ABSTRACT
A variety of polyphenols have been isolated from plants, and their biological activities have been examined. Sudachitin (5,7,4′-trihydroxy-6,8,3′-trimethoxyflavone) is a polymethoxyflavone that is isolated from the peel of Citrus sudachi. Although we previously reported that sudachitin possesses an anti-inflammatory activity, its other biological activities are not yet understood. In this study, we report a novel biological activity of sudachitin, which selectively induced apoptosis in human keratinocyte HaCaT cells. Another polymethoxyflavone, nobiletin (5,6,7,8,3′,4′-hexamethoxyflavone), promoted autophagy but not apoptosis in HaCaT cells. On the other hand, 3′-demethoxysudachitin (5,7,4′-trihydroxy-6,8-dimethoxyflavone) failed to induce apoptosis and autophagy. These results show that three polymethoxyflavones have different effects on apoptosis and autophagy in HaCaT cells. Understanding the structure and biological activity of polymethoxyflavones may lead to the discovery of potential candidates for cancer drug development without significant toxic side effects.
Abbreviations: ROS: reactive oxygen species; DMSO: dimethyl sulfoxide; MTT: 3-(4, 5-dimethylthiazol-2yl)-2, 5-diphenyltetrazolium bromide; GAPDH: glyceraldehyde-3-phosphate dehydrogenase; PARP: poly(ADP-ribose) polymerase; PI: propidium iodide; MAPK: mitogen-activated protein kinase.
Graphical Abstract
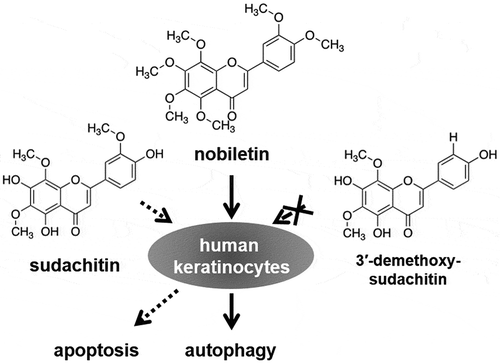
Three polymethoxyflavones have different effects on apoptosis and autophagy in human keratinocytes.
KEYWORDS:
In multicellular organisms, cell death is an important process that maintains tissue homeostasis and eliminates potentially harmful cells [Citation1]. There are various modalities of cell death, including apoptosis (type I), autophagic cell death (type II), and necrosis (type III) [Citation2]. Apoptosis and autophagic cell death are not completely distinct and are affected by each other [Citation3]. Apoptosis usually functions to maintain homeostasis in cell populations in development and aging [Citation4]. It induces morphological changes, such as cell shrinkage, membrane blebbing, chromatin condensation, and nuclear fragmentation, which are almost triggered by activation of the caspase family of cysteine proteases. Apoptosis also plays a pivotal role in eliminating genetically damaged cells that might progress to cancer. Therefore, there have been many trials to develop new compounds that induce apoptosis. On the other hand, autophagy constitutively occurs at low levels to degrade cytoplasmic components including damaged organelles and long-lived proteins, but it is dramatically induced by stresses, such as nutrient starvation and oxidative stress, to promote cell survival [Citation5,Citation6]. It begins with the formation of autophagosomes, followed by their fusion with lysosomes to produce autolysosomes where the engulfed content is digested by lysosomal hydrolases. Although the overactivation of autophagy may result in autophagic cell death, autophagy dysfunction is associated with a number of diseases, including infection, cancer, and neurodegeneration, suggesting that targeting autophagy may be an attractive therapeutic approach.
A wide variety of polyphenols have been identified in many plants, including citrus fruits, cocoa, and tea [Citation7,Citation8]. Several studies have shown that most of them exhibit biological activities, including antioxidant and anticancer effects. Some natural polyphenolic compounds, such as genistein and curcumin, induce apoptosis and autophagy [Citation9]. Polymethoxyflavones, a class of flavonoid compounds that are almost exclusively found in the peel of citrus fruits, also possess various biological activities [Citation10,Citation11]. For example, nobiletin (5,6,7,8,3′,4′-hexamethoxyflavone) (, right), which is a typical polymethoxyflavone from the peel of Citrus depressa and is one of the most studied flavonoids, exhibits anti-inflammatory and carcinogenesis-inhibitory activities [Citation12,Citation13]. Additionally, nobiletin has been reported to induce apoptosis and autophagy in human gastric cancer cells [Citation14]. Other polymethoxyflavones, sudachitin (5,7,4′-trihydroxy-6,8,3′-trimethoxyflavone) (, left) and 3′-demethoxysudachitin (5,7,4′-trihydroxy-6,8-dimethoxyflavone) (, middle), have been isolated from the peel of Citrus sudachi, a well-known fruit in Tokushima Prefecture, Japan [Citation15]. Although we previously reported that sudachitin possesses an anti-inflammatory activity, their other biological activities are not yet fully understood [Citation16].
Figure 1. Effect of sudachitin, 3′-demethoxysudachitin, and nobiletin on cell proliferation of HaCaT cells.
(a) Chemical structure of sudachitin (5,7,4′-trihydroxy-6,8,3′-trimethoxyflavone), 3′-demethoxysudachitin (5,7,4′-trihydroxy-6,8-dimethoxyflavone), and nobiletin (5,6,7,8,3′,4′-hexamethoxyflavone). (b) HaCaT cells were treated with sudachitin (30, 100 μM), 3′-demethoxysudachitin (30, 100 μM), nobiletin (30, 100 μM) or DMSO. After 24 hours (left panel) and 48 hours (right panel), cell proliferation was measured by MTT assay. Results are expressed relative to cells treated with DMSO (= 100%). The data are expressed as the mean ± standard error for at least four cultures, and statistical analysis was performed by Student’s t-test. *** p < 0.001, ** p < 0.01, and * p < 0.05 compared with cells treated with DMSO.
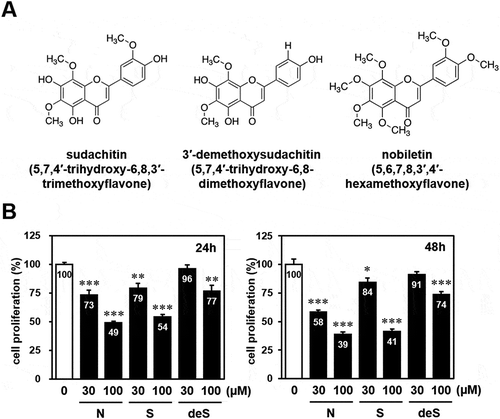
In this study, we attempted to elucidate novel biological activities of sudachitin and 3′-demethoxysudachitin. We found that three polymethoxyflavones including nobiletin suppressed cell proliferation in human keratinocyte HaCaT cells at a high concentration (100 μM). We demonstrated that sudachitin promoted apoptosis in HaCaT cells in a concentration- and time-dependent manner, while nobiletin induced autophagy. These results show that three polymethoxyflavones have different effects on apoptosis and autophagy, and that the marginal structural differences among polymethoxyflavones affect their biological activities.
Materials and methods
Cell culture
Human keratinocyte HaCaT cells were cultured in DMEM supplemented with 10% fetal bovine serum, 100 units/mL penicillin, and 100 μg/mL streptomycin at 37°C in 5% CO2.
Cell proliferation assay
HaCaT cells were seeded at a density of 2 × 103 cells per well in 96-well culture plates. After 24 hours, the cells were treated with 30 or 100 μM sudachitin, 3′-demethoxysudachitin, nobiletin, or dimethyl sulfoxide (DMSO) for 24 and 48 hours. Sudachitin, nobiletin (Wako Pure Chemical Industries), and 3′-demethoxysudachitin [Citation17] were dissolved in 100% DMSO at a concentration of 100 mM, and the final concentration of DMSO in the culture medium was 0.1%. The cell proliferation was assayed by the 3-(4, 5-dimethylthiazol-2yl)-2, 5-diphenyltetrazolium bromide (MTT) assay. After 1-hour incubation with MTT, the resulting formazan crystals were dissolved in DMSO. The absorbance at 570 nm was determined using the Infinite M200 plate reader (TECAN Japan).
Immunoblot analysis
Cells were treated with sudachitin (30, 100 µM), 3′-demethoxysudachitin (30, 100 µM), nobiletin (30, 100 µM), or DMSO for 24 and 48 hours. Then the cells were lysed in an ice-cold TNE buffer consisting of 20 mM Tris-HCl (pH 7.5), 150 mM NaCl, 0.5% Nonidet P-40, 1 mM EDTA, and protease inhibitors (10 µg/mL leupeptin and 10 µg/mL aprotinin). The protein extracts were clarified by centrifugation at 10,000 × g for 10 min. Protein amounts were quantitated by BCA protein assay (Thermo Fisher Scientific). The equal amount of protein was subjected to SDS-PAGE and immunoblotting with antibodies against LC3, glyceraldehyde-3-phosphate dehydrogenase (GAPDH) (Wako Pure Chemical Industries), or poly(ADP-ribose) polymerase (PARP) (Cell Signaling Technology). The luminescent signals were analyzed using LAS-4000 image analyzer (Fuji Film).
Apoptosis assay by annexin V/propidium iodide (PI) staining
HaCaT cells were treated with sudachitin (30, 100 µM), 3′-demethoxysudachitin (30, 100 µM), nobiletin (30, 100 µM), or DMSO for 24 and 48 hours. Cells were detached from the culture dish by trypsin/EDTA treatment and washed with culture medium and PBS. Annexin V-FITC and PI stating was performed using a MEBCYTO Apoptosis Kit (Medical & Biological Laboratories) according to the manufacturer’s instructions. The samples were analyzed by flow cytometry (BD FACSVerse system, BD Biosciences).
Immunofluorescence analysis
Immunofluorescence analysis was performed as previously described [Citation18]. In brief, HaCaT cells were treated with 100 µM nobiletin, sudachitin, or DMSO. After 24 hours, cells were fixed, permeabilized, and then blocked in 5% BSA. Cells were subsequently incubated with mouse anti-LC3 antibody (Wako Pure Chemical Industries) overnight at 4°C. After washing with PBS, cells were incubated for 1 hour with Alexa Fluor 488-conjugated goat anti-rabbit IgG antibody (Thermo Fisher Scientific). Fluorescent images were obtained using an IN Cell Analyzer 6000 system (GE Healthcare).
Autolysosome detection
HaCaT cells were treated with 1 µM DALGreen (Dojindo Laboratories) at 37°C for 30 min. Then the culture medium was removed, and cells were incubated in culture medium containing 100 µM nobiletin at 37°C for 24 hours. Cells were observed by IN Cell Analyzer 6000 to obtain fluorescence images.
Statistical analysis
All experiments were performed multiple times to confirm their reproducibility. One representative set of data was shown in the figures. Immunoblot band intensities were quantified using Image J software (NIH). Data were expressed as the mean ± standard error, and statistical analysis was performed by Student’s t-test or one-way analysis of variance (ANOVA) with Tukey’s multiple comparison tests using GraphPad Prism (GraphPad Software).
Results
Effect of sudachitin and nobiletin on the proliferation of keratinocytes
The primary function of the skin is to protect the body against undesired influences from the environment. The skin is composed of the epidermis and the dermis, and keratinocytes are the principal cell type of the epidermis (90% of total epidermal cells). In order to assess the effects of sudachitin (5,7,4′-trihydroxy-6,8,3′-trimethoxyflavone) (, left) and 3′-demethoxysudachitin (5,7,4′-trihydroxy-6,8-dimethoxyflavone) (, middle) on cell proliferation in keratinocytes, immortalized human keratinocyte HaCaT cells were treated with 30 or 100 µM sudachitin or 3′-demethoxysudachitin for 24 and 48 hours and then subjected to an MTT assay. As shown in , sudachitin significantly suppressed the proliferation of HaCaT cells in a dose-dependent manner. Although 3′-demethoxysudachitin exhibited an antiproliferative activity at a concentration of 100 µM, little effect was observed at 30 µM. Additionally, their analogous compound, nobiletin (5,6,7,8,3′,4′-hexamethoxyflavone) (, right), was also tested, resulting in significant growth suppression at 30 and 100 µM. The results showed that three polymethoxyflavones suppressed the proliferation of HaCaT cells at a high concentration (100 µM).
Sudachitin specifically induces apoptosis in a concentration- and time-dependent manner
Next, we examined whether these polymethoxyflavones induce apoptosis. Due to the fact that poly(ADP-ribose) polymerase (PARP) (116 kDa) is cleaved to fragments of molecular sizes of 85 and 27 kDa by activated caspase-3 in response to apoptotic signals, the cleavage of PARP is widely used as an apoptotic marker [Citation19]. Immunoblotting analysis with an anti-PARP antibody revealed that the amount of cleaved PARP significantly increased in HaCaT cells treated with 100 µM sudachitin, but not in those treated with 3′-demethoxysudachitin and nobiletin at an equivalent concentration (). As shown in , sudachitin induced PARP cleavage in a concentration- and time-dependent manner. In order to obtain further evidence that sudachitin induces the apoptosis of HaCaT cells, sudachitin-treated cells were subjected to annexin V-FITC/PI staining and analyzed by flow cytometry. As shown in , the percentages of annexin V-positive/PI-negative cells, indicative of early apoptosis, were significantly increased to 26% and 41%, respectively, when cells were treated with 100 µM sudachitin for 24 and 48 hours, compared with DMSO treatment (5.9% and 6.5%). In contrast, nobiletin and 3′-demethoxysudachitin hardly affected. We also examined the dose dependence of the effect of sudachitin on induction of early apoptosis. As shown in , sudachitin promoted early apoptosis in a concentration-dependent manner. Additionally, the percentage of the sum of early apoptotic (annexin V-positive/PI-negative) and late apoptotic/necrotic (annexin V-positive/PI-positive) cells was also calculated. As shown in , the percentage significantly increased to approximately 80% in the cells treated with 100 µM sudachitin for 48 hours, which almost correlated with the percentage of cleaved PARP (84–94%) in . These results show that sudachitin but not 3′-demethoxysudachitin and nobiletin specifically induces apoptosis.
Figure 2. Sudachitin specifically induces apoptosis in a concentration- and time-dependent manner.
(a) HaCaT cells were stimulated with 100 μM nobiletin (N), 100 μM sudachitin (S), 100 μM 3′-demethoxysudachitin (deS), or DMSO (C) for 48 hours. (b and c) HaCaT cells were treated with 30 and 100 μM sudachitin for 24 and 48 hours. The cell lysates were analyzed by immunoblotting using anti-PARP and anti-GAPDH antibodies. The detected bands were quantitated by ImageJ software, and the percentage of cleaved PARP (associated to apoptosis) relative to the total amount of PARP (full-length plus cleaved PARP) was quantified. The data were expressed as the mean ± standard error, and statistical analysis was performed by one-way analysis of variance (ANOVA) with Tukey’s multiple comparison tests using GraphPad Prism. *** p < 0.001, ** p < 0.01, * p < 0.05. (d) HaCaT cells were treated with sudachitin (30, 100 μM), nobiletin (100 μM), 3′-demethoxysudachitin (100 μM), or DMSO (control) for 24 or 48 hours. The cells were stained by annexin V-FITC and PI, and were analyzed using flow cytometry. (e and f) The amount of early apoptosis were determined as the percentage of annexin V (+)/PI (-) (the lower right quadrants of flow cytometric assay). (g) The percentage of both annexin V (+)/PI (-) (early apoptosis) and annexin V (+)/PI (+) (late apoptosis/necrosis) cells were shown. The data were expressed as the mean ± standard error, and statistical analysis was performed by one-way ANOVA with Tukey’s multiple comparison tests. *** p < 0.001.
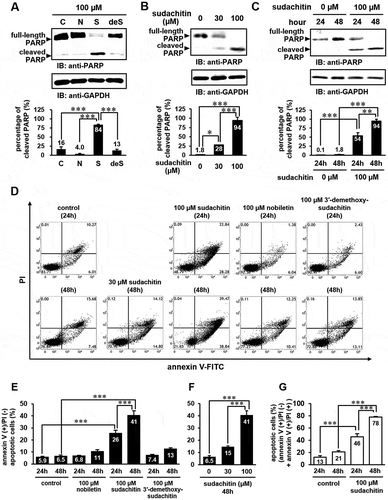
Nobiletin specifically triggers autophagy
Some natural polyphenolic compounds, such as genistein, quercetin, curcumin, and resveratrol, are known to induce not only apoptosis but also autophagy [Citation9]. Furthermore, it was reported that nobiletin induces apoptosis-mediated protective autophagy in human gastric cancer SNU-16 cells [Citation14]. Therefore, we examined whether nobiletin, sudachitin, and 3′-demethoxysudachitin induce autophagy in HaCaT cells. During the formation of autophagosomes, LC3-I is converted into LC3-II conjugated to phosphatidylethanolamine and recruited to the autophagosome membrane, although LC3-I is diffusely distributed in the cytoplasm under normal conditions [Citation5,Citation20]. The conversion of LC3-I into LC3-II through proteolytic cleavage and lipidation is a hallmark of autophagy, which can be quantified by immunoblotting analysis with an anti-LC3 antibody. As shown in , the level of LC3-II was significantly increased by treatment with nobiletin (30 and 100 μM) in HaCaT cells. On the other hand, 3′-demethoxysudachitin and sudachitin failed to induce autophagy. We subsequently confirmed that nobiletin induces autophagy by immunofluorescence analysis with an anti-LC3 antibody and cell staining with DALGreen, which is a fluorescent probe for monitoring the formation of autolysosomes [Citation21]. Immunofluorescence analysis showed that LC3 puncta, which represent autophagosome formation, were observed in HaCaT cells treated with 100 μM nobiletin (). Furthermore, an increase in DALGreen fluorescence was observed in cells treated with nobiletin (). These results show that three polymethoxyflavones have different effects on apoptosis and autophagy.
Figure 3. Nobiletin specifically triggers autophagy in HaCaT cells.
(a) HaCaT cells were treated with 100 μM nobiletin (N), 100 μM sudachitin (S), 100 μM 3′-demethoxysudachitin (deS), or DMSO (C) for 48 hours. (b) HaCaT cells were stimulated with nobiletin (30, 100 μM) or DMSO for 48 hours. The cell lysates were analyzed by immunoblotting using anti-LC3 and anti-GAPDH antibodies. The detected bands were quantitated by ImageJ software, and quantification of results is presented as the amount of LC3-II normalized against GAPDH. The data are expressed as means ± standard error of three separate experiments, and statistical analysis was performed by one-way ANOVA with Tukey’s multiple comparison tests. *** p < 0.001, * p < 0.05. (c and d) HaCaT cells were stimulated with 100 μM nobiletin, 100 μM sudachitin, or DMSO for 24 hours, and were subjected to immunofluorescence with an anti-LC3 antibody (c) and DALGreen staining (d). All experiments were performed multiple times with similar results.
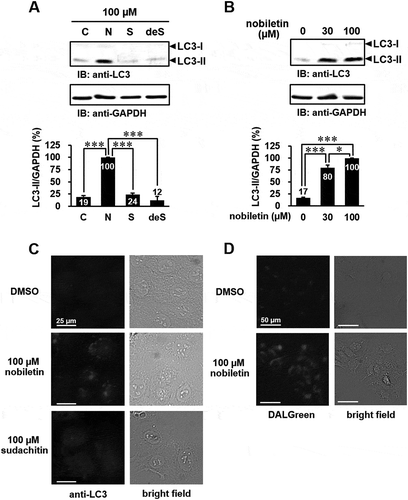
Discussion
A wide range of compounds that regulate apoptosis and/or autophagy have been identified and are expected to act as anticancer agents [Citation22]. Natural polyphenolic compounds isolated from plants could also affect apoptosis and autophagy [Citation9,Citation10,Citation13,Citation14]. Due to the fact that the avoidance of cell death is closely related to the promotion and progression of cancer, it is important to identify and characterize compounds that can regulate cell death processes. In this study, we demonstrated that sudachitin (5,7,4′-trihydroxy-6,8,3′-trimethoxyflavone), a polymethoxyflavone isolated from the peel of Citrus sudachi, could induce apoptosis in human keratinocyte HaCaT cells. On the other hand, nobiletin (5,6,7,8,3′,4′-hexamethoxyflavone) could induce autophagy, whereas it failed to promote apoptosis. Additionally, 3′-demethoxysudachitin (5,7,4′-trihydroxy-6,8-dimethoxyflavone) did not induce apoptosis or autophagy.
Citrus polymethoxyflavones are flavones that bear two or more methoxy groups on their basic benzo-γ-pyrone skeleton with a carbonyl group at the C-4 position [Citation23]. Maximum number of the methoxy groups can be up to seven (3,5,6,7,8,3′,4′-heptamethoxyflavone and 5,6,7,8,3′,4′,5′-heptamethoxyflavone), and one or more methoxy group(s) is (are) replaced by hydroxyl group(s) (hydroxylated polymethoxyflavone or demethyl polymethoxyflavone). The number and positions of methoxy group greatly influence the biological functions of polymethoxyflavones. For example, although nobiletin has an anti-apoptotic effect in human neuroblastoma SH-SY5Y cells, 5-demethylnobiletin (5-hydroxy-6,7,8,3′,4′-pentamethoxyflavone), which is demethylated at the 5-methoxy group in nobiletin, exhibits a growth inhibitory effect [Citation24]. These data suggest that the methoxy group at the C-5 position of nobiletin may contribute to the anti-apoptotic effect, and that hydroxylated polymethoxyflavones may effectively induce cell death. Sudachitin is also a hydroxylated polymethoxyflavone, which the methoxy groups at the C-5, C-7, and C-4′ positions in nobiletin are replaced by the hydroxyl groups, and therefore, sudachitin but not nobiletin may exhibit pro-apoptotic activity. On the other hand, 3′-demethoxysudachitin only slightly suppressed cell proliferation in HaCaT cells. Although 5-demethylnobiletin can strongly induce apoptosis in HL-60 leukemia cells, neither tangeretin (5,6,7,8,4′-pentamethoxyflavone) nor 5-demethyltangeretin (5-hydroxy-6,7,8,4′-tetramethoxyflavone) affects cell proliferation [Citation25]. Taken together, the methoxy group at the C-3′ position may exhibit a growth inhibitory effect. Further study will be required to elucidate the related structure-activity relationship of other polymethoxyflavones.
Although we demonstrated that nobiletin did not induce apoptosis in HaCaT cells, it has been reported that nobiletin promotes apoptosis by suppressing mitogen-activated protein kinases (MAPKs) in human breast cancer cell lines [Citation26]. Previous studies showed that some compounds induced cell-type-specific apoptosis and autophagy [Citation27,Citation28]. For example, honokiol, a natural biphenolic compound derived from the bark of magnolia trees, induces apoptosis and autophagy via the reactive oxygen species (ROS) signaling pathway in human osteosarcoma, whereas it does not induce cell death in normal human primary skin fibroblasts [Citation27]. Furthermore, a green tea polyphenol analog, JP8, preferentially induces cell death in cancer cells, such as murine B16 melanoma cells [Citation28]. JP8 can apparently induce selective ROS accumulation in cancer cells but not in normal cells, resulting in autophagic cell death. Interestingly, we found that, in B16 melanoma cells, sudachitin fails to induce apoptosis and triggers autophagy (S. Abe, S. Hirose, and K. Yuasa, unpublished data). A more recent study showed that sudachitin decreases the activation of MAPKs and the ROS production evoked by the receptor activator of NF-κB ligand in osteoclasts [Citation29], suggesting that sudachitin may also induce apoptosis and autophagy through a pathway such as ROS production.
In conclusion, we demonstrated that two polymethoxyflavones, sudachitin and nobiletin, induce distinct cellular responses in keratinocytes. Further studies are needed to clarify the mechanism behind sudachitin and nobiletin-induced cell death, and understanding the structure and biological activity of polymethoxyflavones may lead to the discovery of potential therapeutic agents.
Acknowledgments
The authors thank Miss Sara Murakami and Mr. Yusei Shinohara for their technical assistance.
Disclosure statement
No potential conflict of interest was reported by the authors.
References
- Green DR, Llambi F. Cell death signaling. Cold Spring Harb Perspect Biol. 2015;7:a006080.
- Mariño G, Niso-Santano M, Baehrecke EH, et al. Self-consumption: the interplay of autophagy and apoptosis. Nat Rev Mol Cell Biol. 2014;15:81–94.
- Booth LA, Tavallai S, Hamed HA, et al. The role of cell signalling in the crosstalk between autophagy and apoptosis. Cell Signal. 2014;26:549–555.
- Elmore S. Apoptosis: a review of programmed cell death. Toxicol Pathol. 2007;35:495–516.
- Mah LY, Ryan KM. Autophagy and cancer. Cold Spring Harb Perspect Biol. 2012;4:a008821.
- Martinet W, Agostinis P, Vanhoecke B, et al. Autophagy in disease: a double-edged sword with therapeutic potential. Clin Sci. 2009;116:697–712.
- Kim HS, Quon MJ, Kim JA. New insights into the mechanisms of polyphenols beyond antioxidant properties; lessons from the greentea polyphenol, epigallocatechin 3-gallate. Redox Biol. 2014;2:187–195.
- Yao LH, Jiang YM, Shi J, et al. Flavonoids in food and their health benefits. Plant Foods Hum Nutr. 2004;59:113–122.
- Hasima N, Ozpolat B. Regulation of autophagy by polyphenolic compounds as a potential therapeutic strategy for cancer. Cell Death Dis. 2014;5:e1509.
- Sergeev IN, Ho CT, Li S, et al. Apoptosis-inducing activity of hydroxylated polymethoxyflavones and polymethoxyflavones from orange peel in human breast cancer cells. Mol Nutr Food Res. 2007;51:1478–1484.
- Wang L, Wang J, Fang L, et al. Anticancer activities of citrus peel polymethoxyflavones related to angiogenesis and others. Biomed Res Int. 2014;2014:1–10.
- Zhang L, Zhang X, Zhang C, et al. Nobiletin promotes antioxidant and anti-inflammatory responses and elicits protection against ischemic stroke in vivo. Brain Res. 2016;1636:130–141.
- Yoshimizu N, Otani Y, Saikawa Y, et al. Anti-tumour effects of nobiletin, a citrus flavonoid, on gastric cancer include: antiproliferative effects, induction of apoptosis and cell cycle deregulation. Aliment Pharmacol Ther. 2004;20:95–101.
- Moon JY, Cho SK. Nobiletin induces protective autophagy accompanied by ER-stress mediated apoptosis in human gastric cancer SNU-16 cells. Molecules. 2016;21:e914.
- Nakagawa H, Takaishi Y, Tanaka N, et al. Chemical constituents from the peels of Citrus sudachi. J Nat Prod. 2006;69:1177–1179.
- Yuasa K, Tada K, Harita G, et al. Sudachitin, a polymethoxyflavone from Citrus sudachi, suppresses lipopolysaccharide-induced inflammatory responses in mouse macrophage-like RAW264 cells. Biosci Biotechnol Biochem. 2012;76:598–600.
- Tsukayama M, Sasaki T, Yamamoto K, et al. Microwave-assisted extraction and methylation of useful flavones from waste peels of Citrus sudachi. Nippon Shokuhin Kagaku Kogaku Kaishi. 2010;57:427–433.
- Matsuda S, Kawamoto K, Miyamoto K, et al. PCTK3/CDK18 regulates cell migration and adhesion by negatively modulating FAK activity. Sci Rep. 2017;7:45545.
- Yuasa K, Ota R, Matsuda S, et al. Suppression of death-associated protein kinase 2 by interaction with 14-3-3 proteins. Biochem Biophys Res Commun. 2015;464:70–75.
- Kabeya Y, Mizushima N, Ueno T, et al. LC3, a mammalian homologue of yeast Apg8p, is localized in autophagosome membranes after processing. EMBO J. 2000;19:5720–5728.
- Iwashita H, Sakurai HT, Nagahora N, et al. Small fluorescent molecules for monitoring autophagic flux. FEBS Lett. 2018;592:559–567.
- Wong VK, Li T, Law BY, et al. Saikosaponin-d, a novel SERCA inhibitor, induces autophagic cell death in apoptosis-defective cells. Cell Death Dis. 2013;4:e720.
- Gao Z, Gao W, Zeng SL, et al. Chemical structures, bioactivities and molecular mechanisms of citrus polymethoxyflavones. J Funct Foods. 2018;40:498–509.
- Akao Y, Itoh T, Ohguchi K, et al. Interactive effects of polymethoxy flavones from citrus on cell growth inhibition in human neuroblastoma SH-SY5Y cells. Bioorg Med Chem. 2008;16:2803–2810.
- Li S, Pan MH, Lai CS, et al. Isolation and syntheses of polymethoxyflavones and hydroxylated polymethoxyflavones as inhibitors of HL-60 cell lines. Bioorg Med Chem. 2007;15:3381–3389.
- Chen C, Ono M, Takeshima M, et al. Antiproliferative and apoptosis-inducing activity of nobiletin against three subtypes of human breast cancer cell lines. Anticancer Res. 2014;34:1785–1792.
- Kangmao H, Yanyan C, Rui Z, et al. Honokiol induces apoptosis and autophagy via the ROS/ERK1/2 signaling pathway in human osteosarcoma cells in vitro and in vivo. Cell Death Dis. 2018;9:157.
- Xie J, Yun JP, Yang YN, et al. A novel ECG analog 4-(S)-(2,4,6-trimethylthiobenzyl)-epigallocatechin gallate selectively induces apoptosis of B16-F10 melanoma via activation of autophagy and ROS. Sci Rep. 2017;7:42194.
- Ohyama Y, Ito J, Kitano VJ, et al. The polymethoxy flavonoid sudachitin suppresses inflammatory bone destruction by directly inhibiting osteoclastogenesis due to reduced ROS production and MAPK activation in osteoclast precursors. PLoS One. 2018;13:e0191192.