ABSTRACT
Asparagus (Asparagus officinalis) has several traits that make it a useful model for cytogenetic studies, however, few studies of the meiosis process have been made in asparagus. Here, we present in detail an atlas of male meiosis in asparagus, from preleptotene to telophase II. The meiosis process in asparagus is largely similar to those of the well-characterized model plants Arabidopsis thaliana, Zea mays, and Oryza sativa. However, most asparagus prophase I meiotic chromosomes show a strongly aggregated morphology, and this phenotype persists through the pachytene stage, highlighting a property in the control of chromosome migration and distribution in asparagus. Further, we observed no obvious banding of autofluorescent dots between divided nuclei of asparagus meiocytes, as one would expect in Arabidopsis. This description of wild-type asparagus meiosis will serve as a reference for the analyses of meiotic mutants, as well as for comparative studies among difference species.
Abbreviations: DAPI: 4’,6-diamidino-2-phenylindole; FISH: fluorescence in situ hybridization; PBS: phosphate-buffered saline; PMC: pollen mother cell; SEM: Scanning Electron Microscope
Graphical Abstract
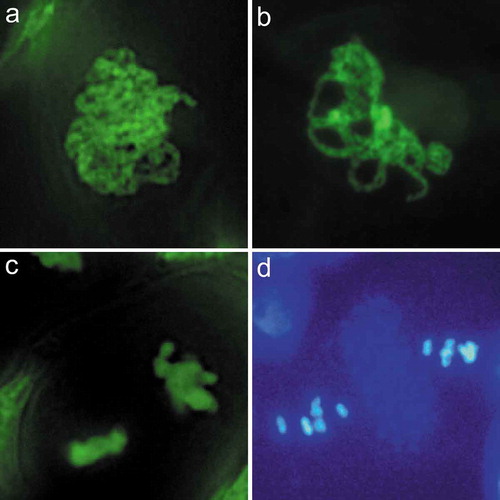
Asparagus chromosomes (a) are in a more aggregated state than Arabidopsis (b), and lacking autofluorescent band between nuclei (c), as one would expect in Arabidopsis (d).
KEYWORDS:
Meiosis is a succession of two rounds of cell division following a single DNA replication. This special type of cell division results in the production of haploid gametes. Pairing, synapsis, and recombination of homologous chromosomes each occur during prophase I of meiosis, resulting in increased genetic variation in progeny but maintaining a constant number of chromosomes. Even though homologous recombination forms the theoretical foundation for classical crop breeding and has profoundly impacted molecular crop breeding for the selection and maintenance of desirable traits, some of the mechanistic aspects of the process itself remain largely unknown [Citation1,Citation2]. A step towards resolving this issue of incomplete knowledge is to study the cytogenetic and molecular processes that occur during meiosis [Citation3–Citation5].
In flowering plants, male meiocytes (pollen mother cells, PMCs) develop in anther lobes. This renders these cells rather inaccessible and their extremely small size makes the cytological investigation of meiotic chromosomes difficult [Citation3,Citation6]. Early accounts about meiotic chromosome behavior were incomplete, and this lack of knowledge has been particularly pronounced for the events that may occur during prophase I, as the techniques used for cytogenetic investigations were technically challenging [Citation7–Citation12]. Ross et al. achieved great success in providing a comprehensive and clear atlas of meiosis in the model plant Arabidopsis (Arabidopsis thaliana) that was based on 4’,6-diamidino-2-phenylindole (DAPI) staining in conjunction with a spreading technique, and the use of a simple light microscope [Citation6]. This technique is the most commonly used method so far for characterizing chromosome appearance and for preparing chromosome samples for fluorescence in situ hybridization (FISH). We recently reported a fast labeling protocol for the visualization of male meiotic chromosomes in Arabidopsis in which meiocytes were squashed into a high-efficiency staining buffer and the chromosome phenotype could then be analyzed using a fluorescence microscope [Citation13].
Garden asparagus (Asparagus officinalis) is a valuable vegetable crop, a flowering plant in the genus Asparagus, it is also commonly used as a model system for the study of sex determination and evolution in plants [Citation14,Citation15]. Its haploid genome consists of five long, one medium, and four small chromosomes [Citation16,Citation17]. Relatively little progress has been made in the study of the meiosis process in asparagus. Early accounts of this area of research involved the use of light microscopes and recording results using hand-drawn illustrations [Citation18,Citation19]. Since the 1990s, more reports on meiosis of asparagus have appeared, in which researchers used light microscopy and recorded their results with photographs [Citation20–Citation23], but these results are relatively incomplete, in the sense they focused on those relatively condensed chromosomes of post-diplotene stages, the earlier prophase I stages have been avoided.
Current research examining meiotic progress has mainly focused on Arabidopsis, maize (Zea mays), and rice (Oryza sativa) [Citation1,Citation2,Citation24]. With the rapid progress in plant genomics, it is possible to undertake detailed structural and functional comparisons of genes among model systems and crop plants that are involved in various aspects of the control of meiosis. Since the quick labeling protocol works well in Arabidopsis, which has small chromosome size and thick meiotic cell walls [Citation13], it promises to be useful for studying meiotic behavior in Asparagus. In this report, we provide a comprehensive microscopic view of meiosis in asparagus.
Materials and methods
Plant materials
A. officinalis plants were grown in the field (35°19′27.32′′N, 113°54′25.45′′E) with standard horticultural management practices. Plants were grown under natural environmental conditions. The average day/night temperatures were 32°C/20°C during the experiment.
Cytological preparation
The inflorescences of male A. officinalis were collected from young shoots and placed on ice. The male buds were used for specimen preparation. A glass slide was placed on the stage of a ZX61 dissecting microscope (Olympus, Tokyo, Japan), and 5 μL of labeling buffer containing 5 μM SYTOX Green Nucleic Acid Stain (Life Technologies, Carlsbad, USA) in phosphate-buffered saline (PBS, pH = 7.2–7.4) was applied to the slide. Three to six anthers were dissected from the flower buds ()) and collected into the labeling buffer using a pair of 1 mL disposable syringes with needles. A 22 × 22 mm cover slip (thickness 0.13–0.17 mm) was placed over the sample area and a gentle pressure was applied to release meiocytes, the slide is now ready for examination using a fluorescence microscope. Chromosome spreading and DAPI staining were performed as described [Citation6].
Figure 1. Anther and pollen development in asparagus (Asparagus officinalis). (a). Asparagus inflorescence (above) and transparent anthers dissected from floral buds (bottom). (b). Dissection of an asparagus bud showing the number of anthers. (c). A cross-sectional view of the asparagus anther. (d). A young anther with flat epidermal cells and a smooth surface. (e). A mature anther with wizened epidermal cells and a shrunken surface. (f). An asparagus tetrad with four divided nuclei and transparent callose walls. (g-h). A pollen grain imaged under a brightfield microscope (g) and a scanning electron microscope (h). Bars, 250 μm (a, c-e); 500 μm (b); 20 μm (f); 5 μm (g-h).
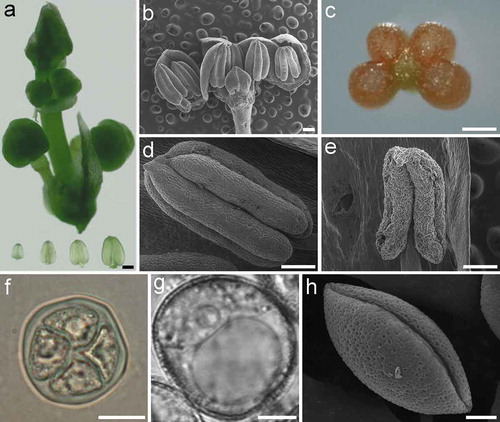
Light microscopy
The images of buds and anthers were captured using an Mshot MD30 camera (Micro-shot Technology, Guangzhou, China). Fluorescent dyes were observed using an Olympus BX63 fluorescence microscope and images were captured with a Zyla 5.5 sCMOS camera (Andor Technology, Belfast, UK) and the Metamorph software (Version 7.8.10.0, Molecular Devices, Downingtown, USA).
Scanning electron microscope (SEM)
Plant materials were sputter-coated with gold-palladium for 60 seconds with a KYKY SBC-12 Sputter Coater (KYKY Technology, Beijing, China) prior to imaging. SEM samples were attached to double sided carbon conductive tape and imaged with a Hitachi TM3030Plus TableTop SEM equipped with a Deben Coolstage (Hitachi, Hitachi, Japan). Mixed signals for secondary electrons and backscattered electrons of the SEM samples held under −20°C were captured with the SEM at an accelerating voltage of 15 kV.
Results
Asparagus anther development and microspore formation
Young healthy asparagus inflorescences and anthers dissected from floral buds are shown in ). The asparagus bud typically has six anthers ()); as in most flowering plants, each anther has four lobes ()), each of which contains pollen mother cells. The surfaces of young anthers are smooth, with flat epidermal cells ()), when pollen matures and is ready to be released from the anther, the epidermal cells of the anthers appear wizened ()), these appearance could be used as an assistant criteria for selecting appropriate anthers undergoing meiosis. Meiosis in microspore mother cells (PMCs) produces tetrads that have four haploid microspores ()). The microspore undergoes mitosis to give rise to a pollen grain (,h). Mature pollen grains have a net-like extine surface with a single sulcus (monosulcate), which is an elongated aperture ()).
Stage determination
The pressure resulting from application of the cover slip released three types of cells from the anther: early meiotic cell clusters that look like “worms”, these are mainly prophase I cells ()); separated meiocytes at later developmental stages, mainly containing cells at dyad stage to tetrad stage ()); and microspores ()). The released cells could be analyzed using fluorescence microscope without any additional treatment. The nucleic acids stained with SYTOX Green emitted bright green fluorescence and these cells examined in this way encompassed all meiotic stages (). Microspores could be also be thusly labeled ()).
Figure 2. An illustration of different cell types released from asparagus anthers. (a). Released meiotic cell clusters with a worm shape containing prophase I meiocytes. (c). Separated meiocytes with cells mainly at tetrad stage. (e). Microspores. (b), (d), and (f) are the labeling results of different cell types shown in (a), (c), and (e), respectively. Bars, 250 μm (a); 10 μm (b-f).
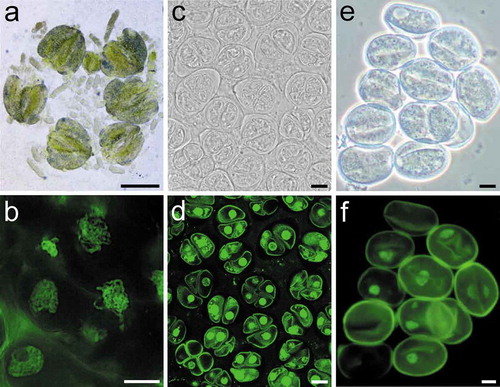
The meiotic stage of the cells was identified mainly based on knowledge of meiotic development in other Angiosperms, such as Arabidopsis [Citation6], maize [Citation25,Citation26], tomato [Citation27], and rice [Citation28,Citation29], and partly according to the morphology of anthers and/or buds. However, it should be noted that the size of anthers or buds in meiosis can be affected by environmental factors and varies among different shoots. The anther transparency is a helpful indicator of meiosis. Most, if not all, types of meiotic cells can be obtained from anthers that look transparent and are of a size ranging from 0.5 mm to 0.9 mm ()). The floral buds at the base portion of an asparagus inflorescence develop earlier than those near the top. The quick labeling protocol provides a quick method for obtaining buds at the appropriate meiotic stage by quickly determining the meiotic stage of one bud, and then switching to another bud on the same inflorescence in a manner consistent with the order of the development of the buds.
Prophase I
Prophase I, the earliest stage of meiosis, is typically divided into five substages including the leptotene, zygotene, pachytene, diplotene, and diakinesis stages. The preleptotene cell shows a relatively undifferentiated and evenly-distributed nucleus with only faint indicators of chromosome threads ()).
Figure 3. Meiotic stages of asparagus. (a). Preleptotene, showing a relatively undifferentiated nucleus with some faint indications of chromosome threads. (b). Leptotene, showing unpaired chromosomes. (c). Zygotene, showing partially paired homologous chromosomes; both synapsed (arrow) and unsynapsed (concave arrows) regions can be observed, showing synapsis in progress. (d). Pachytene, showing complete synapsis of homologous chromosomes. In asparagus, the chromosome groups are still prominent in this stage. (e). Diplotene, showing partially desynapsed chromosomes. (f). Diakinesis, chromosomes are highly condensed, individual bivalents can be identified. An “X” shape (arrow) or an “O” shape (concave arrow) can be seen in bivalents. (g). Metaphase I, condensed bivalents aligned along the spindle equator, the insert is a meiocyte when the source light is perpendicular with the equatorial plate of the cell. (h). Anaphase I, chromosomes are separating and are moving toward the spindle poles. (i). Telophase I, showing two polar groups of chromosomes. (j). Dyad stage, two nuclei with partially decondensed chromosomes. (k). Metaphase II, showing two groups of condensed chromosomes aligned at each spindle equator. (l). Anaphase II, showing separation of chromatids towards each spindle pole. (m). Telophase II, showing four groups of chromatids. (n). Tetrads of four haploid nuclei. Figures (a-f), and (g-n) are at the same magnification, respectively. Bars = 10 μm.
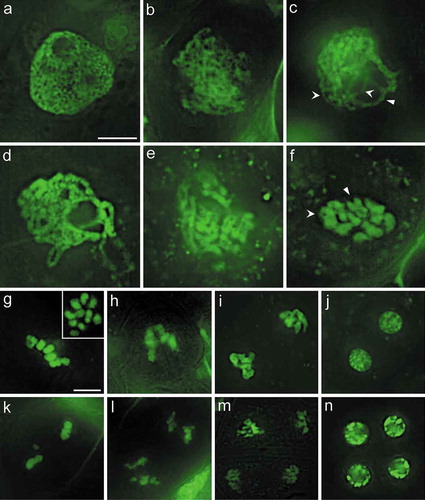
Leptotene is the first substage of the meiosis prophase I. During this stage, fine chromosome threads are visable; these are single threads, as the homologous chromosomes have not yet paired ()).
Alignment of the homologous chromosome occurs during the zygotene stage. Both synapsed and unsynapsed regions can be observed, showing synapsis in progress. Synapsed homologous chromosomes can be clearly identified as a double structure, which reflects the initial pairing of the homologous chromosomes ()).
Chromosomes are completely synapsed during the pachytene stage ()). It is easy to identify bivalents that are formed by two homologous chromosomes juxtaposed against each other. The chromosome threads are heavily stained and increasingly thick by this stage. Although aligned chromosome bivalents can be individually tracked in this stage in Arabidopsis and rice [Citation6,Citation13,Citation28], this remains difficult in asparagus ()), therefore, further improvement of the fast labeling method or application of chromosome spreading [Citation6] before labeling might increase the availability of this stage.
Chromosomes were extended, tenuous, and tangled together until the late diplolene stage and onwards to the end of the second meiotic division. Paired homologous chromosomes begin to desynapse during the diplotene stage ()). Homologous chromosomes in bivalents were not in close contact along most of the length of the chromosome. However, they remained in contact at one or a few nodes, referred to as chiasmata, which is the cytological manifestation of crossover between homologous chromosomes ()).
Diakinesis is the last substage of prophase I. The bivalents get more condensed during diakinesis than during the diplotene stage, and become evenly dispersed in the nucleus, allowing the ten bivalents to be tracked ()). As the crossover resulted in the formation of one or a few chiasmata, local “X” (one crossover) or “O”(two crossovers) shapes could be seen in the bivalents ()).
Meiosis I stages following prophase I
At metaphase I ()), bivalents are aligned along the spindle equator of the cell, and the kinetochores come into contact with the spindle apparatus. All chromosomes were maximally condensed during metaphase I, leading to small size bivalents ()). In addition to the diakinesis stage, the metaphase I stage is another stage during which one is able to count the chromosome number of the asparagus meiocytes when the source light is perpendicular with the equatorial plate of the cell () insert).
During anaphase I, the homologous chromosomes of asparagus migrate away from the equator and separate towards opposite poles of the spindle, resulting in two groups of chromosomes ()). Although the chromosomes could be clearly divided into two groups at this stage, the cohension between them had not totally ended yet. Anaphase I cells were rarely observed, owing to the short temporal duration of this stage [Citation6]. Nevertheless, finding anaphase I cells was relatively easy here through screening for these cells in the commonly observed metaphase I meiotic cell clusters by considering the slightly asynchronous meiotic stages in single asparugus meiotic cell clusters.
Telophase I is the last stage of meiosis I. During this stage, two groups of chromosomes are already found at the poles of the spindle ()). Each group of chromosomes is completely separated in telophase I. This stage leads to the formation of a dyad in which the chromosomes in each nucleus decondenses ()), an event that indicates the completion of the first cell division of meiosis.
Meiosis II
At metaphase II, the chromosomes align along the equatorial plate of each nucleus and condense again to the second most compactly arranged state after that of metaphase I ()). The two sister chromatids of each chromosomes were separated and migrated toward the spindle poles during anaphase II ()), breaking the cohesion between the sister chromatids. Consequently, telophase II cells containing four chromatid groups were formed ()). The chromatids of the four groups were decondensed and formed four haploid interphase nuclei. The membrane of the pollen mother cells disappeared, and new membranes formed around each haploid nucleus to form a tetrad ()). The four haploid cells then separated from each other and developed into mature pollen grains.
Comparison of meiosis in asparagus and other model organisms
Meiosis in Arabidopsis, maize, and rice has been extensively studied in recent years and provides reference observations for meiotic process. Here we briefly compared the meiosis in asparagus and Arabidopsis and found some differences between them.
The protocol been used here is an “in situ” labeling technique that stains meiotic chromosomes in intact cells, which facilitates the observation of natural distribution pattern of meiotic chromosomes. At the leptotene and zygotene, the Arabidopsis chromosome threads observed through our labeling method frequently looped out from the dense chromosome group [Citation13], which is similar with observations of meiotic chromosomes prepared after spreading and DAPI staining in Arabidopsis, maize, and rice [Citation6,Citation25,Citation28]. However, this looping out of chromosome threads from the chromosome patches was barely observed in asparagus. In contrast, most meiocytes (>99%) completed meiosis I within an aggregated state, and this phenotype persisted through to the pachytene ()).
Figure 4. Comparison of meiosis in asparagus and Arabidopsis. (a). Pachytene of asparagus. (b). Pachytene of Arabidopsis. Asparagus chromosomes are in a more aggregated stage than Arabidopsis. (c). Metaphase II of asparagus. (d). Metaphase II of Arabidopsis, showing two nuclei separated by a band of autofluorescent dots (arrow). Figures (a-b), and (c-d) are at the same magnification, respectively. Bars = 10 μm.
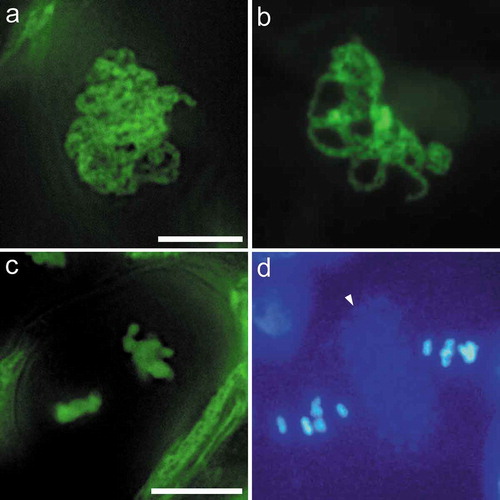
In meiocytes of Arabidopsis, the daughter nuclei are clearly separated by numerous autofluorescent dots; these DAPI-stained dots form a distinct band at the dyad stage, and this band persists into meiosis II (), shows that of the Metaphase II stage) [Citation6]. This band was also observed in Arabidopsis during Metaphase II and Anaphase II with our labeling method [Citation13]. In contrast, there was no obvious autofluorescent dots present throughout meiosis II in asparagus ( and ).
Discussion
This report presents a morphological study of meiosis and pollen development in asparagus. Asparagus has determinate inflorescence, the flower buds on a single asparagus inflorescence undergo meiosis in a relatively short time span, similar to the situation in maize and rice [Citation3,Citation26], but different from meiosis in Arabidopsis, which has an indeterminate inflorescence with buds that undergo different meiotic stages over a relatively long period. However, asparagus shoots with inflorescences sprout continuously for a period of a few months, thereby practically providing a long time span during which buds are available for studies of meiosis. Asparagus is a perennial plant and is easy to grow. Asparagus plants can reproduce through sexual reproduction with seeds, as well as through asexual reproduction via tuberous roots. It is therefore easy to create new genetic variations in asparagus through sexual reproduction, and to maintain and propagate experimental germplasm via asexual reproduction. These traits make asparagus an alternative model organism for understanding the meiotic biology of flowering plants.
In a previous study, we presented the meiosis process of Arabidopsis as visualized using the same labeling method that was used in the present study [Citation13]. During leptotene to zygotene of prophase I, the Arabidopsis chromosome threads frequently looped out from the relatively dense chromosome groups [Citation13]. However, this looping out of chromosome threads from the chromosome patches was barely observed in asparagus, and these chromosomes completed meiosis I within an aggregated state. This difference may reflect a natural difference in the process of meiotic chromosome migration and/or distribution between the two organisms, since our protocol stains meiotic chromosomes in intact cells and remained their natural distribution. Therefore, if it is required to tracking the individual pachytene chromosome bivalents in this stage, a fine spreading and purifying procedure might be conducted [e.g. Citation30]. During the diakinesis stage of meiosis I and metaphase I, all ten of the bivalents could be observed (), making them obvious stages of choice for investigating a complete set of bivalents.
The DAPI or SYTOX Green stained autofluorescent dots between meiotic daughter nuclei were clearly observed in Arabidopsis ()) [Citation13]. In rice, although lacking an obvious autofluorescent dots, a clear cell plate that can be observed following meiosis I [Citation28]. However, there was no obvious band of autofluorescent dots and cell plate observed throughout meiosis II in asparagus, at least with our labeling method ( and ), indicates it should be caution to use them as a indicator of meiotic stages in all organisms. Although we currently do not know whether the observed autofluorescent signal is associated with any organelle or other cellular structure, our results suggests a difference in cytokinesis or cell structure biogenesis materials among these plant species.
In this study, meiocytes were squashed out into PBS buffer with SYTOX Green stain on a slide to easily enable the observation of the meiotic process. The various stages of meiocytes could be identified in a timely rapid manner. Thus, this protocol could be used in rapid screening of materials in meiotic studies.
Conclusions
The present study describes a comprehensive visualization of normal meiotic chromosomes in asparagus. This study will serve as a reference for comparing meiosis in wild-type and mutant phenotypes, and establishes a basis for karyotype comparisons and phylogenetic analyses.
Author Contribution
JH.Y. performed the experiments, analyzed data, and wrote the paper. JJ.Y., H.Z., Q.S., S.J., Y.L. and S.G. performed some of the experiments and data analysis. J.L. designed the experiments, analyzed data, and wrote the paper.
Availability of data
All data generated or analysed during this study are included in this article.
Acknowledgments
We acknowledge help from Dr. Changbin Chen, University of Minnesota, with suggestions for improve this study.
Disclosure statement
No potential conflict of interest was reported by the authors.
Additional information
Funding
References
- Luo Q, Li Y, Shen Y, et al. Ten years of gene discovery for meiotic event control in rice. J Genet Genomics. 2014 Mar 20;41(3):125–137. PubMed PMID: 24656233; eng.
- Osman K, Higgins JD, Sanchez-Moran E, et al. Pathways to meiotic recombination in Arabidopsis thaliana. New Phytol. 2011 May;190(3):523–544. PubMed PMID: 21366595; eng.
- Cheng Z. Analyzing meiotic chromosomes in rice. Methods Mol Biol. 2013;990:125–134. PubMed PMID: 23559209; eng.
- Armstrong S. Spreading and fluorescence in situ hybridization of male and female meiocyte chromosomes from Arabidopsis thaliana for cytogenetical analysis. Methods Mol Biol. 2013;990:3–11. PubMed PMID: 23559197; eng.
- Sheehan MJ, Pawlowski WP. Imaging chromosome dynamics in meiosis in plants. Methods Enzymol. 2012;505:125–143. PubMed PMID: 22289451; eng.
- Ross KJ, Fransz P, Jones H. A light microscopic atlas of meiosis in Arabidopsis thaliana. Chromosome Res. 1996;4:507–516.
- Steinitz-Sears LM. Chromosome studies in Arabidopsis thaliana. Genetics. 1963 Apr;48(4):483–490. PubMed PMID: 17248159; PubMed Central PMCID: PMCPMC1210487. eng.
- Steinitz-Sears L, Lee-Chen S. Cytogenetic studies in Arabidopsis thaliana. Can J Genet Cytol. 1970;12:217–223.
- Ramulu K, Sybenga J. Comparison of fast neutrons and X-rays in respect of genetic effects accompanying induced chromosome aberrations: induction and analysis of aberrations in Arabidopsis thaliana. Arabidopsis Inf Serv. 1979;16:27–34.
- Klasterska I, Ramel C. Meiosis in PMCs of Arabidopsis thaliana. Arabidopsis Inf Serv. 1980;17:1–10.
- Vieira M, Briarty L, Mulligan B. A method for analysis of meiosis in anthers of Arabidopsis thaliana. Ann Bot. 1990;66:717–719.
- Albini SM. A karyotype of the Arabidopsis thaliana genome derived from synaptonemal complex analysis at prophase I of meiosis. Plant J. 1994;5(5):665–672.
- Li J, Yuan J, Zhao X, et al. A simple and rapid method for visualization of male meiotic chromosomes in Arabidopsis thaliana. Biosci Biotechnol Biochem. 2014;78(6):1026–1028.
- Harkess A, Zhou J, Xu C, et al. The asparagus genome sheds light on the origin and evolution of a young Y chromosome. Nat Commun. 2017 Nov 2;8(1):1279. PubMed PMID: 29093472; PubMed Central PMCID: PMCPMC5665984. eng.
- Li SF, Zhang GJ, Zhang XJ, et al. Comparative transcriptome analysis reveals differentially expressed genes associated with sex expression in garden asparagus (Asparagus officinalis). BMC Plant Biol. 2017 Aug 22;17(1):143. PubMed PMID: 28830346; PubMed Central PMCID: PMCPMC5567890. eng.
- Deng C, Qin R, Wang N, et al. Karyotype of asparagus by physical mapping of 45S and 5S rDNA by FISH. J Genet. 2012;91(2):209–212.
- Löptien H. Giemsa-Banden auf Mitosechromosomen desSpargels (Asparagus officinalis L.) und des Spinats (Spinacia oleracea L.). Z Pflanzenzüecht. 1976;76:225–230.
- Flory WS. Genetic and cytological investigations on Asparagus officinalis L. Genetics. 1932;17(4):432.
- Ammal EKJ, Kaul BL. Cytomorphological studies in autotetraploid Asparagus officinalis L. Proc Indian Acad Sci Sect B. 1967 Jan 1;65(1):1–9.
- Camadro EL. Cytological mechanism of 2n microscope formation in garden asparagus. HortScience. 1992;27(7):831–832.
- Camadro EL. Second meiotic division restitution (SDR) 2n pollen formation in diploid and hexaploid species of Asparagus. Genet Resour Crop Evol. 1994 Jan 1;41(1):1–7. English.
- Galli L, Viégas J, Augustin E, et al. Meiosis of anther culture regenerants in asparagus (Asparagus officinalis L.). Genet Mol Biol. 1998;21(1):93–97.
- Pontaroli AC, Camadro EL. Somaclonal variation in Asparagus officinalis plants regenerated by organogenesis from long-term callus cultures. Genet Mol Biol. 2005;28:423–430.
- Pawlowski WP. Chromosome organization and dynamics in plants. Curr Opin Plant Biol. 2010 Dec;13(6):640–645. PubMed PMID: 20970369; eng.
- Pawlowski WP, Golubovskaya IN, Cande WZ. Altered nuclear distribution of recombination protein RAD51 in maize mutants suggests the involvement of RAD51 in meiotic homology recognition. Plant Cell. 2003 Aug;15(8):1807–1816. PubMed PMID: 12897254; PubMed Central PMCID: PMCPMC167171. eng.
- Dukowic-Schulze S, Sundararajan A, Ramaraj T, et al. Sequencing-based large-scale genomics approaches with small numbers of isolated maize meiocytes. Front Plant Sci. 2014;5:57. PubMed PMID: 24611068; PubMed Central PMCID: PMCPMC3933774. eng.
- Moens PB. A new interpretation of meiotic prophase in lycopersicon esculentum (tomato). Chromosoma. 1964 Jan 1;15(3):231–242.
- Chen C, Xu Y, Ma H, et al. Cell biological characterization of male meiosis and pollen development in rice. J Integr Plant Biol. 2005;47(6):734–744.
- Wang K, Tang D, Wang M, et al. MER3 is required for normal meiotic crossover formation, but not for presynaptic alignment in rice. J Cell Sci. 2009 Jun 15;122(Pt 12):2055–2063. PubMed PMID: 19470578; eng.
- Caixeta E, Carvalho C, Clarindo W. Modified protocol for obtaining isolated and high-resolution pachytene chromosomes. Nucleus. 2011 Apr 1;54(1):3–7. English.