ABSTRACT
MicroRNA-125b (miR-125b), which was previously proved to be a potential immunomodulator in various disease, attenuated mouse hepatic ischemia/reperfusion (I/R) injury in this study. miR-125b was decreased in RAW 264.7 cells exposed to hypoxia/reoxygenation (H/R). The expression of IL-1β, IL-6 and TNF-α in both serum and supernate were reduced in miR-125b over-expression groups. The hepatic histopathological changes were reduced in miR-125b agomir groups. In the miR-125b antagomir groups, serum levels of alanine aminotransferase (ALT) and aspartate aminotransferase (AST) were significantly elevated compared with negative control (NC) groups. The protein expression of TNF receptor-associated factor 6 (TRAF6), IL-1β and the phosphorylation of p65 (p-p65) were suppressed by the up-regulation of miR-125b. Furthermore, the nuclear translocation of p-p65, measured by immunofluorescence, was enhanced by the miR-125b inhibitors. In conclusion, our study indicates that miR-125b protects liver from hepatic I/R injury via inhibiting TRAF6 and nuclear factor kappa-light-chain-enhancer of activated B cells (NF-κB) signal pathway.
Graphical abstract
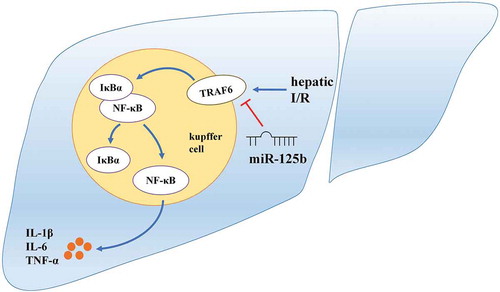
miR-125b attenuates hepatic I/R injury by suppressing TRAF6/NF-κB.
Hepatic I/R is unavoidable and harmful in the liver transplantation as well as other hepatic surgical and pathological processes [Citation1]. The main molecular mechanism of hepatic I/R, including apoptosis, oxidative stress and the release of inflammatory cytokines, remains to be uncovered [Citation2,Citation3]. Kupffer cells were reported as a major role in regulating the immune activity during hepatic I/R injury. Moreover, when triggered by hepatic I/R, NF-κB pathway in kupffer cells would be activated and lead to the generation of inflammation and aggravation of liver tissue damage [Citation4,Citation5].
TRAF6, a key mediator of NF-κB signalling, associates with IL-1R/TLR pathway and restrains the phosphorylation of IκBα [Citation6]. When activated, TRAF6 initiates an inflammatory cascade amplification, resulting in tissue damage and organ dysfunction [Citation7]. Giving rise to the release of IL-1β, IL-6 and TNF-α, TRAF6 plays a critical role in the signal conduction of NF-κB [Citation8]. Recent studies reported that TRAF6 might be a vital modulator in inflammatory response, which provides a new target for the therapy of hepatic I/R injury [Citation9,Citation10].
miR-125b is a novel potent anti-inflammatory factor, participating in the regulation of multiple inflammatory disease by targeting different signals [Citation11,Citation12]. It is reported that NF-κB pathway is the downstream of miR-125b while the specific mechanisms are diverse in different situations [Citation13–Citation15]. Previous research has shown that miR-125b could be a novel marker on renal I/R injury diagnosis [Citation16]. However, no study has been conducted to manifest the exact function and mechanism of miR-125b in the hepatic I/R injury. Thereby, miR-125b might serve as a protective factor in hepatic I/R injury and the further exploration of miR-125b in this model is needed. Here we investigated the function of miR-125b in hepatic I/R and found out that miR-125b protected liver through suppressing TRAF6 and NF-κB signalling.
Materials and methods
Animals
C57BL/6J mice were purchased from Chongqing Medical University Laboratory Animals Center, given free approach to water and food, and maintained in a standard environment with the light, humidity and temperature controlled. Healthy male mice weighing 25–28 g and aged 6–8 weeks were used. All animal procedures were approved by the Animal Care and Use Committee, Chongqing Medical University.
Hepatic I/R model
A 70% warm hepatic ischemia/reperfusion (1 h/6 h) model was established after anesthetized with the pentobarbital sodium (intraperitoneal injection, 50 mg/kg) according to a previous study [Citation17]. The sham groups underwent the same routine except the liver vascular clamping.
miR-125b agomir, negative control and antagomir transfection
Mice in the miR-125b agomir, negative control, antagomir or normal saline (NS) groups were injected with the agomir, negative control, antagomir (Genepharma, Shanghai, China) of miR-125b or normal saline via the tail vein, respectively, 1 h prior to I/R treatment. Blood and liver samples were collected after the reperfusion.
Biochemical detection of aminotransferase
Serum ALT and AST were detected by commercial kits (JianCheng Bioengineering Institute, Nanjing, China) according to the manufacturer’s instructions. A microplate reader (Biotek, Vermont, USA) was used to read the absorbance of the samples.
H&E staining
Liver tissues were fixed by formalin and then embedded into paraffin. After immobilization, samples were cut into sections (5 μm) and then stained with hematoxylin&eosin. A microscope (Olympus, Tokyo, Japan) was used to observe the results at an original magnification of 200×.
Cell culture
RAW 264.7 cells were bought from Cell Bank of the Type Culture Collection of the Chinese Academy of Sciences. Dulbecco’s modified Eagle’s medium (Gibco, Grand Island, USA) were used to culture cells, added with 10% fetal bovine serum and antibiotics (Gibco, Grand Island, USA). Cultivation atmosphere of cell incubator (Thermo, MA, USA) was controlled to be 95% air and 5% CO2 at the temperature of 37°C.
Cell hypoxia/reoxygenation model
The tri-gas incubator (Thermo, MA, USA) was used to attain the atmosphere of 94% N2, 5% CO2 and 1% O2 for hypoxia. As for the optimum H/R time, hypoxia for 1, 6, 9 or 12 h were set up followed by reoxygenation for 1, 6, 12 or 24 h according to a previous study [Citation18]. Finally hypoxia for 12 h within reoxygenation for 24 h was chosen to be an appropriate H/R time combination for the subsequent experiments.
miR-125b mimics, negative control and inhibitors transfection
The mimics, negative control (NC) and inhibitors (Genepharma, Shanghai, China) of miR-125b were transfected into cells at the concentration of 100 nM, 100 nM and 200 nM, respectively, according to the manufacturer’s instructions using Lipofectamine 2000 (Thermo, MA, USA). The double distilled water (ddwater) groups were set to verify the NC groups, and the cell culture medium was renewed 6 hours after transfection in all groups.
Immunofluorescence assay
RAW 264.7 cells were seeded on glass coverslips. After the transfection of miR-125b mimics, negative control and inhibitors for 24 h, cells were treated by H/R (12 h/24 h) and then immobilized using 4% paraformaldehyde for 20 min. Cells were blocked with goat serum for 1 h and permeabilized with 0.3% Triton X-100 for half an hour. Then the cells were incubated with anti-TRAF6, p-p65 and F4/80 antibodies (Abcam, Cambridge, UK) at 4°C overnight. Goat anti-mouse (Cy3-labeled, Beyotime Biotechnology, Shanghai, China) and Goat anti-rabbit (FITC-labeled, Beyotime Biotechnology, Shanghai, China) immunoglobulin G (IgG) were used for subsequent 1 h incubation at 37°C. The 4ʹ,6-diamidino-2-phenylindole was used to stain the cell nucleus. The results of immunofluorescence were analyzed using fluorescent microscopy (Olympus, Tokyo, Japan).
Enzyme-linked immunosorbent assay (ELISA)
The levels of IL-1β, IL-6 and TNF-α in mouse serum and cellular supernate were detected using the ELISA kits (Neobioscience, Beijing, China) according to the manufacturer’s instructions. A microplate reader (Biotek, Vermont, USA) was used to read the samples absorbance at 450 nm.
Western blot assay
Total protein was extracted from cells using protein extraction buffer (Beyotime Biotechnology, Shanghai, China). The protein concentration was measured using the bicinchoninic acid protein assay (Beyotime Biotechnology, Shanghai, China). After subjected to 10% sodium dodecyl sulfate-polyacrylamide gel electrophoresis, the protein was electrobloted to the polyvinylidene fluoride membranes. The defatted milk (5%) dissolved in TBST was used to block the PVDF membranes for 2 h. Washed by TSBT for 3 times after the block, the membranes were incubated overnight at 4 degrees centigrade with primary antibodies as followed: TRAF6 (Abcam, Cambridge, UK), IκBα, p-IκBα (Santa Cruz Biotechnology, Santa Cruz, USA), p65, p-p65, β-actin (Beyotime Biotechnology, Shanghai, China), IL-1β (Wanleibio, Shanghai, China). Then the membranes were washed and incubated with horseradish peroxidase-conjugated secondary antibodies (goat anti- rabbit or goat anti-mouse IgG, Beyotime Biotechnology, Shanghai, China) for 1 h. The ECL Chemiluminescence (Beyotime Biotechnology, China) was used to visualize (Bio-Rad, CA, USA) the protein.
Quantitative real-time PCR (qRT-PCR) assay
Total RNA was extracted from RAW 264.7 cells using Trizol (Takara, Dalian, China) according to the manufacturer’s instructions. The levels of mRNA were normalized to β-actin and the levels of miRNA were normalized to U6 according to a previous study [Citation19]. SYBP Premix Ex Taq (Takara, Dalian, China) was used to quantify the mRNA and miRNA levels, while the primer sequences were designed and synthesized as followed: miR-125b forward, 5ʹ-GCG CGT CCC TGA GAC CCT AAC-3ʹ and reverse, 5ʹ-ATC CAG TGC AGG GTC CGA GG-3ʹ; U6 forward, 5ʹ-AGA GAA GAT TAG CAT GGC CCC TG-3ʹ and reverse, 5ʹ-ATC CAG TGC AGG GTC CGA GG-3ʹ; TNF-α forward, 5ʹ-TAT GGC TCA GGG TCC AAC TC-3ʹ and reverse, 5ʹ-GGA AAG CCC ATT TGA GTC CT-3ʹ; IL-1β forward, 5ʹ-TGT AAT GAA AGA CGG CAC ACC-3ʹ and reverse, 5ʹ- TCT TCT TTG GGT ATT GCT TGG −3ʹ; IL-6 forward, 5ʹ-ACC AGA GGA AAT TTT CAA TAG GC-3ʹ and reverse, TGA TGC ACT TGC AGA AAA CA-3ʹ; TRAF6 forward, 5ʹ-AAC TGT GCT GTG TCC ATG GC-3ʹ and reverse, 5ʹ-CAG TCT CAT GTG CAA CTG GG-3ʹ; β-actin forward, 5ʹ-GGC TGT ATT CCC CTC CAT CG-3ʹ and reverse, 5ʹ-CCA GTT GGT AAC AAT GCC ATG T-3ʹ; All samples were run in triplicates thus taking the average in each experiment.
Statistical analysis
All experiment data both in vitro and in vivo was analyzed using the Statistical Package for the Social Sciences (version 22.0). All in vitro experiments were repeated thrice while the in vivo experiments were repeated five times (each group contains 5 samples each time). Comparisons between two different groups were determined using Student’s-t test. Differences among groups were performed using one-way ANOVA. P < 0.05 was considered significant.
Results
miR-125b reduces mRNA levels of pro-inflammatory cytokines and TRAF6
To investigate the role of miR-125b in hepatic I/R, we constructed cell H/R time gradients in vitro. miR-125b was significantly decreased after H/R treatment compared to the control groups, while 12/24 h was chosen to be a reasonable H/R time combination for subsequent experiments ()). LPS is a strong NF-κB stimulator [Citation20], and ) showed that the level of miR-125b was also decreased in LPS treated cells ()). The mimics, negative control and inhibitors of miR-125b were transfected into cells for further determination ()). As is consistent with above, the mRNA levels of IL-1β, IL-6 and TNF-α were decreased by miR-125b mimics, and on the contrary, increased by miR-125b inhibitors (-g)). The same situation happened to TRAF6 ()), which is critical in NF-κB signal transduction, suggesting that miR-125b might suppress TRAF6.
Figure 1. The related expression and effects of miR-125b in cell hypoxia/reoxygenation model. (a-b) The reoxygenation as well as the hypoxia time were fixed respectively to explore an appropriate H/R time combination according to the related expression of miR-125b. Finally hypoxia for 12 h followed by reperfusion for 24 h was chosen for subsequent experiments. (c) The cells were stimulated by LPS (100 ng/ml) for 6 h. (d) miR-125b mimics, negative control and inhibitors were transfected into cells for 24 h. (e-h) The mRNA levels of IL-1β, IL-6, TNF-α and TRAF6 after H/R treatment were detected. aP < 0.05 compared with control groups. bP < 0.01 compared with control groups. cP < 0.01 compared with NC groups.
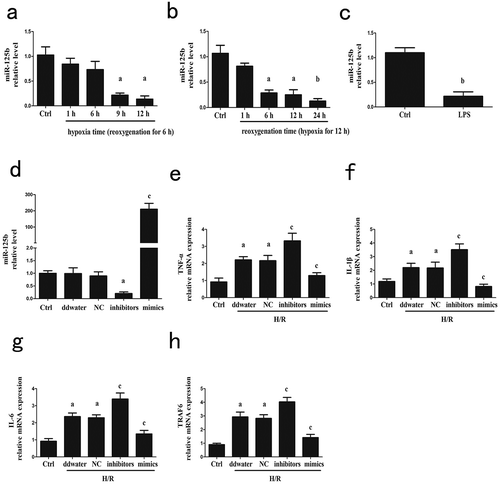
miR-125b decreases TRAF6 and NF-κB pathway protein expression
We further investigated the expression of TRAF6 and NF-κB related protein to clarify the internal mechanism. Data showed that the protein expression of p-p65, IL-1β and TRAF6 were up-regulated by miR-125b inhibitors, and p-IκBα was suppressed at the same time ()). In line with Western blot, the results of immunofluorescence manifested miR-125b as a depressor of TRAF6 ()). The protein expression of TRAF6 was remarkably reduced by the up-regulation of miR-125b. In addition, F4/80 was also detected as a biology marker of mature macrophages [Citation21]. Therefore, we concluded that miR-125b might reduce the expression of pro-inflammatory cytokines through inhibiting TRAF6 and NF-κB.
miR-125b suppresses the nuclear translocation of p-p65 and pro-inflammatory cytokine release
We assessed the nuclear translocation of p-p65 to evaluate the influence of miR-125b using the immunofluorescence ()). The H/R treatment improved p-p65 nuclear translocation. Transfection of miR-125b mimics relieved the nuclear translocation of p-p65, while miR-125b inhibitors reversed this phenomenon, obviously. Besides, miR-125b mimics blocked the levels of IL-1β, IL-6 and TNF-α in cell supernate, and the opposite effects were achieved by miR-125b inhibitors ()).
Figure 3. Effects of miR-125b on p-p65 nuclear translocation and pro-inflammatory cytokines.(a) The nuclear translocation of p-p65 in cells was measured. F4/80 was used as the molecular marker of RAW 264.7 cells. The original magnification = 400×. (b-d) Cell supernate levels of TNF-α, IL-1β and IL-6 were measured. aP < 0.05 compared with Ctrl groups. cP < 0.05 compared with NC groups.
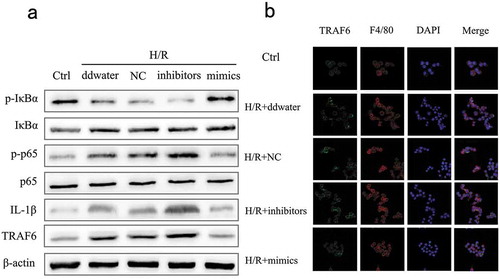
miR-125b attenuates mouse hepatic I/R injury
To further identify the protective effects of miR-125b upon hepatic I/R injury, we assessed the liver histopathological characteristics using H&E staining. After I/R treatment, the pathological changes were observed in the liver. The negative control groups showed no differences from normal saline groups. The transfection of miR-125b agomir, which increased the level of miR-125b in liver, decreased the histopathological changes (). Serum IL-1β, IL-6 and TNF-α were enhanced in miR-125b down-regulated groups. In contrast, a significant decrease of these pro-inflammatory cytokines was observed in the miR-125b over-expression groups (). In addition, miR-125b also reduced ALT and AST in mice serum faced with I/R injury ().
Figure 4. Effects of miR-125b on liver tissue treated by I/R. (a) H&E staining of liver tissue, the original magnification = 200 × . Histopathological changes of liver were marked by black line. (b-d) Serum TNF-α, IL-1β and IL-6 levels. (e-f) Serum level of ALT and AST. Mice were injected with miR-125b agomir, negative control, antagomir or normal saline 1 h prior to I/R treatment. aP < 0.05 compared with Ctrl groups. bP < 0.05 compared with NC groups.
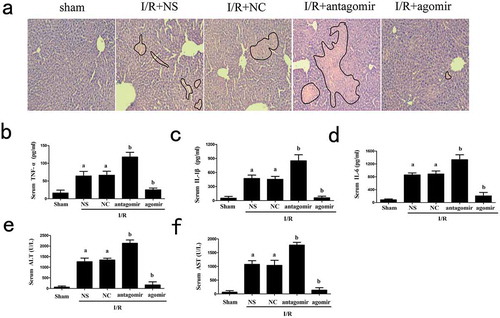
Discussion
As the primary reason of hepatic post transplantation damage, hepatic I/R injury initiates an inflammatory cascade mediated by NF-κB in the form of phosphorylation and nuclear translocation [Citation2,Citation22]. In this study, we demonstrated the protective effects of miR-125b in hepatic I/R, and our data showed that miR-125b attenuated hepatic I/R injury by suppressing TRAF6 and NF-κB signal pathway.
A dramatical decreased level of miR-125b was detected after H/R treatment. H/R enhanced the expression of IL-1β, IL-6 and TNF-α in cellular supernate, which are end products of NF-κB [Citation23], while miR-125b mimics reversed the impacts of H/R. In common with our findings, miR-125b was reported to be down-regulated in both cardiac and renal I/R injury [Citation16,Citation24]. LPS is a strong NF-κB stimulant that can increase the levels of pro-inflammatory cytokines. We also found a decreased expression of miR-125b after LPS stimulation in RAW 264.7 cells, indicating miR-125b might regulate the immune response caused by LPS. Furthermore, we detected a reduced expression of TRAF6 after miR-125b mimics transfection.
TRAF6 modulates NF-κB signal pathway, and its activation leads to the pathogen recognition and activation of inflammatory response [Citation25]. Activated TRAF6 can reduce the phosphorylation of IκBα, which works as an inhibitory combiner to NF-κB under normal circumstances [Citation26,Citation27]. In this study, we found that transfection of miR-125b mimics gave rise to p-IκBα protein expression and simultaneously caused the decline of TRAF6, p-p65 and IL-1β protein levels, thus blocking the subsequent NF-κB inflammatory cascades. Moreover, we proved that the enhancement of miR-125b reduced the nuclear translocation of p-p65. In cardiac I/R, TRAF6 and NF-κB pathway were proved to be therapeutic targets as reported [Citation24]. In our study, we demonstrated miR-125b suppressed TRAF6 and NF-κB pathway in hepatic I/R injury for the first time, and we further measured the phosphorylation of p65 and nuclear translocation of p-p65.
Recent studies showed that miR-125b might protect against I/R injury of other organs [Citation16,Citation24]). In harmony with them, we found that miR-125b decreased histopathological changes of liver tissue and the serum levels of IL-1β, IL-6 and TNF-α, implying an attenuated inflammatory response after I/R injury. Furthermore, we also found that ALT and AST were decreased by the miR-125b transfection. Our findings indicated miR-125b alleviated liver tissue damage after hepatic I/R injury.
From the above, we demonstrated that miR-125b induced an anti-inflammatory effect in hepatic I/R injury. What we found indicated that miR-125b functioned in hepatic I/R injury through inhibiting TRAF6 and NF-κB pathway. Therefore, further work is needed to establish the role of miR-125b in the hepatic I/R injury in clinical.
Author Contribution
Wu Z.J. and Huang Z.T. put forward the idea; Wu Z.J. and Zheng D.F. designed experiments; Huang Z.T., Pu J.L. and Dai J.W. carried out experiments; Zhang Y.C. and Zhang W.Q. analyzed data; Huang Z.T. and Zheng D.F. wrote the manuscript.
Disclosure statement
No potential conflict of interest was reported by the authors.
Additional information
Funding
References
- Montalvo-Jave EE, Escalante TT, Ortega-Salgado JA, et al. Factors in the pathophysiology of the liver ischemia-reperfusion injury. J Surg Res. 2008;147:153–159.
- Zhang Y, Lei XM, Li W, et al. TNIP1 alleviates hepatic ischemia/reperfusion injury via the TLR2-Myd88 pathway. Biochem Biophys Res Commun. 2018;501:186–192.
- Gao L, Chen X, Peng T, et al. Caveolin-1 protects against hepatic ischemia/reperfusion injury through ameliorating peroxynitrite- mediated cell death. Free Radic Biol Med. 2016;95:209–215.
- Luan X, Liu Y, Li M. The role of CD14 and Toll-like receptor 4 of Kupffer cells in hepatic ischemia-reperfusion injury in rats. Transplant Proc. 2012;44:937–941.
- Robinson SM, Mann DA. Role of nuclear factor κB in liver health and disease. Clin Sci. 2010;118:691–705.
- Wong BR, Besser D, Kim N, et al. TRANCE, a TNF family member, activates Akt/PKB through a signaling complex involving TRAF6 and c-Src. Mol Cell. 1999;4:1041–1049.
- Khursigara G, Orlinick JR, Chao MV. Association of the p75 neurotrophin receptor with TRAF6. J Biol Chem. 1999;274:2597–2600.
- Hu L, Xu JF, Xie XM, et al. Oligomerization-primed coiled-coil domain interaction with Ubc13 confers processivity to TRAF6 ubiquitin ligase activity. Nat Commun. 2017;8:814.
- Wu CL, Su ZX, Lin M, et al. NLRP11 attenuates Toll-like receptor signalling by targeting TRAF6 for degradation via the ubiquitin ligase RNF19A. Nat Commun. 2017;8:1977.
- Zhang QB, Qing YF, Yin CC, et al. Mice with miR-146a deficiency develop severe gouty arthritis via dysregulation of TRAF 6, IRAK 1 and NALP3 inflammasome. Arthritis Res Ther. 2018;20:45–53.
- Glaser S, Meng F, Han YY, et al. Secretin stimulates biliary cell proliferation by regulating expression of microRNA 125b and microRNA let7a in mice. Gastroenterology. 2014;146:1795–1808.
- Diao WL, Lu L, Li S, et al. MicroRNA-125b-5p modulates the inflammatory state of macrophages via targeting B7-H4. Biochem Biophys Res Commun. 2017;491:912–918.
- Tili E, Michaille JJ, Cimino A, et al. Modulation of miR-155 and miR-125b levels following lipopolysaccharide/TNF-alpha stimulation and their possible roles in regulating the response to endotoxin shock. J Immunol. 2007;179:5082–5089.
- Ma H, Wang XH, Ha TZ, et al. MicroRNA-125b prevents cardiac dysfunction in polymicrobial sepsis by targeting traf6-mediated nuclear factor κB activation and p53-mediated apoptotic signaling. J Infect Dis. 2016;214:1773–1783.
- Le MT, Teh C, Shyh-Chang N, et al. MicroRNA-125b is a novel negative regulator of p53. Genes Dev. 2009;23:862–876.
- Gu¨Clu¨ A, Kocak C, Kocak FE, et al. MicroRNA-125b as a new potential biomarker on diagnosis of renal ischemia/reperfusion injury. J Surg Res. 2017;207:241–248.
- He D, Guo Z, Pu JL, et al. Resveratrol preconditioning protects hepatocytes against hepatic ischemia reperfusion injury via Toll-like receptor 4/nuclear factor-κB signaling pathway in vitro and in vivo. Int Immunopharmacol. 2016;35:201–209.
- Wang XZ, Mao WZ, Fang C, et al. Dusp14 protects against hepatic ischaemia-reperfusion injury via Tak1 suppression. J Hepatol. 2018;68:118–129.
- Yang DK, Yuan QG, Balakrishnan A, et al. MicroRNA-125b-5p mimic inhibits acute liver failure. Nat Commun. 2016;7:11916.
- Medzhitov R, Preston HP, Janeway CA Jr. A human homologue of the Drosophila Toll protein signals activation of adaptive immunity. Nature. 1997;388:394–397.
- Wynn TA, Vannella KM. Macrophages in tissue repair, regeneration, and fibrosis. Immunity. 2016;44:450–462.
- Peralta C, Jimenez-Castro MB, Gracia-Sancho J. Hepatic ischemia and reperfusion injury: effects on the liver sinusoidal milieu. J Hepatol. 2013;59:1094–1106.
- Arkan MC, Hevener AL, Greten FR, et al. IKK-β links inflammation to obesity- induced insulin resistance. Nat Med. 2005;11:191–198.
- Wang XH, Ha TZ, Zou JH, et al. MicroRNA-125b protects against myocardial ischaemia/reperfusion injury via targeting p53-mediated apoptotic signalling and TRAF6. Cardiovasc Res. 2014;102:385–395.
- Chantzoura E, Prinarakis E, Panagopoulos D,G, et al. Glutaredoxin-1 regulates TRAF6 activation and the IL-1 receptor/TLR4 signalling. Biochem Biophys Res Commun. 2010;403:335–339.
- Ghosh S, Hayden M. Celebrating 25 years of NF-κB research. Immunol Rev. 2012;246:5–13.
- Zhang GL, Ghosh S. Toll-like receptor-mediated NF-κB activation: a phylogenetically conserved paradigm in innate immunity. J Clin Invest. 2001;107:13–19.