ABSTRACT
Bioavailability and bone loss inhibitory effects of vitamin D2 derived from UV-irradiated shiitake mushroom were determined in vivo. The effect of the absence of ovaries on the bioavailability of vitamin D2 and bone structure was also investigated. Sham operated (sham) and ovariectomized (OVX) rats were divided in 3 groups according to their diets, i.e. control: only vitamin D-deficient diets; UV(X): vitamin D-deficient diets with non-irradiated mushroom powder; UV(O): vitamin D-deficient diets with irradiated mushroom powder. The obtained results showed that vitamin D2 from shiitake mushroom was able to increase bone mineral density and trabecular bone structure of femur bone as well as its bioavailability. The absence of estrogen induced adverse effects not only on bioavailability of vitamin D2 but also on trabecular bone. In conclusion, vitamin D2-fortified shiitake mushroom might help postmenopausal women increase vitamin D2 bioavailability and retard trabecular bone loss.
Abbreviations: OVX: ovariectomized; 25(OH)D: 25-hydroxyvitamin D; 1,25(OH)2D: 1,25-dihydroxyvitamin D; BMD: bone mineral density; micro-CT: micro computed tomography; RSM: response surface methodology; RP-HPLC: Reverse phase-high performance liquid chromatography; MS/MS: tandem mass spectrometry; E2: estradiol; NTx: N-terminal telopeptide of type I collagen; BV/TV: bone volume/total volume; BS/BV: bone surface/bone volume; Tb.Th: trabecular thickness; Tb.Sp: trabecular separation.
Graphical abstract
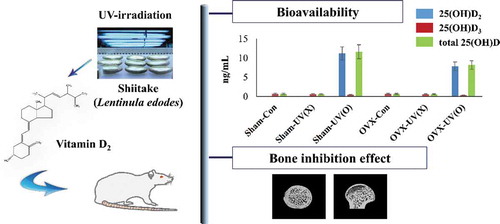
Vitamin D2-fortified shiitake mushroom not only showed high bioavailability, but also prevented loss of trabecular bone, and estrogen deficiency had negative effects on both.
The primary role of vitamin D is to maintain bone health, because vitamin D stimulates calcium absorption from the intestine and maintains calcium homeostasis [Citation1–Citation3]. Only 10–15% of dietary calcium and 50–60% of dietary phosphorus is absorbed in the absence of vitamin D [Citation4]. The interaction between 1,25-dihydroxyvitamin D [1,25(OH)2D], the final active form of vitamin D in the body, and the vitamin D receptor elevates the efficacy of intestinal calcium and phosphorus absorption to 30–40% and approximately 80%, respectively [Citation5]. In addition to calcium homeostasis, the roles played by vitamin D in arterial hypertension, autoimmunity and cancer have also been studied [Citation6].
Vitamin D deficiency is a global disorder, which also occurs in continents receiving sufficient sunlight [Citation7]. Insufficient vitamin D levels might worsen bone loss by decreasing calcium absorption and increasing parathyroid hormone level [Citation8]. The level of 25-hydroxyvitamin D [25(OH)D] is commonly regarded as a biomarker of vitamin D status because it has a longer half-life and is present at a higher concentration in the body, compared to 1,25(OH)2D [Citation9]. When the level of 25(OH)D is lower than 60 nmol/L, the fracture risk is higher [Citation10]. The lack of understanding prompted a clinical investigation into the association between vitamin D deficiency and immunity deficiency, diabetes mellitus, hypertension, and cancer [Citation11].
Osteoporosis, a bone disease generally occurring in the elderly, is defined by a bone mass of 2.5 standard deviations (SD) below that of healthy premenopausal adult females [Citation12]. The vertebrae, femoral neck, and distal radius of the patients suffering from osteoporosis are highly susceptible to fractures [Citation13]. There are two types of osteoporosis, “type 1 osteoporosis” is caused by estrogen deficiency, and “type 2 osteoporosis” is caused by aging [Citation14]. Type 1 osteoporosis is primarily characterized by the loss of trabecular bone within the first 10 years of menopause [Citation14], and the bone loss rate is more rapid than that observed in type 2 osteoporosis [Citation15]. Inhibition of osteoclastogenesis is one of the main mechanisms involving estrogen, which explains how bone loss could be prevented [Citation16]. Therefore, under conditions of estrogen deficiency, loss of bone mineral density (BMD) is accelerated by an increase in bone turnover, which is caused by more active bone resorption than bone formation [Citation17].
Ovariectomized (OVX) rat model is widely selected as a postmenopausal osteoporotic animal model, as the mechanism of bone mass gain and loss is known to be similar to that observed in humans [Citation18]. In some comparison studies, there was a similar trend in bone loss due to hormone deficiency, between postmenopausal women and ovariectomized rats [Citation19]. Although the remodeling characteristics of rat and human bone are not identical, reliable results can be obtained by studying the effects of estrogen on mineral density and other bone factors [Citation14].
Shiitake mushroom (Lentinula edodes) is one of the world’s famous edible, and second most commonly produced mushrooms [Citation20]. Shiitake mushroom showed anticancer properties against skin carcinoma cells [Citation21], as well as antioxidative properties after UV-B irradiation [Citation22]. A recent study conducted by Spim et al. [Citation23] showed that L. edodes is a good nutraceutical that can prevent hypercholesterolemia by reducing the level of very-low-density lipoprotein, while increasing the level of high-density lipoprotein. In addition, shiitake mushroom has higher content of ergosterol (6.05 ± 0.07 mg/g dry weight), a precursor of vitamin D2, compared to other types of mushrooms [Citation24].
The effects of vitamin D2 from UV-irradiated mushrooms on biological effects such as improvement in bioavailability or trabecular bone structure already have been reported previously. Bioavailability of vitamin D2-enriched shiitake mushroom was examined using male Wistar rats, and serum 25(OH)D level was notably increased in mushroom powder consumed groups compared to the other group [Citation9]. UV-B irradiated white button mushroom (Agaricus bisporis) was also effective in not only increasing serum 25(OH)D level but also improving femur bone architecture [Citation25]. Effect of vitamin D2 from pulsed-irradiated large oyster mushroom (Pleurotus ferulae) on bone health was studied toward OVX mice [Citation26]. In the study, mice group who consumed pulsed mushroom showed higher bone density and osteoblast indicators and lower osteoclast indicators compared to OVX control group. To the best of our knowledge, however, very few attempts have been made to study biological effects of vitamin D2 from mushrooms by dividing sham and OVX groups to study the effects of ovaries at the same time. The objective of this study was to investigate the effects of vitamin D2-fortified shiitake mushroom on serum 25(OH)D level as well as serum calcium level in both sham and OVX rats for 10 weeks. Moreover, bone loss-inhibitory effects were studied by micro computed tomography (micro-CT).
Materials and methods
Animals and ovariectomy
Forty-eight female Sprague–Dawley rats (4 weeks old) were purchased from Doo-Yeol Biotech. (Seoul, Korea). The animals were fed deionized water during the experiment to avoid the intake of calcium contained in tap water. Animals were housed in a room where temperature (23°C ± 1°C) and humidity (55% ± 5%) were constantly controlled, along with a 12-h light/dark illumination cycle. The rats were subjected to sham operation or bilateral ovariectomy, when the rats were 5 weeks old. The rats were anesthetized with a combined cocktail of ketamine and rompun (3:1). The dose, administered subcutaneously, was 0.44 mL/kg body weight. The rats underwent two-week acclimatization prior to diet-based grouping. During these two weeks, all the rats were fed the standard rodent chow (Altromin 1314; Altromin, Germany) and water ad libitum. The protocols followed for caregiving, experimentation, and killing in the study were approved by the Korea University Institutional Animal Care and Use Committee (no. KUIACUC-2015–274).
Vitamin D2 fortification and grouping
Sham and OVX rats were randomly divided into three groups according to their diet. To induce vitamin D deficiency, vitamin D deficient diet (Harlan Teklad, TD.89123, 0.47% Ca, 0.3% P) was purchased from Doo-Yeol Biotech. (Seoul, Korea) and used as the base diet and in cases where vitamin D2 was required, either UV-irradiated or non-irradiated shiitake mushroom powder was used. The optimal conditions derived from our previous study were applied for vitamin D2 fortification of shiitake mushrooms [Citation27]. In short, we selected three main factors (i.e. temperature, UV dosage, and moisture content) that can affect vitamin D2 synthesis, and determined the optimal conditions by using response surface methodology (RSM). The moisture content of shiitake mushrooms was controlled to 80.46% in a drying oven (80°C), and the mushrooms were subjected to UV irradiation (36.27 kJ/m2) at 40.56°C. The vitamin D2 content in UV-irradiated shiitake mushrooms was 25.54 ± 1.86 μg/g, which is slightly lower than the expected content (32.33 μg/g) by RSM. Both non-irradiated and UV-irradiated mushrooms were lyophilized and ground, before mixing with the vitamin D-deficient diet powder.
At six weeks of age, the rats were body-weight-matched and assigned into six groups, according to the presence/absence of ovaries and the type of diet provided (). One of three types of diets was fed to each group, for a period of 10 weeks. The diets were prepared as following: control (Con), only TD.89123; UV(X), TD.89123 mixed with non-irradiated mushroom powder; UV(O), TD.89123 mixed with irradiated mushroom powder. To adjust vitamin D2 content of UV(O) diet to the desired level (750 μg of vitamin D2/kg diet powder), 30 g of UV-irradiated shiitake mushroom powder and 970 g of vitamin D deficient diet powder were evenly mixed and pelleted. The same process was performed using non-irradiated mushrooms to prepare UV(X) diet.
Table 1. Grouping for experimental design.
Sham groups were allowed free consumption of diet and deionized water. The three diet groups in the OVX group were pair-fed with the diet of the corresponding sham groups’ to minimize the effects of hyperphagia that could accelerate the increase in body weight. Body weight and diet consumption were recorded weekly during the 10-week-experiment.
Vitamin D2 analysis
The vitamin D2 content of the UV-irradiated shiitake mushroom powder, before adding to the diet and after pelleting, were determined using a modified version of the method described by Ko et al. [Citation28]. Briefly, one gram of lyophilized sample powder and l-ascorbic acid were mixed in a 250-mL round-bottom flask, followed by alkaline saponification. Vitamin D2 was extracted with 30 mL of n-hexane and 10 mL of de-ionized water. The extraction step was repeated twice after the aqueous layer was removed. The organic layer was washed with de-ionized water until neutralization, and then transferred to a round flask for complete drying by rotary evaporation at 50°C. RP-HPLC was performed using an isocratic HPLC system (Waters 2690; Waters Corp.) equipped with a 996-photodiode array detector (Waters Corp.); the wavelength was set at 265 nm. The C-18 column (Polaris 3 μm, 3.0 × 250 mm; Agilent, Netherlands) was used for HPLC analysis. The mobile phase consisted of methanol/acetonitrile (25:75, v/v), and the flow rate was 0.43 mL/min. The recovery of vitamin D2 during the extraction process was determined by spiking the samples with known amounts of vitamin D2 (n = 3).
Preparation of serum and femur samples
All the rats were sacrificed through carbon dioxide asphyxiation and blood samples were collected via cardiac puncture at the end of the experiment. Serum samples were collected by clotting at room temperature for 30 min, followed by centrifugation for 10 min at 3000 rpm. The top layer was collected, and these samples were stored at – 80°C until analysis. The right femur was removed from each rat and fixed in 10% buffered formalin until histomorphometric analysis, and the left femur was stored at – 80°C right after dissection and thawed before a mechanical test. Both femurs were cleaned of soft adhering tissue and muscle.
Serum parameter analysis
Serum total 25(OH)D, calcium, and estradiol (E2) levels were measured at Green Cross Laboratories, Korea. Serum samples (100 μL) collected in microtubes were precipitated using 200 μL of the Daily Precipitation Solution, along with the standards, and quality controls (QCs). 2H6-25(OH)D3 and 2H6-25(OH)D2 were used as the standard and QC, respectively. The microtube containing the mixture was vortexed for 30 s and centrifuged at 10,000 rpm for 15 min. The supernatant (150 μL) of all the samples was then transferred into 96-well plates, which were then placed under a stream of high purity dry nitrogen gas (45–50°C) until dry. In each well, 50 μL of the derivatization reagent was added, followed by shaking at 750 rpm for 10 min at 30°C. The plate was loaded onto the autosampler and 50 μL of the samples was injected into the HPLC-MS/MS system. Solvent delivery and sample introduction were performed using Waters ACQUITY LC system (Waters, Milford, MA, USA) which was connected with a BEH C18-column (2.1 × 50 mm, packed with 1.7-μm particles; Waters). Mobile phases A and B consisted of 0.025% methylamine in 50% methanol and 100% methanol, respectively. The gradient program was as follows: initial was 60% B; 0–1.3 min: 100% B; 1.3–3.8 min and reversion of the mobile phase to 60% B; 3.8–4.3 min: 60% B. Serum total 25(OH)D level was calculated as the sum of 25(OH)D2 and 25(OH)D3 contents. The detection limit of both 25(OH)D2 and 25(OH)D3 was 0.13 ng/mL, and the limit of quantification was 0.38 ng/mL.
Serum calcium was determined by a quantitative colorimetric assay kit (CA2; Roche, Germany). To confirm ovariectomy, the control groups of Sham and OVX rats were subjected to serum E2 test. The serum E2 level was measured by an enzyme-linked immunoassay kit (Calbiotech, USA). The sensitivity of the assay was <3 pg/mL; the sensitivity was determined by calculating the mean ± 2SD of the standard zero point tested 20 times in the same run.
Histomorphometric analysis of the right femur by using micro-CT
The trabecular bone of the right proximal femurs, close to the growth plate was analyzed by micro-CT (VivaCT80; Scanco Medical AG, Switzerland) for all the 48 rats. The entire femur samples were imaged at an isotropic voxel size of 10 μm. Quantitative analyses were performed using μCT Evaluation Program V6.6 (Scanco Medical AG, Switzerland), and the same software was used for acquiring femur images. The trabecular bone was analyzed and set as the region of interest (ROI), starting at 60 μm distal to the proximal growth plate, to 750 μm downward (). The trabecular bone images of the femur were obtained using constant segmentation values: threshold of 350, Gauss sigma of 0.7, and Gauss support of 1.0. The following parameters were recorded: BMD, bone volume/total volume (BV/TV), bone surface/bone volume (BS/BV), trabecular thickness (Tb.Th), trabecular number (Tb.N), and trabecular separation (Tb.Sp).
Figure 1. Micro-CT images of trabecular bone of a proximal femur.
The region of interest (ROI) was set as starting from 60 μm distal to a proximal growth plate, including a 750 μm and spongy bone for analyses. (a) overall outline (yellow dotted contour), (b) cross section of ROI (transverse, as yellow contour).
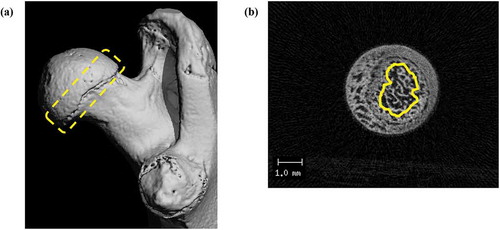
Length and bending strength of the left femur
Left femurs (total, 30; 5 samples/group) were randomly selected and rehydrated overnight in phosphate-buffered saline (PBS) at room temperature. Femur length was measured using a digital Vernier scale. Then, the femurs were subjected to mechanical strength test, by using a table top Instron 5569 universal testing machine. The maximum load of the mid-shaft of the left femur was measured by the three-point bending test. The cross-head speed was 10.0 mm/min, and the span between lower supports was 20 mm.
Statistical analysis
Data are presented as means ± SD. One-way analysis of variance (ANOVA) was used to compare different diet groups. Student’s t-test was performed to investigate the effect of the presence/absence of ovary in each diet group. A P value of <0.05 was considered significant (SPSS statistics software).
Results and discussion
Body weight of rats and daily vitamin D2 intake
illustrates the changes in body weight during the 10-week experiment period in the six groups. The initial body weight was similar (P > 0.05) across all six groups, except for the sham-UV(X) group, which showed a significant difference compared to other sham groups as well as with the diet counterpart OVX-UV(X) group. However, from week 1, no significant difference in body weight among all sham groups was noted until the end of the experiment as well as OVX groups. These findings suggest that vitamin D2 or mushroom components had no effect on body weight. When each sham-OVX counterpart diet group was compared, all OVX groups showed significantly higher body weight starting from week 1 until the end of the experiment. Therefore, it is likely that the absence of ovaries was a key factor to trigger an increase in body weight. Although it is not clear how ovariectomy induces body weight gain, fat deposition could be the reason for weight gain [Citation17,Citation26]. As estrogen is known to play an important role in inducing the differentiation of progenitor cells through the osteoblast lineage rather than the adipocyte lineage [Citation29], alterations in lipid metabolism might have been induced by the lack of estrogen [Citation30].
Figure 2. Body weight of rats over 10 weeks.
One-way ANOVA analysis was performed in sham or OVX groups, respectively, and Student’s t-test was used to compare each counterpart diet group. #P < 0.05 versus Sham-con by one-way ANOVA. *P < 0.05 versus Sham-con; †P < 0.05 versus Sham-UV(X); ‡P < 0.05 versus Sham-UV(O) by Student’s t-test.
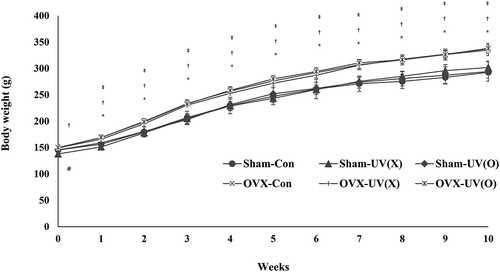
The purpose of this experiment was to determine the effects of vitamin D2, obtained from shiitake mushrooms fortified by UV irradiation under optimal conditions, on increasing serum 25(OH)D level in both sham and OVX rats. Before determining the dietary level of vitamin D2, we determined the optimum content of vitamin D2 that can enhance serum 25(OH)D level and prevent the induction of hypercalcemia in rats. A previous study had focused on enhancing 25(OH)D level by feeding vitamin D2-enhanced white button mushrooms to rats [Citation25]. The rats were fed with several diets that were mixed with AIN-93G, along with non-irradiated or UV-irradiated mushroom powder. The findings demonstrated the safety of supplying around 30,000 IU (750 μg) of vitamin D2 per kilogram of diet was safe; calcium accumulation was not observed in the kidney, liver, or spleen. The indirect effects of vitamin D on bone mineralization are well studied by Underwood and Deluca [Citation31]. They proved that in the presence of abundant calcium and phosphorus, vitamin D is not necessary for bone growth. To avoid such confounding effects of calcium, TD89123, a commonly used vitamin D deficient diet with normal calcium content (0.46%), was selected as the base diet for this experiment. The vitamin D2 content in the diet of the UV (O) group was 0.63 ± 0.09 μg/g, which was slightly lower than the expected content (0.75 μg/g). The average amount of the diet consumed by the rats in all groups for 10 weeks was 15.24 ± 0.30 g. Therefore, the daily intake of vitamin D2 in both groups through the UV (O) diet was approximately 9.60 μg, which was near the range of the recommended daily allowance of vitamin D for humans [i.e. from 400 IU (10 μg) to 600 IU (15 μg)], as reported by the Institute of Medicine [Citation32].
Serum vitamin D2 and calcium analyses
The results of serum metabolic analyses are shown in . Serum 25(OH)D2 was detected only in groups that consumed UV(O) diets, not in the control and UV(X) groups, irrespective of the presence of estrogen. Comparison of the 25(OH)D2 level between the sham-UV(O) and OVX-UV(O) groups showed that its value dropped by 70% in the absence of estrogen, suggesting that the presence of ovaries is highly associated with the bioavailability of vitamin D2. Serum 25(OH)D3 level showed an opposite tendency. Both sham-UV(O) and OVX-UV(O) groups showed a significant decrease (P < 0.05) in the serum 25(OH)D3 level, compared to the groups consuming vitamin D2-deficient diet (Con). It is likely that the increased serum 25(OH)D2 level because of vitamin D2 supplementation, triggered some sort of antagonism between 25(OH)D2 and 25(OH)D3. Our findings were consistent with the results of some clinical trials. In a six-week study conducted in healthy adults (either sex; age, 20–59 year), the group fed with UV-treated mushrooms showed an increase in the 25(OH)D2 level and a decrease in 25(OH)D3 level compared to the control group (received untreated mushrooms plus placebo capsule) [Citation33]. The authors also showed that the degree of the mean increase in serum 25(OH)D2 level in the treated groups was proportional to the magnitude of the decrease in serum 25(OH)D3 level. Lehmann et al. [Citation34] investigated the effects of vitamin D2 and D3 on the increase in the serum 25(OH)D2, 25(OH)D3, and total 25(OH)D levels in healthy volunteers fed with the same amount (50 μg/day) of vitamin D2 and D3, respectively. The authors observed that vitamin D2 lowered 25(OH)D3 level and suggested that the possibility of vitamin D2 interference in the hydroxylation of vitamin D3 should not be dismissed. In this study, comparison of the 25(OH)D3 level between each counterpart did not show any significant difference, indicating that the presence of ovaries had limited effect on 25(OH)D3. Along with other studies to confirm the bioavailability of vitamin D2 derived from mushrooms [Citation9,Citation25,Citation35], our results indicated that although vitamin D2 from shiitake mushroom decreased 25(OH)D3 level, it could elevate serum total 25(OH)D level.
Table 2. Serum vitamin D and calcium analyses.
In case of serum calcium we confirmed that the serum calcium level in the UV(O) groups was significantly lower than that in the other treated groups (). The drop in serum calcium level in the sham-UV(O) group was ~26% and ~23%, respectively, compared to the sham-con and sham-UV(X) groups. A similar decrease in the serum calcium level was observed in the OVX groups. The OVX-UV(O) group recorded a 7.5% and 2.6% decrease in the serum calcium level, compared to the OVX-con and OVX-UV(X) groups, respectively, indicating a significant difference when compared to the OVX-con group.
Initially, we thought that the decrease in calcium was due to bone mineralization. Jasinghe et al. [Citation9] also reported that the serum calcium level in rats, which received 1 μg of vitamin D2 daily, was significantly lower, compared to that in the control group after 4 weeks of experimentation. They speculated that active bone mineralization might have caused the decrease in serum calcium level, because the BMD of the group receiving vitamin D2 was higher than that of the base group. Similar results were found in another study, demonstrated that serum calcium level of the mice fed UV-pulsed oyster mushroom (Pleurotus ferulae) was significantly lower than that of sham and OVX control groups [Citation26]. We then revisited the reason why such decrease in calcium level took place in the vitamin D-fortified groups on the basis of the results of serum bone indicators, conducted at the end of the 10-week experimental period in all sham groups (data not shown). Among other indicators, N-terminal telopeptide of type I collagen (NTx) level of sham-UV(O) decreased significantly compared to other sham groups. As NTx is a bone resorption marker [Citation26], low rate of bone resorption might have caused low levels of serum calcium in the sham-UV(O) group. Thus, we speculate that the vitamin D2 from shiitake mushrooms inhibited calcium migration from the bone to the blood vessel.
A comparison of the sham and OVX groups that consumed identical diets showed, a significant decrease (P < 0.05) in the serum calcium level across all OVX groups. Similar outcome of the effect of estrogen deficiency on calcium absorption was also ascertained by Thomas et al. [Citation36]. In their study, serum level of OVX rats, fed the 0.5% Ca diet, was significantly lower than that of sham rats which fed the same diet. O’Loughlin and Morris discussed some adverse effects of the absence of the ovarian hormone on impaired calcium balance induced by an increase in intestinal calcium secretion in young rats [Citation37], and on the diminution of intestinal calcium absorption due to increased fecal calcium excretion [Citation38]. Thus, the underlying reason for the decrease in calcium absorption in the OVX group is likely to be the absence of estrogen.
When comparing calcium concentration between the control and UV(X) groups in both sham and OVX rats, we found that the calcium concentration in both UV(X) groups was slightly decreased, compared to both control groups. However, no significant difference (P > 0.05) was noted. Therefore, it is likely that shiitake mushroom itself was not effective in altering serum calcium level. Ovariectomy caused the expected decrease in E2 level. The E2 level in OVX-con group was less than the sensitivity of the assay (<3 pg/mL; ).
Femur BMD
The BMD of the proximal right femur was analyzed after 10 weeks of experiment period. shows the results for BMD determination in the sham and OVX groups. The BMD of the sham-UV(O) group was significantly higher (P < 0.05) than that of the sham-con and sham-UV(X) groups. Similar results were observed in the OVX groups, with the vitamin D2-supplemented group showing significantly higher (P < 0.05) BMD than the other two groups [i.e., OVX-con and OVX-UV(X)]. In a comparison between pair-fed groups, the BMD values of the sham-con and sham-UV(O) groups were significantly higher (P < 0.05) than those of the OVX-con and OVX-UV(O) groups, respectively. Our results were in good agreement with those of Kalu and Orhii who demonstrated that ovariectomy induced a significant decrease in trabecular bone mineral density and content [Citation39]. It is likely that estrogen played an important role in expressing high values of BMD and vitamin D2. Because there was no significant difference in the BMD of the control and UV(X) groups irrespective of the presence of ovaries, it seems that the components of shiitake have limited effects on BMD. Moreover, although insignificant, the BMD level of the OVX-UV(O) group was 950.84 ± 9.63, which was slightly higher than that of the sham-con (942.45 ± 8.57) and sham-UV(X) (947.83 ± 19.37) groups. These findings substantiated that the adverse effect of the absence of estrogen on BMD could be compensated to some extent by the vitamin D2 from shiitake mushroom. We confirmed that vitamin D2-fortified shiitake mushroom was effective in improving the BMD, without causing any adverse effects.
Trabecular and cortical bone analyses
The results of trabecular bone structure analyses along with the length and strength of femurs are shown in . The sham-UV(O) group showed a significant difference in the BS/BV and Tb.Th values compared to the sham group fed with the control diet. The BS/BV value of the sham-con group was increased by ~21%, compared to that of the sham-UV(O) group, whereas the Tb.Th value of the sham-con group was decreased by 13% compared to the sham-UV(O) group. No significant difference was noted in the other parameters due to vitamin D2 fortification. Although there was no significant difference, we found that the vitamin D2-fortified diet was effective in improving the trabecular structure of the femur by slightly increasing the BV/TV and Tb.N values and decreasing the Tb.Sp values, compared to the sham-con or sham-UV(X) groups. Among all trabecular bone parameters in the OVX groups, only Tb.Th showed a significant difference according to the diet type. The Tb.Th value of the OVX-UV(O) group was 5% and 6% higher than that of the OVX-con and OVX-UV(X) groups, respectively. The bone loss-inhibitory effect exerted by vitamin D2 in the OVX groups was marginal, compared to that in the sham groups. We speculated that the young age of the rats in this experiment, could be one of the reasons for this finding. According to Sims, a mature OVX rat is a suitable model for examining the bone loss caused by estrogen deficiency [Citation40]. We decided to select young female rats to avoid overlapping and adverse effects on the bone structure due to aging; however, the rats were too young to exhibit a significant difference in the bone structure after ovariectomy. Therefore, we concluded that using premature rats showed limited involvement of vitamin D2 activity in bone structure analysis, especially in the OVX group. The results of the length and strength of the left femurs are shown in . No significant difference was noted in the length and strength of the sham groups. Similar results were observed for all OVX groups. Moreover, there was no significant difference in the length and strength of the counterparts between the sham and OVX rats, indicating that the presence of the ovarian hormone did not affect length or strength of femur. Our results were consistent with those of previous studies [Citation41,Citation42], indicating that the cortical bone properties remain unchanged irrespective of varied vitamin D levels. Therefore, our present findings indicate that vitamin D2, obtained from shiitake mushroom, caused no difference in the cortical bone structure.
Table 3. Trabecular and cortical bone analyses.
Representative 3D images of the femurs are shown in . In a transversal tomogram of the sham groups ((a-c))), the sample from the sham-UV(O) showed less space and dense structure compared to the sample from the sham-con or sham-UV(X) groups. In the OVX groups ((d-f))), the samples from the OVX-UV(X) and OVX-UV(O) groups showed no virtual difference, while that from the OVX-con group showed less trabeculae with numerous perforations. This suggests that certain components from mushroom can induce denser and thicker trabecular bone conditions. A previous study had investigated the effect of UV-pulsed Pleurotus ferulae mushrooms on the bone health of OVX mice [Citation26]. For instance, UV-pulsed mushrooms significantly elevated the serum levels of all amino acids and metabolites related to energy metabolism in the treated group, compared to the OVX control group. Non-pulsed-mushroom also showed a significant increase in the taurine level. Taurine is known to be involved in bone metabolism, and in the induction of cell proliferation in human osteoblast cells [Citation43]. Therefore, several amino acids associated with bone activity, which are present in shiitake mushroom, may have caused such visual differences in the 3D images. The structural differences between the rats from the sham and OVX groups consuming the same diet were obvious. When the transversal tomograms of the counterparts fed with the same diet were compared, more space and loosened trabecular network were observed in those of the OVX groups. The tomogram result shows that the absence of estrogen is an underlying factor responsible for the a less compact trabecular bone structure in the OVX groups, in line with the significantly higher BS/BV and Tb.Sp values compared to the sham groups. The sagittal image ()), made it slightly more difficult to identify the effects of vitamin D2 on the trabecular bone architecture. However, the decrease in trabecular number and thickness was confirmed in groups lacking estrogen (d, e, and f), compared to the sham groups (a, b, and c). Although it was difficult to examine the exclusive effect of vitamin D2 through 3D images, we were able to investigate the effects of mushroom components and the presence of ovaries on bone density through micro-CT images.
Conclusion
Our results indicated that vitamin D2 from UV-irradiated shiitake mushrooms was well absorbed in the rats, as serum total 25(OH)D level was remarkably raised compared to the control or UV(X) groups, when vitamin D2 was consumed consistently near the recommended daily allowance (i.e. 9.60 μg). Moreover, it was clear that vitamin D2 from shiitake mushroom was also effective in trabecular bone architecture, since BMD and Tb.Th were significantly higher than those of control groups in both sham and OVX groups. There were adverse effects on trabecular bone structure as well as bioavailability of vitamin D and calcium due to the absence of estrogen. Although there was a decrease in serum total 25(OH)D, calcium level, and other parameters that are indicative of trabecular bone structures including BMD, vitamin D2-fortified shiitake mushroom might help retard bone loss, which can be accelerated by the absence of estrogen after menopause.
Acknowledgment
This work was supported by a grant from the Institute of Biomedical Science & Food Safety and the School of Life Sciences and Biotechnology for BK21PLUS, Korea University and High Value-added Food Technology Development Program, funded by the Ministry of Agriculture, Food and Rural Affairs (MAFRA) (114021021HD020).
Disclosure statement
No potential conflict of interest was reported by the authors.
References
- Holick MF, Vitamin D. A millenium perspective. J Cell Biochem. 2003;88(2):296–307.
- Christakos S, Dhawan P, Porta A, et al. Vitamin D and intestinal calcium absorption. Mol Cell Endocrinol. 2011;347(1–2):25–29.
- Wang Y, Wactawski-Wende J, Sucheston-Campbell LE, et al. The influence of genetic susceptibility and calcium plus vitamin D supplementation on fracture risk. Am J Clin Nutr. 2017;105(4):970–979.
- Holick MF. Resurrection of vitamin D deficiency and rickets. J Clin Invest. 2006;116(8):2062–2072.
- Kaushik R, Sachdeva B, Arora S, et al. Bioavailability of vitamin D(2) and calcium from fortified milk. Food Chem. 2014;147:307–311.
- Holick MF. The vitamin D deficiency pandemic and consequences for nonskeletal health: mechanisms of action. Mol Aspects Med. 2008;29(6):361–368.
- Holick MF, Chen TC. Vitamin D deficiency: a worldwide problem with health consequences. Am J Clin Nutr. 2008;87(4):1080S–1086S.
- Chailurkit LO, Kruavit A, Rajatanavin R. Vitamin D status and bone health in healthy Thai elderly women. Nutrition. 2011;27(2):160–164.
- Jasinghe VJ, Perera CO, Barlow PJ. Bioavailability of vitamin D2 from irradiated mushrooms: an in vivo study. Br J Nutr. 2005;93(6):951–955.
- Jesudason D, Need AG, Horowitz M, et al. Relationship between serum 25-hydroxyvitamin D and bone resorption markers in vitamin D insufficiency. Bone. 2002;31(5):626–630.
- de Paula FJ, Rosen CJ. Vitamin D safety and requirements. Arch Biochem Biophys. 2012;523(1):64–72.
- Bartl R, Frisch B. Definition of osteoporosis. Osteoporosis: Springer; 2004.
- Chen H, Zhou X, Fujita H, et al. Age-related changes in trabecular and cortical bone microstructure. Int J Endocrinol. 2013;2013:213–234.
- Roudebush RE, Magee DE, Benslay DN, et al. Effect of weight manipulation on bone loss due to ovariectomy and the protective effects of estrogen in the rat. Calcif Tissue Int. 1993;53(1):61–64.
- Chonan O, Matsumoto K, Watanuki M. Effect of Galactooligosaccharides on Calcium-Absorption and Preventing Bone Loss in Ovariectomized Rats. Biosci Biotech Bioch. 1995;59(2):236–239.
- Srivastava S, Toraldo G, Weitzmann MN, et al. Estrogen decreases osteoclast formation by down-regulating receptor activator of NF-kappa B ligand (RANKL)-induced JNK activation. J Biol Chem. 2001;276(12):8836–8840.
- Zhao X, Wu ZX, Zhang Y, et al. Anti-osteoporosis activity of Cibotium barometz extract on ovariectomy-induced bone loss in rats. J Ethnopharmacol. 2011;137(3):1083–1088.
- Wang J, He M, Wang G, et al. Organic gallium treatment improves osteoporotic fracture healing through affecting the OPG/RANKL ratio and expression of serum inflammatory cytokines in ovariectomized rats. Biol Trace Elem Res. 2017;183(2):270–279.
- Kalu DN. The ovariectomized rat model of postmenopausal bone loss. Bone Miner. 1991;15(3):175–191.
- Saif A, Wende K, Lindequist U. In vitro bone inducing effects of Lentinula edodes (shiitake) water extract on human osteoblastic cell cultures. Nat Prod Bioprospect. 2013;3(6):282–287.
- Gu YH, Belury MA. Selective induction of apoptosis in murine skin carcinoma cells (CH72) by an ethanol extract of Lentinula edodes. Cancer Lett. 2005;220(1):21–28.
- Kim MJ, Lee J, Lee JS. Effect of ultraviolet‐B irradiation on antioxidative properties of aqueous extracts from shiitake (Lentinus edodes) mushrooms. Int J Food Sci Tech. 2014;49(10):2276–2282.
- Spim SRV, de Oliveira B, Leite FG, et al. Effects of Lentinula edodes consumption on biochemical, hematologic and oxidative stress parameters in rats receiving high-fat diet. Eur J Nutr. 2017;56(7):2255–2264.
- Jasinghe VJ, Perera CO. Distribution of ergosterol in different tissues of mushrooms and its effect on the conversion of ergosterol to vitamin D2 by UV irradiation. Food Chem. 2014;92(3):541–546.
- Calvo MS, Babu US, Garthoff LH, et al. Vitamin D2 from light-exposed edible mushrooms is safe, bioavailable and effectively supports bone growth in rats. Osteoporos Int. 2013;24(1):197–207.
- Chen SY, Yu HT, Kao JP, et al. Consumption of vitamin D 2 enhanced mushrooms is associated with improved bone health. J Nutr Biochem. 2015;26(7):696–703.
- Won DJ, Kim SY, Jang CH, et al. Optimization of UV irradiation conditions for the vitamin D2-fortified shiitake mushroom (Lentinula edodes) using response surface methodology. Food Sci Biotechnol. 2018;27(2):417–424.
- Ko JA, Lee BH, Lee JS, et al. Effect of UV-B exposure on the concentration of vitamin D2 in sliced shiitake mushroom (Lentinus edodes) and white button mushroom (Agaricus bisporus). J Agr Food Chem. 2008;56(10):3671–3674.
- Dang ZC, van Bezooijen RL, Karperien M, et al. Exposure of KS483 cells to estrogen enhances osteogenesis and inhibits adipogenesis. J Bone Miner Res. 2002;17(3):394–405.
- Sung MJ, Davaatseren M, Hur HJ, et al. Antiosteoporotic activity of saururus chinensis extract in ovariectomized rats. Phytother Res. 2012;26(8):1182–1188.
- Underwood JL, DeLuca HF. Vitamin D is not directly necessary for bone growth and mineralization. Am J Physiol-Endoc M. 1984;246(6):E493–E498.
- Ross AC, Manson JE, Abrams SA, et al. The 2011 report on dietary reference intakes for calcium and vitamin D from the institute of medicine: what clinicians need to know. J Clin Endocr Metab. 2011;96(1):53–58.
- Stephensen CB, Zerofsky M, Burnett DJ, et al. Ergocalciferol from mushrooms or supplements consumed with a standard meal increases 25-hydroxyergocalciferol but decreases 25-hydroxycholecalciferol in the serum of healthy adults. J Nutr. 2012;142(7):1246–1252.
- Lehmann U, Hirche F, Stangl GI, et al. Bioavailability of vitamin D2 and D3 in healthy volunteers - a Randomized trial. Ann Nutr Metab. 2013;98(11):4339–4345.
- Koyyalamudi SR, Jeong SC, Song CH, et al. Vitamin D2 formation and bioavailability from agaricus bisporus button mushrooms treated with ultraviolet irradiation. J Agr Food Chem. 2009;57(8):3351–3355.
- Thomas ML, Simmons DJ, Kidder L, et al. Calcium metabolism and bone mineralization in female rats fed diets marginally sufficient in calcium: effects of increased dietary calcium intake. Bone Miner. 1991;12(1):1–14.
- O’Loughlin PD, Morris HA. Oophorectomy in young rats impairs calcium balance by increasing intestinal calcium secretion. J Nutr. 1994;124(5):726–731.
- O’Loughlin PD, Morris HA. Oestrogen deficiency impairs intestinal calcium absorption in the rat. J Physiol. 1998;511(1):313–322.
- Kalu DN, Orhii PB. Calcium absorption and bone loss in ovariectomized rats fed varying levels of dietary calcium. Calcif Tissue Int. 1999;65(1):73–77.
- Sims NA, Morris HA, Moore RJ, et al. Increased bone resorption precedes increased bone formation in the ovariectomized rat. Calcif Tissue Int. 1996;59(2):121–127.
- Lee AM, Anderson PH, Sawyer RK, et al. Discordant effects of vitamin D deficiency in trabecular and cortical bone architecture and strength in growing rodents. J Steroid Biochem Mol Biol. 2010;121(1–2):284–287.
- Kaastad TS, Reikeras O, Halvorsen V, et al. Vitamin D deficiency and ovariectomy reduced the strength of the femoral neck in rats. Calcif Tissue Int. 2001;69(2):102–108.
- Jeon SH, Lee MY, Kim SJ, et al. Taurine increases cell proliferation and generates an increase in [Mg2+]i accompanied by ERK 1/2 activation in human osteoblast cells. FEBS Lett. 2007;581(30):5929–5934.