. Louis, MO, USA) 5 days a week for 2 weeks, whereas the normal control group was only injected with olive oil. The doses of DSEE were dissolved in Kolliphor® EL (Sigma-Aldrich Co.) 5% (w/v) solution (vehicle) and administered by oral gavage once a day for 14 days; the normal control group was only administrated with vehicle. At the end of the experimental period, all rats were euthanatized using CO2 gas. Thereafter, all prostates were removed and preserved in 10% neutral buffered formalin for histopathological examination. They were then frozen at <−70°C for further analysis.
Absolute and relative prostate weights
The ventral lobes of the prostate were separated before preserving the prostate tissues. The absolute weights of the prostate ventral lobes were then measured, and the relative weights were calculated as the ratio of the prostate weight to the animal’s body weight.
Histopathological examination
The fixed prostate (ventral lobe) tissues were trimmed, processed, embedded in paraffin, and sectioned in 4-μm increments. The sections were stained with haematoxylin and eosin and histopathologically examined using a microscope. Epithelial thicknesses were randomly measured in six epithelia using the ImageJ software (National Institute of Health, Bethesda, MD, USA)
Reverse transcriptase quantitative polymerase chain reaction analysis
Total RNA was isolated from the prostate tissue using TRIzol Reagent (InvitrogenTM, Thermo Fisher Scientific Inc., Waltham, MA, USA) according to the manufacturer’s instructions. Total RNA concentrations were determined by UV absorbance, after which 2 μg of total RNA was reverse transcribed using random primers and High-Capacity cDNA Reverse Transcription Kits (Applied BiosystemsTM, Thermo Fisher Scientific Inc., Waltham, MA, USA). Quantitative PCR was performed using the Power SYBR® Green PCR Master Mix (Applied Bio-systemsTM) using a real-time PCR system (Model 7500, Applied BiosystemsTM). Each PCR program began with uracil-N-glycosylase incubation at 50°C for 2 min, followed by incubation at 95°C for 10 min. This was followed by 40 cycles of denaturation at 95°C for 15 s and annealing and extension at 60°C for 1 min. Data were acquired and analyzed using the 7500 system and SDS software (version 1.3.1.21; Applied BiosystemsTM). Amplification kinetics were recorded in real-time mode as sigmoid process curves for which fluorescence was plotted against the number of amplification cycles. Glyceraldehyde 3-phosphate dehydrogenase (GAPDH) was used as an internal standard to normalize the expression of the target transcripts. The relative ratios of each target gene mRNA to GAPDH mRNA that precisely quantified the expression levels of the target gene were calculated using standard curves. The primer sets are shown in . Data were analyzed using the comparative Ct method [Citation21].
Table 1. Primers used for quantitative real-time PCR analysis.
Western blot analysis
Prostate tissue was homogenized on ice in RIPA lysis buffer (Thermo Fisher Scientific Inc.) that contained a protease inhibitor and phosphatase inhibitor cocktails (Sigma-Aldrich Co.). The tissue lysates were incubated for approximately 30 min on ice and clarified by centrifugation at 15,300 × g for 10 min at 4°C. The protein content was quantified using a detergent-compatible Bradford assay kit (Thermo Fisher Scientific Inc.). The tissue lysates were separated using 12% sodium dodecyl sulfate (SDS) polyacrylamide gel electrophoresis, and the separated proteins were transferred to a polyvinylidene difluoride membrane (Merck Millipore, Billerica, MA, USA), which was then blocked with 5% non-fat dried milk dissolved in 0.05% Tris-buffered saline containing Tween 20. The membranes were incubated overnight at 4°C with specific primary antibodies for GAPDH, Bax (Cell Signaling Technology Inc., Beverly, MA, USA), and Bcl-2 (Proteintech Group Inc., Rosemont, IL, USA). After washing, the membranes were incubated with secondary anti-rabbit IgG or anti-mouse IgG (Cell Signaling Technology Inc.) for 1 h at room temperature. After washing, the blots were visualized using LuminataTM Crescendo Western HRP substrate (Merck Millipore).
Measurement of 2-thiobarbituric acid reactive substances (TBARS)
Approximately 50 mg of prostate tissue was homogenized with 0.5 mL of phosphate buffered saline at 4°C. Then, 0.25 mL of the homogenate was mixed in a capped vial with 0.25 mL SDS (Sigma-Aldrich Co.) solution (8.1%, w/v), 0.5 mL acetic acid solution (20%, v/v), and 0.25 mL 2-thiobarbituric acid (Sigma-Aldrich Co.) solution (0.75%, w/v). The vials were incubated at 95°C for 30 min and then incubated on ice for 30 min. The mixture was clarified by centrifugation at 15,300 × g for 10 min at 4°C. 1,1,3,3-Tetraethoxypropane (Sigma-Aldrich Co.) solution in various concentrations was used as the standard; it was prepared in the same manner as the prostate homogenate. The optical densities of the sample and standard were measured in a 200-μL aqueous layer using a microplate reader at 520 nm. The TBARS concentration in the sample was calculated from the standard curve and corrected according to the pre-homogenized prostate weight.
Statistical analysis
Data are presented as the mean ± standard error from at least three independent experimental results. The statistical analysis was performed using SPSS version 23 (IBM Corp., Armonk, NY, USA). Statistical significance was assessed using one-way analysis of variance followed by Duncan’s test. Values of p < 0.05 were considered statistically significant.
Results
DSEE suppresses prostate weight gain
illustrates the prostate weight differences between the five groups. The absolute and relative prostate weights in the normal control group were 0.42 ± 0.04 g and 0.12% ± 0.01%, respectively. During exogenous testosterone administration, absolute and relative prostate weights for the BPH control group were significantly increased by 0.54 ± 0.07 g and 0.15% ± 0.02%, respectively. In the 150 mg/kg DSEE administered group, testosterone-induced absolute and relative prostate weight gains (0.44 ± 0.03 g and 0.12 ± 0.01%, respectively) were significantly inhibited compared to those in the BPH control group but were not significantly different from those in the normal control group. The absolute and relative prostate weights in the 50 and 100 mg/kg DSEE-administered groups were 0.49 ± 0.07 g and 0.14 ± 0.02% and 0.49 ± 0.07 g and 0.14 ± 0.02%, respectively.
Figure 1. Donganme sorghum ethyl-acetate extract (DSEE) inhibited the absolute and relative weight gains of the prostate (ventral lobe) in rats treated with testosterone.Values represent the mean ± SE. a and b indicate statistical differences from the groups labeled with different letters (p < 0.05).
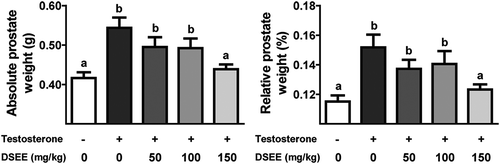
DSEE ameliorates the histopathological symptoms
The histological morphology of prostates in the normal control group showed no abnormal alteration in glandular cell lining (cuboidal or columnar cells of a regular shape and size). In the BPH control group, treatment with exogenous testosterone resulted in significantly increased epithelium folding in the acini, and the lining of the glandular cells thickened and was stratified with hyperplasia. These features were gradually improved by DSEE at 50, 100, and 150 mg/kg.
The average epithelial thickness of the BPH control group was significantly increased by 340.1% ± 147.0% compared with the normal control group (100.0% ± 34.4%). The groups administered with DSEE at 50 and 100 mg/kg were respectively measured by 316.3% ± 135.8% and 274.2% ± 80.7%. Furthermore, the epithelial thickness in the 150 mg/kg DSEE administered group was significantly inhibited by 189.8% ± 104.6% (p < 0.05, ).
Figure 2. Histopathological observations and epithelial thickness of the ameliorative effect of Donganme sorghum ethyl-acetate extract (DSEE) on benign prostatic hyperplasia (BPH) in rats treated with testosterone (hematoxylin and eosin stain). (a) Normal control (NC) group. (b) BPH control group, subcutaneously injected with 3 mg/kg testosterone 5 days a week for 2 weeks. (c) BPH + DSEE 50 mg/kg group. (d) BPH + DSEE 100 mg/kg group. (e) BPH + DSEE 150 mg/kg group. Scale bar indicates 100 μm. Values represent the mean ± SE. a and b indicate statistical differences from the groups labeled with different letters (p < 0.05).
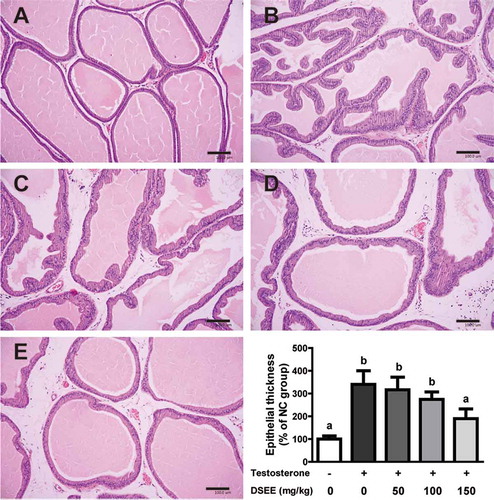
DSEE suppresses mRNA expression associated with testosterone
The mRNA levels of AR and 5α-reductase II in the BPH control group were significantly elevated by 111% and 33%, respectively, compared with those in the normal control group (p < 0.05). The groups administered with DSEE (50, 100, and 150 mg/kg) showed significantly lower levels of AR and 5α-reductase II than the BPH control group (), indicating that DSEE provided protection against the testosterone-mediated elevation of the mRNA expression of these enzymes.
Figure 3. Donganme sorghum ethyl-acetate extract (DSEE) inhibited mRNA expression of androgen receptor and 5α-reductase II in the prostate tissue of rats treated with testosterone.Values represent mean ± SE. a and b indicate statistical differences from the groups labeled with different letters (p < 0.05).
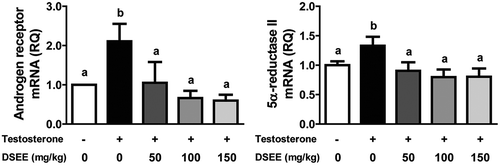
DSEE induces expression of proteins associated with cell proliferation
Compared with the normal control group, the BPH control group showed no significant difference in the expression of Bcl-2 but significant downregulation of Bax protein expression (). The groups administered with DSEE (50, 100, and 150 mg/kg) did not show downregulation of Bax protein expression, indicating that DSEE provided protection against the testosterone-induced decrease in Bax.
Figure 4. Donganme sorghum ethyl-acetate extract (DSEE) improved the ratio of Bax/Bcl-2 and the protein expression of Bax and Bcl-2 in the prostate tissue of rats treated with testosterone.Values represent mean ± SE. a, b, c and d indicate statistical differences from the groups labeled with different letters (p < 0.05).
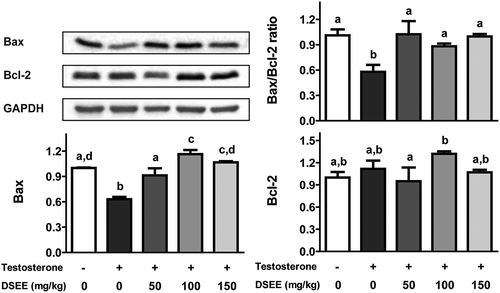
DSEE reduces oxidative stress
Testosterone significantly elevated prostatic TBARS concentration in the BPH control group by 168% compared with that in the normal control group (). Treatment with DSEE significantly reduced this TBARS increase.
Discussion
BPH is characterized by the excessive growth of glandular and stromal tissue in the periurethral zone of the prostate, with hypertrophic basal cells. Compression of the urethra by BPH may lead to urodynamic obstruction [Citation22]. Studies have reported preventive or inhibitory effects of extracts of pomegranate, Pueraria mirifica, and silymarin, which contain various polyphenols, on testosterone-induced BPH in rats [Citation23–Citation25]. Sorghum extract is a rich source of polyphenols and has antidiabetic, anti-inflammatory, and antioxidant properties [Citation20,Citation26,Citation27], which are associated with the prevention and/or therapy for BPH [Citation28,Citation29].
We previously reported the methods and results of the analysis of DSEE on polyphenolic contents and antioxidative property [Citation19]. DSEE contained 33.0 mg total free phenolic acid/g DSEE (by a HPLC performance), 204.39 mg gallic acid equivalents of polyphenol/g DSEE (by the Folin–Ciocalteu assay), and 126.76 mg catechin equivalents of flavonoid/g DSEE (by the modified method of Ghasemzadeh, Jaafar, and Rahmat). For the identification of free phenolic acid, the ethyl acetate fraction was dissolved in methanol and separated by a HPLC systems with a Mightysil RP-18 GP column and in-series UV detection (absorbance at 280 nm). The mobile phases were 0.1% acetic acid in acetonitrile and 0.1% acetic acid in water and the run time was 70 min and flow rate was 1 mL/min. Samples were quantified by comparing retention times within identified standards. Free phenolic acids included chlorogenic acid (11.699 mg/g), catechinic acid (13.267 mg/g), salicylic acid (5.688 mg/g), protocatechiuic acid (0.093 mg/g), ρ-coumaric acid (0.090 mg/g), ferulic acid (0.091 mg/g), vanillic acid (0.122 mg/g), caffeic acid (0.891 mg/g), quercetin (0.462 mg/g), naringin (0.120 mg/g), hesperidin (0.355 mg/g), and naringenin (0.117 mg/g). Furthermore, the radical scavenging activities of DSEE were 705.0 mg ascorbic acid equivalents (AAE)/g DSEE (by the DPPH method) and 335.3 mg AAE/g DSEE (by the ABTS method), respectively [Citation19]. Free phenolic acids such as chlorogenic, vanillic acid, and quercetin were reported to have ameliorative effects on BPH in rats or mice [Citation30–Citation32]. We also confirmed the inhibitory effect of DSEE on the proliferation of PC3M prostate adenocarcinoma cells via the downregulation of cyclins associated with cell cycle progression as well as the induction of apoptosis [Citation19]. The present study therefore investigated the effect of DSEE on testosterone-induced BPH in rats.
In the present study, the absolute and relative prostrate weights of rats treated with testosterone significantly increased compared with those of the normal control group. However, oral administration of 150 mg/kg DSEE for 14 days significantly inhibited this weight increase, and histological examination confirmed amelioration of the pathological changes caused by exogenous testosterone after DSEE treatment. These results suggest that treatment with 150 mg/kg DSEE was effective in protecting against testosterone-induced BPH.
Exogenous testosterone increases the AR and 5α-reductase II expression of prostate tissue in rodents [Citation33,Citation34]. In the present study, treatment with DSEE significantly inhibited the testosterone-mediated increases of AR and 5α-reductase II in prostate tissue. The enzyme 5α-reductase catalyzes dihydrotestosterone from testosterone; 5α-reductase II isozyme is more potent than 5α-reductase I in human prostate tissue [Citation35]. Dihydrotestosterone is a more potent androgen than testosterone because of its higher affinity for AR [Citation36]. Thus, the inhibition of the mRNA expression of AR and 5α-reductase II by DSEE has a potent impact against BPH.
Reportedly, Bax mRNA or protein is downregulated in the prostate tissue of rats treated with testosterone [Citation25,Citation37]. In the present study, the expression of Bax protein was significantly downregulated in rats treated with testosterone compared with the normal control group, but treatment with DSEE (50, 100, and 150 mg/kg) significantly inhibited this downregulation, improving the Bax/Bcl-2 ratio. In this study, although no significant difference was noted, the expression of Bcl-2 in the BPH control group was slightly higher than that in the normal control group. Castration and/or extended period of testosterone-treatment may be required for the significant elevation of Bcl-2 expression because significant increase in its expression has been reported to occur 7 days after castration [Citation38–Citation40]. Furthermore, the expression of Bcl-2 in the ventral lobe has been reported to be lower than that in the other prostate lobes [Citation40].
Oxidative stress promotes BPH [Citation41], and exogenous testosterone increases oxidative stress and reduces the anti-oxidative status in vivo [Citation42]. Biri et al. reported increased TBARS concentrations suggesting oxidative stress and accelerated peroxidative reactions in cancerous prostate tissue [Citation43]. In the present study, treatment with testosterone increased the level of TBARS, but treatment with DSEE significantly ameliorated this increase. Previously, we reported that DSEE contains polyphenols, flavonoids, and various free phenolic acids, which have potent anti-oxidative properties [Citation19]. This may explain why DSEE markedly reduced the increase in TBARS with testosterone.
In conclusion, the findings of the present study demonstrate the effectiveness of DSEE in preventing or ameliorating testosterone-induced BPH in rats. These results may be attributed to the ability of DSEE to decrease the expression of AR and 5α-reductase II, improve the oxidative status, and increase the ratio of Bax/Bcl-2 expression in the prostate. DSEE has the potential to be a preventive or therapeutic agent against human BPH.
Author contributions
JMR, TMK, HSJ, and DJK designed the study. JMR, GYJ, and DP performed the animal and analysis experiments. JMR, GYJ, KSW, DP, and TMK analyzed data. JMR wrote the manuscript. HSJ and DJK revised the manuscript.
Disclosure statement
No potential conflict of interest was reported by the authors.
References
- Roehrborn CG. Benign prostatic hyperplasia: an overview. Rev Urol. 2005;7(Suppl 9):S3–S14. PubMed PMID: 16985902; PubMed Central PMCID: PMCPMC1477638.
- Park HJ, Won JE, Sorsaburu S, et al. Urinary Tract Symptoms (LUTS) secondary to Benign Prostatic Hyperplasia (BPH) and LUTS/BPH with erectile dysfunction in Asian men: a systematic review focusing on tadalafil. World J Mens Health. 2013 Dec;31(3):193–207. PubMed PMID: 24459652; PubMed Central PMCID: PMCPMC3888888.
- Berges R, Hofner K, Gedamke M, et al. Impact of desmopressin on nocturia due to nocturnal polyuria in men with lower urinary tract symptoms suggestive of benign prostatic hyperplasia (LUTS/BPH). World J Urol. 2014 Oct;32(5):1163–1170. PubMed PMID: 25135845.
- Moul S, McVary KT. Lower urinary tract symptoms, obesity and the metabolic syndrome. Curr Opin Urol. 2010 Jan;20(1):7–12. PubMed PMID: 19904208.
- Rohrmann S, Giovannucci E, Willett WC, et al. Fruit and vegetable consumption, intake of micronutrients, and benign prostatic hyperplasia in US men. Am J Clin Nutr. 2007 Feb;85(2):523–529. PubMed PMID: 17284753.
- Parsons JK, Kashefi C. Physical activity, benign prostatic hyperplasia, and lower urinary tract symptoms. Eur Urol. 2008 Jun;53(6):1228–1235. PubMed PMID: 18358592.
- Parsons JK. Modifiable risk factors for benign prostatic hyperplasia and lower urinary tract symptoms: new approaches to old problems. J Urol. 2007 Aug;178(2):395–401. PubMed PMID: 17561143.
- Dimitropoulos K, Gravas S. New therapeutic strategies for the treatment of male lower urinary tract symptoms. Res Rep Urol. 2016;8:51–59. PubMed PMID: 27218069; PubMed Central PMCID: PMCPMC4853157.
- Lowe FC, Ku JC. Phytotherapy in treatment of benign prostatic hyperplasia: a critical review. Urology. 1996 Jul;48(1): 12–20. PubMed PMID: 8693632.
- Curtis Nickel J, Shoskes D, Roehrborn CG, et al. Nutraceuticals in prostate disease: the urologist’s role. Rev Urol. 2008 Summer;10(3):192–206. Pub-Med PMID: 18836556; PubMed Central PMCID: PMCPMC2556486.
- Wilt TJ, Ishani A, Rutks I, et al. Phytotherapy for benign prostatic hyperplasia. Public Health Nutr. 2000 Dec;3(4A):459–472. PubMed PMID: 11276294.
- Roehrborn CG. Pathology of benign prostatic hyperplasia. Int J Impot Res. 2008 Dec;20(Suppl 3):S11–S18. PubMed PMID: 19002119.
- Izumi K, Mizokami A, Lin WJ, et al. Androgen receptor roles in the development of benign prostate hyperplasia. Am J Pathol. 2013 Jun;182(6):1942–1949. PubMed PMID: 23570837; PubMed Central PMCID: PMCPMC3668026.
- Raheem OA, Parsons JK. Associations of obesity, physical activity and diet with benign prostatic hyperplasia and lower urinary tract symptoms. Curr Opin Urol. 2014 Jan;241:10–14. PubMed PMID: 24247174.
- Minciullo PL, Inferrera A, Navarra M, et al. Oxidative stress in benign prostatic hyperplasia: a systematic review. Urol Int. 2015;94(3):249–254.
- de Morais Cardoso L, Pinheiro SS, Martino HSD, et al. Sorghum (Sorghum bicolor L.): Nutrients, bioactive compounds, and potential impact on human health. Crit Rev Food Sci Nutr. 2017;57(2):372–390.
- Darvin P, Joung YH, Nipin S, et al. Sorghum polyphenol suppresses the growth as well as metastasis of colon cancer xenografts through co-targeting jak2/STAT3 and PI3K/Akt/mTOR pathways. J Funct Foods. 2015;15:193–206.
- Park JH, Darvin P, Lim EJ, et al. Hwanggeumchal sorghum induces cell cycle arrest, and suppresses tumor growth and metastasis through Jak2/STAT pathways in breast cancer xenografts. PLoS One. 2012;7(7):e40531.
- Ryu J-M, Jang GY, Woo KS, et al. Effects of sorghum ethyl-acetate extract on PC3M prostate cancer cell tumorigenicity. J Funct Foods. 2017;37:449–459.
- Awika JM, Rooney LW. Sorghum phytochemicals and their potential impact on human health. Phytochemistry. 2004;65(9):1199–1221.
- Livak KJ, Schmittgen TD. Analysis of relative gene expression data using real-time quantitative PCR and the 2− ΔΔCT method. Methods. 2001;25(4):402–408.
- Untergasser G, Madersbacher S, Berger P. Benign prostatic hyperplasia: age-related tissue-remodeling. Exp Gerontol. 2005 Mar;403:121–128. PubMed PMID: 15763388.
- Ammar AE, Esmat A, Hassona MD, et al. The effect of pomegranate fruit extract on testosterone‐induced BPH in rats. Prostate. 2015;75(7):679–692.
- Masrudin S, Mohamad J. Preventive effect of Pueraria mirifica on testosterone‐induced prostatic hyperplasia in Sprague Dawley rats. Andrologia. 2015;47(10):1153–1159.
- Atawia RT, Tadros MG, Khalifa AE, et al. Role of the phytoestrogenic, pro-apoptotic and anti-oxidative properties of silymarin in inhibiting experimental benign prostatic hyperplasia in rats. Toxicol Lett. 2013 May;219(2):160–169. PubMed PMID: 23500659.
- Park JH, Lee SH, Chung I-M, et al. Sorghum extract exerts an anti-diabetic effect by improving insulin sensitivity via PPAR-γ in mice fed a high-fat diet. Nutr Res Pract. 2012;6(4):322–327.
- Shim T-J, Kim TM, Jang KC, et al. Toxicological evaluation and anti-inflammatory activity of a golden gelatinous sorghum bran extract. Biosci Biotechnol Biochem. 2013;77(4):697–705.
- Elkahwaji JE. The role of inflammatory mediators in the development of prostatic hyperplasia and prostate cancer. Res Rep Urol. 2013;5:1.
- Wang Z, Olumi AF. Diabetes, growth hormone-insulin-like growth factor pathways and association to benign prostatic hyperplasia. Differentiation. 2011;82(4–5):261–271.
- Huang Y, Chen H, Zhou X, et al. Inhibition effects of chlorogenic acid on benign prostatic hyperplasia in mice. Eur J Pharmacol. 2017;809:191–195.
- Jung Y, Park J, Kim H-L, et al. Vanillic acid attenuates testosterone-induced benign prostatic hyperplasia in rats and inhibits proliferation of prostatic epithelial cells. Oncotarget. 2017;8(50):87194.
- Pagano E, Laudato M, Griffo M, et al. Phytotherapy of benign prostatic hyperplasia. A minireview. Minireview Phytother Res. 2014;28(7):949–955.
- Takeda H, Nakamoto T, Kokontis J, et al. Autoregulation of androgen receptor expression in rodent prostate: immunohistochemical and in situ hybridization analysis. Biochem Biophys Res Commun. 1991;177(1):488–496.
- Torres JM, Ruiz E, Ortega E. Development of a quantitative RT‐PCR method to study 5α‐reductase mRNA isozymes in rat prostate in different androgen status. Prostate. 2003;56(1):74–79.
- Zhu YS, Imperato‐McGinley JL. 5α‐reductase isozymes and androgen actions in the prostate. Ann NY Acad Sci. 2009;1155(1):43–56.
- Carson C, Rittmaster R. The role of dihydrotestosterone in benign prostatic hyperplasia. Urology. 2003;61(4):2–7.
- Mosli HH, Esmat A, Atawia RT, et al. Metformin attenuates testosterone-induced prostatic hyperplasia in rats: a pharmacological perspective. Sci Rep. 2015 Oct;5:15639. PubMed PMID: 26492952; PubMed Central PMCID: PMCPMC4616049.
- Lee G, Shin J, Choi H, et al. Cynanchum wilfordii ameliorates testosterone-induced benign prostatic hyperplasia by regulating 5α-reductase and androgen receptor activities in a rat model. Nutrients. 2017;9(10):1070.
- Shin SJ, Lee KH, Chung KS, et al. The traditional Korean herbal medicine Ga-Gam-Nai-Go-Hyan suppresses testosterone-induced benign prostatic hyperplasia by regulating inflammatory responses and apoptosis. Exp Ther Med. 2017;13(3):1025–1031.
- Banerjee PP, Banerjee S, Brown TR. Bcl-2 protein expression correlates with cell survival and androgen independence in rat prostatic lobes. Endocrinology. 2002;143(5):1825–1832.
- Vital P, Castro P, Ittmann M. Oxidative stress promotes benign prostatic hyperplasia. Prostate. 2016;76(1):58–67.
- Tothova L, Celec P, Ostatnikova D, et al. Effect of exogenous testosterone on oxidative status of the testes in adult male rats. Andrologia. 2013 Dec;45(6):417–423. PubMed PMID: 23121168.
- Biri H, Öztürk HS, Kaçmaz M, et al. Activities of DNA turnover and free radical metabolizing enzymes in cancerous human prostate tissue. Cancer Invest. 1999;17(5):314–319.