ABSTRACT
MicroRNAs (miRNAs) regulate the development and growth cycle of hair follicles (HFs). The molecular mechanism by which miRNAs determine the development of HFs in the sheep foetus remains elusive. In this study, the expression profiles of miRNAs at 11 development periods (45, 55, 65, 75, 85, 95, 105, 115, 125, 135 and 145 d) in sheep foetus skin were analysed by high-throughput sequencing and bioinformatics analysis. A total of 72 conserved miRNAs, 44 novel miRNAs and 32 known miRNAs were significantly differentially expressed. qRT-PCR results for 18 miRNAs were consistent with the sequencing data. 85 d of foetal development was the starting point for secondary hair follicle (SF) development according to tissue morphology and cluster analysis. In SF development, the prolactin signalling pathway and platelet activation played important roles, and 10 miRNAs were potential candidate miRNAs in SF initiation.
Graphical abstract
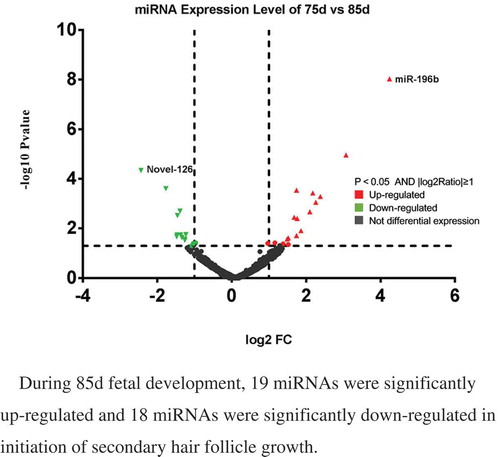
During 85d fetal development, 19 miRNAs were significantly up-regulated and 18 miRNAs were significantly down-regulated in initiation of secondary hair follicle growth
Hair follicles (HFs) are small but complex and are composed of 8 unique cell populations that are derived from the ectoderm and mesoderm. Thickening and elongation of epidermal keratino`cytes (hair placodes) occur in HFs [Citation1], and several studies have suggested that HFs consist of a hair bulb, inner root sheath (IRS), hair shaft, outer root sheath, and connective tissue sheath [Citation2,Citation3]. HFoffine-wool sheep is comprised ofprimary hair follicles (PFs) and secondary hair follicles (SFs) [Citation4–Citation6], PFs occur early, whereas SFs occur late [Citation7]. The growth and development of SFs directly influence the quantity and quality of wool produced [Citation8,Citation9]. SFs re-differentiation is the most important factor affecting HF density, which can be effectively increased thus improving the fineness of wool. The function and gene expression characteristics of HFs are distinct [Citation10]. Initiation is the first and the most important stage of HF morphogenesis. Dermal cells send the initial signals to induce the formation of hair buds in the epidermis, followed by the release of TGF-β, EDA/EDAR/NF-κB, Noggin/Lef-1, shh,BMP-2/4/7,β-catenin and FGF in the hair buds to induce dermal fibrogenic cells to form the dermal papillae that regulate the activity of keratinocytes. The cells of dermal papillae release a second signal to stimulate epithelial cell proliferation, which initiates differentiation and results in a complete HF structure [Citation11–Citation13]. However, the precise timing of these processes and the molecular mechanism underlying HF initiation remain elusive.
Previous studies have primarily focused on gene function, whilethe roles of noncoding RNAs (such as long noncoding RNA, circular RNA, and microRNAs) at various stages have received increasing attentions.MicroRNAs (miRNAs) are a type of genetic material with approximately 22 bases in length and are in a class of endogenous, small, single-stranded noncoding RNA molecules. They play important roles in diverse biological processes by regulating posttranscriptional gene expression via pairing to the 3ʹ-untranslated regions (3ʹUTRs) of target mRNAs [Citation14,Citation15] and have a broad range of biological functions. Many studies have demonstrated that miRNAs play important roles in the regulation of HF development [Citation16]. Yue et al.analysedthe roles of long noncoding RNA and coding RNA expression in sheep (Ovisaries) skin during the initiation of SF development by RNA sequencing (RNA-Seq) analysisandfound that the PPAR pathway likely plays a significant role during the initiation of SF development [Citation17]. Liu et al.studied areas of the skin that produce cashmere during anagen using Solexa sequencing and showed that the miRNAswereco-expressed in goats and sheep andlocated in the same region of the respective chromosomes, they may play essential roles in skin and follicle development [Citation18].
The Merino breed of sheep is prized for its wool, which hasthe finest and softest sheep wool.The Chinese Merino sheep (Junken Type) exhibit a high yield of quality wool with a fibre diameter (FD) of17.0–19.0μm. This study aimed to elucidate the regulatory mechanismof miRNA on Chinese Merino SF development in foetal period, which would benefit the cultivation of sheep with high-quality fine wool.
Materials and methods
Ethics statement
This study was conducted in strict compliance with the Guidelines for the Care and Use of Experimental Animals established by the Ministry of Science and Technology of the People’s Republic of China (approval number:2006–398) and theAnimal Care Guidelines and Regulationsfor the Administration of Laboratory Animals (2013 Revision) of the State Council of the People’s Republic of China, and it was approved by the Biological Studies Animal Care and Use Committee of Shihezi University, Xinjiang Academy of Agricultural and Reclamation Science. All efforts were made to minimize animal suffering.
Experimental animals and sample collection
A total of 50 2.5-year-old multiparous ewes ofthe super-fine-wool strainof Chinese Merino sheep (Junken Type)from the sheep farm of the Xinjiang Academy of Agricultural and Reclamation Science were used in this study.The sheep had an average wool FD of 17.5 ± 0.5μm according to the fibre testing standards of China and the International Wool Textile Organization (IWTO). All the ewes were sourced from a single flock and fed under the same conditions, and the oestrus time of eachwas determined using test rams. According to the pedigree information, the ewes were mated with the same strain of ram (FD = 16.6μm).The day of breeding was designated as d 0, and the gestational age of the foetuses was accurately recorded. A total of 33 foetuses were carefully, surgically removed from uteruses at gestational ages of 45d (period 1), 55d (period 2), 65d (period 3), 75d (period 4), 85d (period 5), 95d (period 6), 105d (period 7), 115d (period 8), 125d (period 9), 135d (period 10) and 145d (period 11); there were three replicates foreach time point. The foetuses were washed in phosphate-buffered saline and exsanguinated, and 1 cm2 skin strips were rapidly removed from both mid-sides of each foetusand snap frozen in liquid nitrogen for RNA extraction. The remainder of each foetus was placed in 4% paraformaldehyde for morphological observation.
Paraffin section fabrication
The skin tissues of the foetuses were treated according to the conventional paraffin-embedded section preparation method. After dressing, the fixed skin was washed with PBS, dehydrated in concentrated ethanol, made transparent with xylene, and embedded in paraffin, and the embedded wax block was fixed on a slicer and continuously sectioned at a thickness of 7μm. Sections were removed with a glass slide, dried at 37°C, H.E. stained with a neutral resin and observed under a microscope; representative images were captured.
Total RNA extraction and small RNA library preparation
Total RNA was isolated from foetal skins using a TruSeq Small RNA Sample Prep Kit (Illumina, San Diego, CA, USA) according to the manufacturer’s protocol. Equal volumes of total RNA were detected by 1% formaldehyde denaturing agarose gel electrophoresis. The bands were clearly visible.Total RNA purity was quantified using an ND 1000 Nanodrop Spectrophotometer (Thermo Scientific, Wilmington, USA), and RNA integrity was verified using an Agilent 2100 Bioanalyzerwithan RNA 6000 Nano Chip (Agilent Technologies, Santa Clara, USA). The OD260/OD280 ratios were greater than 1.8, and the RIN values were greater than 8. The initial total amount of RNA was accurately quantified with a Qubit RNA Assay Kit (Invitrogen, Carlsbad, USA).Ligated 3ʹ and 5ʹ adapters from a TruSeq Small RNA Library Prep Kit (Illumina, San Diego, CA, USA) were ligated at both ends of the small RNAs in two separated reactions using T4 RNA ligase 2 truncated with ATP (NEB, Beijing, China). The reverse transcription (RT) reaction was performed using SuperScript II Reverse Transcriptase, and the cDNA was amplified with PCR master mix according to the manufacturer’s instructions. The libraries were diluted before being runon a High-Sensitivity DNA Chip (Agilent Technologies, Santa Clara, USA), purified by 6% PAGE and quantified with a Qubit DNA HS Assay Kit (Invitrogen, Carlsbad, USA).Library quality was assessed using an Agilent 2100 Bioanalyzer High-Sensitivity DNA Chip and quantified using KAPA SYBR FAST Master Mix Universal 2X qPCR Master Mix anda DNA Quantification Standards and Primer Premix Kit (KAPA Biosystem, Massachusetts, USA).The libraries were adjusted to a concentration of 2nM for sequencing witha HiSeq2500 System (Illumina, San Diego,CA,USA) following the manufacturer’s protocol.
Small RNA high-throughput sequencing and processing of reads
Total RNA was isolated from the skins of 33 fine-wool sheep foetuses, and equal quantities of three samples from the same developmental period were pooled and prepared according to the Small RNA Sample Prep Kit protocol. Illumina small RNA deep sequencing was performed at the Beijing CapitalBioCo.Ltd. Total RNA at approximately 2nM was providedfor each sample set, and individual sequence reads with base quality scores were produced by Illumina sequencing. Adapter sequences were removed from both ends of the reads, and all identical sequences were counted and eliminated from the initial data set. The resulting set of unique sequences, with their associated read counts, was referred to using the sequence tags. The unique reads were mapped onto the O. ariesgenome from Ensembl (http://ensembl.org) using the programBowtie [Citation19].
Differential expression analysis and hierarchical clustering of microRNAs
Similar to the credibility interval approaches for analysing SAGE data [Citation20], we employed edger to identify miRNAs showing statistically significant differences in relative abundance (as reflected by the total count of individual sequence reads) among 11 small RNA libraries [Citation21]. Finally, miRNAs with a P-value<0.05 were considered to be significantly different among the 11 libraries. Differential miRNA TPM (transcripts per million) values were used to representthe level of expression differentiation among HFs at different foetal ages.
Hierarchical clustering analysis was performed with MEV software v4.6, and differently coloured regions were used to represent different clustering groups. Development and expression patterns within the same group were similar, possibly indicating similar functions or participation in the same biological processes.
Prediction of target genes for differential expression of microRNAs and functional analysis
A search for miRNA target genes was then performed using apreviously described approach. All newly identified miRNA sequences were used to query the 3ʹUTRs of O. aries for potential target sequences using RNAhybrid software with default parameters and permitting anenergy threshold of −20 kcal/mol. To determine the function of differentially expressed miRNAs in HF development, we subsequently analysed 3,223 genes through the DAVID website (http://david.abcc.ncifcrf.gov/). Furthermore, Gene Ontology (GO) annotation and Kyoto Encyclopedia of Genes and Genomes (KEGG) pathway annotation were conducted using the DAVID website.The significance threshold was set at a P-value<0.05.
Validation of small RNA sequencing by real-time PCR
The expression patterns of miRNA variedamong the eleven small RNA libraries constructed from foetal skin samples taken duringHF development. Eighteen miRNAs were randomly selected to confirm differential expression by quantitative real-time RT-PCR (qRT-PCR). Qualitative assessments of the purified RNAs were determined utilizing an ImplenNanophotometer UV Spectrophotometer (Germany) and gel electrophoresis, and total RNA was then reverse-transcribed using anmiScript II RT Kit (Qiagen,Germany). First-strand cDNA was synthesized from total RNA (2 μg of each sample)according to the manufacturer’s instructions, and the subsequent cDNA was used as a template for qRT-PCR, which was performed with a QuantiFast SYBR Green PCR Kit (Qiagen, Germany) on a LightCycler 480II Detection System (Roche, Germany). The PCR amplification was conducted in the thermal cycler using 20 μL of reaction mixture composed of 10 μL of 2x QuantiTect SYBR Green PCR Master Mix, 2 μL of 10x miScript Universal Primer, 2 μL of 10x miScript Primer Assay, and 2 μg of cDNA template. The PCR conditions were as follows: initial denaturation at 95°C for 5 min followed by 40 cycles at 98°C for 10 s, annealing at 57°C for 5 s (different miRNAs were slightly different), and extension at 72°C for 20 s. A melting curve was generated to confirm the identity of each PCR product. The entire experiment was performed three times for each developmental period, andeach sample was analysed three times. Expression was normalized to that of 5S, an internal housekeeping control. The primers used in theqRT-PCR are shown in . The miRNA downstreamprimer was the same universal primer as in the QuantiFast SYBR Green PCR kit(Qiagen,Germany); therefore, the table only shows the upstream primers.
Table 1. The primers of real-time PCR.
Statistical analyses
For gene expression analysis, the number of unique-match reads was calculated and normalized to TPM values, and 18 differentially expressed miRNAs were selected according to the sequencing results. Gene expression was calculated and normalized to the 5Sexpression level, and the relative expression level of the target miRNA was calculated using the 2−ΔΔCt method. The statistical software package SPSS17.0 was used to calculate the Ct values and standard errors among replicate samples. The expression levels of miRNAs in different developmental periods were compared using ANOVA.
Results
Morphological changesin hair follicles
The development of HFs was investigated by observing tissue sections. During the process of foetalHF development, epithelial cells and dermal cells initially interacted to form the primitive HF body, followed by the gradual growth and development of the complete HF structure.At 75d, the hair germ cells (a1) and dermal fibroblasts (a2) appeared in the foetal skin ()). At 85d, SFs began to appear, and SFsfurther developed from the enlarged portion of the epidermal layer of the PFs ()).
Figure 1. Variation in process of secondary hair follicle morphogenesis in Chinese Merino sheep during the foetal period.
A: 75 days of foetal development (100×); B: 85 days of foetal development (100×) a1: Hair germ cells, a2: Dermal fibroblasts, PF: Primary hair follicle, SF: Secondary hair follicle
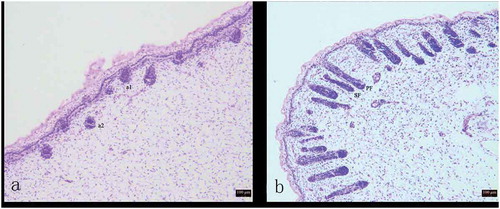
Overview of the Solexa sequencing data
All sequencing data for each small RNA library are presented in . We mapped these clean reads using the sheep (O. aries) genome from Ensembl(http://ensembl.org) to further elucidate the possible mechanisms underlying the diversity of small RNAs involved in HF development. Mapping the total reads to parts of the genomeresulted in 77.4% of the clean reads in the eleven libraries (), and the distribution of small RNA length differedamong the eleven libraries. In all libraries, most of the clean reads (78.03%) were 22 nucleotides long ().
Table 2. Small RNA sequence statistics for 11 libraries.
Table 3. Expression Profile of Sequenced Reads in 11 libraries.
Standard bioinformatics analysis was used to annotate the clean reads into different categories, including miRNA, known miRNA(69.1%), rRNA(2.5%), tRNA(4.0%), snoRNA(0.2%), and other RNA(0.4%). To identify the known miRNAs, unique sequences were aligned with all animal precursor and mature miRNA sequences from miRBase 18.0 (http://mirbase.org/), and the conservation of known miRNAs wasthen compared with those ofthe other species [Citation22].
Identification of differentially expressed miRNAsamong different hair follicle development phases
The biogenesis of miRNAs is highly regulated during various time periods. Thus, comparing differentially expressed miRNAsamong libraries provides insight into the molecular events related to the developmental periods of HFs. We first normalized the readsand then used aP-value<0.05 and an absolute log2 ratio value ≥1.0 as thresholds to determine the statistical significance of differences in miRNA expression.The miRNAs that satisfied the criteria were denoted as differentially expressed miRNAs.Wehave listed the top ten most abundantly expressedknown miRNAs (), conserved miRNAs () and novel miRNAs ().Among these miRNAs, oar-miR-10b had the highest expression ofthe known miRNAs, and miR-27 and Novel-14 had the highest expression ofthe conserved and novel miRNAs, respectively.
Table 4. The 10 most abundantly expressed known miRNAs in the skin and hair follicles.
Table 5. The 10 most abundantly expressed conversed miRNAs in the skin and hair follicles.
Table 6. The 10 most abundantly expressed novel miRNA in the skin and hair follicles.
For the critical SF developmentperiod from 75–85 d, wehave listed the top ten most differentially expressed known miRNAs (), conserved miRNAs () and novel miRNAs (). Six hundred seventy-nine (87.7%) miRNAswere co-expressed from 75–85 d; 61 (7.9%) were expressed at 75d; and 34 (85%) were expressed at 85d. For the differentially expressed miRNAs, 138 (95.8%) were co-expressed from 75–85 d; 4 (2.8%) were onlyexpressed at 75d; and 2 were expressed at 85d (1.4%). Four miRNAs were exclusively differentially expressed in the 75-d-library, namely, miR-146a, miR-196b*, miR-9*-1, and Novel-118*, and 2 miRNAs were exclusively differentially expressed in the 85-d-library, namely, miR-122 and miR-2285ak. A total of 37 miRNAs in sheep skin were differentially expressed between 75 and 85d of SF morphogenesis, among whichthe expression of 19 miRNAs was upregulated and that of 18 miRNAs was downregulated (). Novel-126 showed the most significant downregulation in expression during SF development, whereas miR-196b showed the most significant upregulation.
Table 7. The 10 most abundantly expressed known miRNA in 75d and 85d of sheep hair follicles development.
Table 8. The 10 most abundantly expressed conversed miRNA in 75d and 85d of sheep hair follicles development.
Table 9. The 10 most abundantly expressed novel miRNA in 75d and 85d of sheep hair follicles development.
Hierarchical cluster analysis
To better understand the expression patterns of miRNAs during HF development, a cluster analysis was performed using MEV software v4.6 (), and thedifferentially expressed miRNAs in 11 developmental periods were clustered into 2 categories and 4 subcategories.The development of PFs and SFs occurred from 45-95d.During this period, Primary hair follicle primordia was formed at first, then secondary hair follicles began to develop with primary hair follicles developing into the dermis at the embryonic age of 85 days Based on the foetuses at 95d, HFs continued to extend into the deep dermis withincreased age, gradually becoming longer and thicker. Theother categorywas 105-145d, during which mature sweat glands formed, most of the PFs and some of the SFs matured, and HFs formed keratinized hair shafts that penetrated the body surface. The four subcategories were as follows:the firstincluded 45, 55, and 65d of development, with 65dbeing the critical period for PF development;the second included 75, 85, and 95d of development, with 85d being the critical period for SF development;the third included 105, 115, and 125d of development; and the fourth included 135 and 145d of development.Overall, the cluster analysis results were consistentwith the critical stages for HF growth and development, which showedthat ourestablishedexperimental periods are meaningful.
Predictive analysis of target genes of differentially expressed miRNAs
To understand the functions of differentially expressed miRNAs in hair follicle development, we predicted the target genes of 148 differentially expressed miRNAs using RNAhybrid predictive software, and the results showed that 932 genes were targeted by the differentially expressed miRNAs in the 11 stages ofHF development (Supplementary Table S1).The target genes of 37differentially expressed miRNAs were predicted using RNAhybrid to annotate their biological functions during SFdevelopment at 75 and 85d, and253 target genes were found to functionin SF development (Supplementary Table S2).
Functional analysis of target genes
To better understand the biological process of HF and skin morphogenesis, 582 target genes of differentially expressed miRNAs were categorized into three GO categories: cellular component, biological process and molecular function. GO analysis was conducted on differentially expressed genes in the 11 developmental periods of sheep skin HF morphogenesis, and the results showed that 33 genes in the cellular component, biological process and molecular function categories had significant differences in expression during the 11 periods (). Enrichment analysis found 19 genes that were differentially expressed in the cellular component, biological process and molecular function categories at 75 and 85 d of SF morphogenesis (). There were 5 common GO terms in the 11 SF development periods, including positive regulation of receptor internalization, nucleoplasm, nucleotide binding, poly(A) RNA binding, and ATP binding.
Table 10. Gene ontology analysis of differentially expressed genes in 11 periods development of sheep skin HF morphogenesis.
Table 11. Gene ontology analysis of differentially expressed genes in sheep skin between 75d and 85d of secondary HF morphogenesis.
Because different genes can coordinate their biological functions, in vivo KEGG pathway-based analysis can further elucidate gene function. In this study, we further analysed genes through the DAVID website (http://david.abcc.ncifcrf.gov/), and the results showed that 15 pathways are potentially involved in the corresponding regulation of miRNAs (). For example, mitogen-activated protein kinase (MAPK) and AMPK pathways regulate cell proliferation and differentiation, and the AMPK signalling pathway was the most significantly enriched (P-value = 0.0441). Differentially expressed genes in KEGG biological pathways were primarily involved in the MAPK signalling pathway, prolactin (PRL) signalling pathway, and in platelet activation, among other processes, which previous studies have shown to possibly be associated with biological pathways involved in HF development. Additionally, we conducted KEGG analysis of the target genes of differentially expressed miRNAs in sheep skin between 75 and 85 d of SF morphogenesis (), and a total of three enriched pathways were identified, including the PRL signalling pathway (P-value = 0.0145), platelet activation (P-value = 0.0292) and osteoclast differentiation (P-value = 0.0301). There were 2 common KEGG pathways in the 11 SF development periods, the PRL signalling pathway and platelet activation, and the most significant difference was in the PRL signalling pathway. These results showed that the PRL signalling pathway and platelet activation play important roles in SF development at 85 d. Five PRL signalling pathway genes (SOCS3, FOXO3, STAT1, PIK3R1, and CISH) were significantly enriched at 75 d compared with their expression at 85 d (corrected P-value<0.05). The genes STAT1 (STAT), CISH (Cis), and SOCS3 (SOCS) were significantly upregulated, and the gene FOXO3 was significantly downregulated (). Six platelet activation genes (TLN2, FERMT3, RASGRP1, PIK3R1, PLA2G4D, and SYK) were significantly enriched at 75 d compared with their expression at 85 d (corrected P-value<0.05). The genes PLA2G4D (PLA), TLN2 (Talin), and FERMT3 (Kindlin3) were significantly upregulated, and the genes SYK, RASGRP1, and PIK3R1 (phosphatidylinositol-3-kinase, PI3K) were significantly downregulated (). The target genes of the PRL signalling pathway and of platelet activation in sheep skin between 75 and 85 d of SF morphogenesis corresponded to the following miRNAs: oar-miR-133, Novel-17, miR-196b*, miR-2285 l, Novel-8*, oar-miR376c-3p, miR-504, Novel-64, miR-873, and Novel-20 ().
Table 12. KEGG Pathway analysis of target genes of differentially expressed miRNA in 11 periods development of sheep skin HF morphogenesis.
Table 13. KEGG Pathway analysis of target genes of differentially expressed miRNA in sheep skin between 75d and 85d of secondary HF morphogenesis.
Table 14. Predicted target genes of prolactin signaling pathway and platelet activation in sheep skin between 75d and 85d of secondary HF morphogenesis.
Figure 5. Differentially expressed genes between 75 d and 85 d of secondary HF morphogenesis involved in prolactin signalling pathway.
Note: The red-labelled genes were significantly upregulated at 75 d compared with expression at 85 d (P-value<0.05). The blue-labelled genes were significantly downregulated at 75 d compared with expression at 85 d (P-value<0.05). The green-labelled genes were expressed in sheep skin during secondary HF initiation, but there were no significant differences.
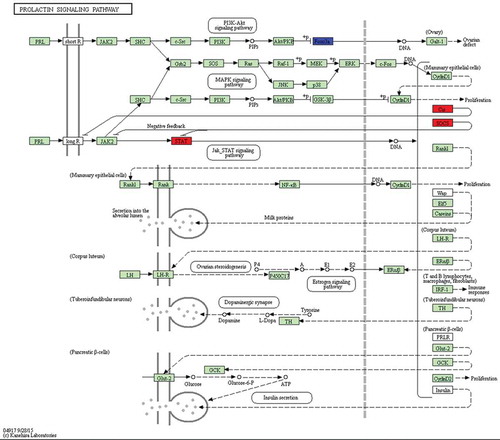
Figure 6. Differentially expressed genes between 75 d and 85 d of secondary HF morphogenesis involved in platelet activation.
Note: The red-labelled genes were significantly upregulated at 75 d compared with expression at 85 d (P-value<0.05). The blue-labelled genes were significantly downregulated at 75 d compared with expression at 85 d (P-value<0.05). The green-labelled genes were expressed in sheep skin during secondary HF initiation, but there were no significant differences.
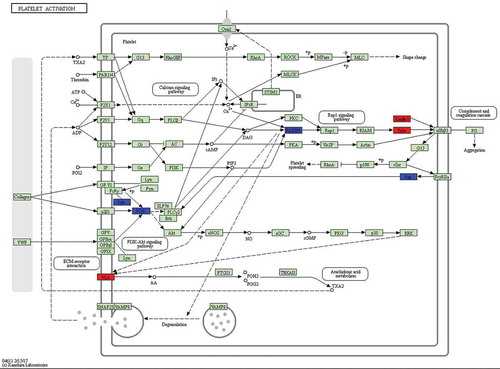
Validation of small RNA sequencing by quantitative real-time PCR (qRT-PCR)
To validate the accuracy and reproducibility of the miRNAome results, we chose to present data for 4 of the 10 miRNAs that were significantly differentially expressed (miR-184 Novel-14 miR-206, miR-767) (). The results of qRT-PCR analysis were partially consistent with the pattern shown by sequencing. miR-184 (1–2, 8–9), Novel-14 (5–6), miR-206 (1–2,2–3), miR-767 (3–4, 4–5, 8–9)did not show consistent expression between qRT-PCR and sequencing. The results for the rest of the miRNAs were consistent between qRT-PCR and sequencing analysis.
The results showed that the qRT-PCR results were highly consistent with those of the sequencing data. Hence, RNA-seq provided reliable data. To clarify the miRNA abundance during the 11 developmental stages, qRT-PCR was used to analyse the expression pattern of oar-miR-133 ().The expression level of this miRNA was highest at 85d (period 5), when it was significantly higher than duringother developmental periods (P-value<0.05), except for at 45d (period 1). The expression level of oar-miR-133 was significantly higher at 45 d (period 1), 55 d (period 2), 65 d (period 3), 75 d (period 4), 95 d (period 6), 105 d (period 7), and 125d (period 9) than at 115 d (period 8),135 d(period 10) and 145d (period 11; P-value<0.05). The results showed that oar-miR-133 plays an important role in SF morphogenesis at 85d.
Discussion
Wool is an important animal product and raw material for the textile industry. The quality of wool is determined by theFD, crimp, yield, colour, and staple strength, among other properties.The quality and commercial value of animal hair depend on the structure and characteristics of HFs, and SFs determine the yield and FD of fine wool. The HF is one of the most unique and complex micro-organs in mammals, andis the only organ that exhibits cylindrical growth.
According to previous studies, after approximately 50 d of embryonic development, the skin of sheep begins to form PFs, and the first SFs begin to appear at up to E85 of development. At the foetal age of 105 d, secondary sub-follicles begin to form, and by birth, all HFs are formed, and PFs are mature. However, SFs do not fully matureuntil4–5 months after birth [Citation23,Citation24]. Additionally, studies have reported that PF development is initiated in sheep at E60, with follicle maturation occurring from E120, whereas SF differentiation occurs from E120 to the formation of cashmere after birth (NB). The foetal development period for PFs is E84 in Chinese super-fine-wool sheep (Gansu type), but in ourstudy,histomorphological observations of the foetal skin of Junken type sheep showed that the development of SFs occurred at E85, suggesting that the starting point of SF development differs among breeds.
miRNAs are involved in a variety of processes related to epidermal morphology and HF growth and development in animals. Some new methods have been used to studymiRNAs, especially high-throughput sequencing techniques. Zhang found that miRNAs are important in the function and evolution of skin based on a high-throughput sequencing techniques [Citation25]. Li compared the miRNA transcriptomes of goat and sheep skin using Solexa sequencing to understand the development of skin and HFs and identified 1,910 known miRNAs and 2,261 novel mature miRNAs, of which 107 novel miRNAs and 1,246 known miRNAs were differentially expressed in the two libraries [Citation26]. Emerging evidence indicates that regulatedmiRNA expression is essential for normal HF development [Citation27–Citation29]. Yi found that epidermal and HF miRNA expression is required for normal skin development in mice [Citation30]. Mardaryev found that miRNA-31 expression in mouse HFs after the withdrawal period significantly increased with the administration of an antisense miR-31 inhibitory factor to the skin, which accelerated the development of HFs, cell differentiation and hair formation [Citation31]. Moreover, investigations of HFs have provide invaluable insights into the possible causes of disorders related to human hair [Citation32–Citation34].
In this study,the miRNA expression profiles in the skin of sheep foetuses at11 developmental periods (45, 55, 65, 75, 85, 95, 105, 115, 125, 135 and 145d) were assessed by high-throughput sequencing and bioinformatics analysis.A total of 507 conserved miRNAs, 179 novel miRNAs and 152 known miRNAs were detected.72 conserved miRNAs, 44 novel miRNAs and 32 known miRNAs were significantly differentially expressed. The miRNAs showing differential expressionin the 11 developmental periods were clustered into 2 categories and 4 subcategories; one category included 45–95d of development, and the other included 105-145d of development.The first subcategory included 45d, 55d and 65d of development. The second subcategory included 75d, 85d and 95d of development. The third subcategory included 105d, 115d and 125d of development. The fourth subcategory included135d and 145d of development.The results of the cluster analysisare consistent with the findings for wool follicle development.Upon further analysis, oar-miR-10b showed the highest expression at 85 d of SF development.The formation and periodic development of mammalian HFs is under molecular regulation and requires the control of complex networks involving different signalling pathways. Liu et al found that differentially expressed miRNAs might regulate wool follicle development by targeting genes in many different pathways, such as the MAPK and Wnt pathways, as well as the pathways that regulate the actin cytoskeleton, focal adhesion, and tight junctions,during the anagen, catagen and telogenphasesin Tibetan sheep [Citation35]. Zhang et al indicated that 7 miRNAs most likely target the genes in the Wnt/β-catenin, Shh/BMP and Notch pathways, which are important in feather morphogenesis. Based on our research, the AMPK and MAPK pathways, which are related to cell proliferation,differentiation and apoptosis,have an important link toHF development. KEGG pathway analysis showed that thetarget genes of the PRL signalling pathway and platelet activation were the most important among the differentially expressed miRNAs in SF morphogenesis.
Prolactin (PRL)has a direct effect on the division and proliferation of somatic cells [Citation36]. Cassy showedthat melatonin acts indirectly on HF growth via the effects of PRL combined with its receptor (PRLR) [Citation37]. PRL activates many signalling cascades, including the Jak2/Stat, which is the primary cascade, Src kinase, PI3K/AKT, and the MAPK pathways. Nixon et al. found that PRLR mRNA was most abundant in the dermal papilla, outer root sheath, hair germ, skin glands and epidermis. These cycle-related patterns of PRLR expression suggest dynamic regulation of PRLR by PRL that thereby modulates the hormonal responsiveness of seasonally growing HFs [Citation38]. McCloghry found that PRL, but not melatonin, may be involved in the regulation of derived SF development [Citation39]. Additionally, platelet activation and HFs are closely linked.Pietro found that platelet-rich plasma (PRP) has emerged as a new treatment modality in regenerative plastic surgery, and preliminary evidence suggests a beneficial role in hair regrowth [Citation40]. Raquel analysed the adult HF dermal stem cell niche and suggested that platelet-derived growth factor signalling promotes self-renewal, which is essential for maintaining the dermal pool of HF stem cellsand, ultimately, their regenerative capacity within the HF [Citation41]. In our study, the AMPK and MAPK pathways, which are related to cell proliferation, differentiation and apoptosis, [Citation42,Citation43] were found to have important links to HF development. The PRL signalling pathway and platelet activation have important rolesin SF morphogenesis. The genes SOCS3, FOXO3, STAT1, PIK3R1 and CISH were significantly enriched in the PRL signalling pathway (p < 0.05), and TLN2, FERMT3, RASGRP1, PIK3R1, PLA2G4D and SYK were significantly enriched in platelet activation (p < 0.05). Target genes in the PRL signalling pathway and of platelet activation corresponded to the following miRNAs: oar-miR-133, oar-miR-376c-3p, miR-196b*, miR-2285 l, miR-504, miR-873, Novel-8*, Novel-17, Novel-20 and Novel-64, which are candidate miRNAs for the initiation of SF development. In summary, our findings, combined with previous results, clearly indicated thatPRL and platelet activation have important roles in HF development. However, the mechanisms of action of signal transduction, apoptosis, nutrition and regulatory factors in HF development require further study.
Conclusions
E85 of foetaldevelopment is the starting point for SF development in Chinese Merino (Junken Type) sheep. High-throughput sequencingand bioinformatic analysis showed that a total of 507 conserved miRNAs, 179 novel miRNAs and 152 known miRNAs were detectedin 11skin expression profiles of sheep foetuses. A total of 37 differentially expressed miRNAs were detected in relation to SF morphogenesis. The PRL signalling pathway and platelet activation pathwaywereconfirmed to play important roles in SF development;oar-miR-133, oar-miR-376c-3p, miR-196b*, miR-2285 l, miR-504, miR-873, Novel-8*, Novel-17, Novel-20 and Novel-64 are potential candidate genes for further study of the molecular mechanisms underlying SF initiation.
Author Contributions
Hua Yang conceived and designed the study. Han-Yu-Lu Yang performed the experiments and wrote the manuscript. Hua Yang and Han-Yu-Lu Yang performed the statistical analysis and the data analysis. Xiao-Jun Liu,Min Shenand Jing–Quan Yangparticipated in performing the experiments. Guo-Qing Shi andYong-Lin Yangparticipated in the design of the study. All authors read and approved the final manuscript.
Supplementary_Table_S2-h.xlsx
Download MS Excel (26.5 KB)Supplementary_Table_S1-h.xlsx
Download MS Excel (78.9 KB)Disclosure statement
No potential conflict of interest was reported by the authors.
Supplementary material
Supplemental data for this article can be accessed here.
Additional information
Funding
References
- Paus R, Müller-Röver S, Van DVC, et al. A comprehensive guide for the recognition and classification of distinct stages of hair follicle morphogenesis. J Invest Dermatol. 1999;113:523–532.
- Sperling LC. Hair anatomy for the clinician. J Am Acad Dermatol. 1991;25:1–17.
- Stenn KS, Paus R. Controls of hair follicle cycling. Physiol Rev. 2001;81:449.
- Lyne AG. The development of the epidermis and hair canals in the merino sheep foetus. Aust J Biol Sci. 1957;10:390–397.
- Lyne AG, Hollis DE. The structure and development of the epidermis in sheep fetuses. J Ultrastruct Res. 1972;38:444–458.
- Mccloghry CE, Hollis DE, Raphael KA, et al. The effects of exogenous melatonin and prolactin on wool follicle development in ovine foetal skin grafts. J Agric Sci. 1993;121:247–253.
- Parry AL, Nixon AJ, Craven AJ, et al. The microanatomy, cell replication, and keratin gene expression of hair follicles during a photoperiod-lnduced growth cycle in sheep. Acta Anat. 1995;154:283–299.
- Gao Y, Wang X, Yan H, et al. Comparative transcriptome analysis of fetal skin reveals key genes related to hair follicle morphogenesis in cashmere goats. PLoS One. 2016;11:e0151118.
- Yu-Yu WU, Yue YJ, Guo TT, et al. Study on fetal skin hair follicle development and morphology of China super-fine merino (gansu type). Sci Agric Sin. 2013;46:1923–1931.
- Shimomura YC, Hristiano AM. Biology and genetics of hair. Ann Rev Genomics Hum Genet. 2010;11:109–132.
- Cadau S, Rosignoli C, Rhetore S, et al. Early stages of hair follicle development: a step by step microarray identity. Eur J Dermatol. 2013;23:4–10.
- Ohyama M, Zheng Y, Paus R, et al. The mesenchymal component of hair follicle neogenesis: background, methods and molecular characterization. Exp Dermatol. 2010;19:89–99.
- Sennett R, Rendl M. Mesenchymal–epithelial interactions during hair follicle morphogenesis and cycling. Semin Cell Dev Biol. 2012;23:917–927.
- Ghelani HS, Rachchh MA, Gokani RH. MicroRNAs as newer therapeutic targets: A big hope from a tiny player. J Pharmacol Pharmacotherapeutics. 2012;3:217–227.
- Wenguang Z, Jianghong W, Jinquan LY, et al. A subset of skin-expressed microRNAs with possible roles in goat and sheep hair growth based on expression profiling of mammalian microRNAs. OMICS. 2007;11:385.
- Alnuaimi Y, Baier G, Watson RE, et al. The cycling hair follicle as an ideal systems biology research model. Exp Dermatol. 2010;19:707.
- Yue Y, Guo T, Chao Y, et al. Integrated analysis of the roles of long noncoding RNA and coding RNA expression in sheep (Ovis aries) skin during initiation of secondary hair follicle. PLoS One. 2016;11:e0156890.
- Liu Z, Xiao H, Li H, et al. Identification of conserved and novel microRNAs in cashmere goat skin by deep sequencing. PLoS One. 2012;7:e50001.
- Langmead B, Trapnell C, Pop M, et al. Ultrafast and memory-efficient alignment of short DNA sequences to the human genome. Genome Biol. 2009;10:R25.
- Vêncio RZN, Brentani H, Pereira CAB. Using credibility intervals instead of hypothesis tests in SAGE analysis. Bioinformatics. 2003;19:2461–2464.
- Robinson MD, Mccarthy DJ, Smyth GK. edgeR: a bioconductor package for differential expression analysis of digital gene expression data. Bioinformatics. 2010;26:139–140.
- Lu T, Cui L, Zhou Y, et al. Transcriptome-wide investigation of circular RNAs in rice. Rna. 2015;21:2076–2087.
- Galbraith H. Fundamental hair follicle biology and fine fibre production in animals. Animal. 2010;4:1490–1509.
- Rogers GE. Biology of the wool follicle: an excursion into a unique tissue interaction system waiting to be re-discovered. Exp Dermatol. 2010;15:931–949.
- Zhang L, Nie Q, Su Y, et al. MicroRNA profile analysis on duck feather follicle and skin with high-throughput sequencing technology. Gene. 2013;519:77–81.
- Li J, Qu H, Jiang H, et al. Transcriptome-wide comparative analysis of microRNA profiles in the telogen skins of liaoning cashmere goats (Capra hircus) and fine-wool sheep (Ovis aries) by Solexa deep sequencing. DNA Cell Biol. 2016;35:696.
- Andl T, Murchison E, Liu F, et al. The miRNA-processing enzyme dicer is essential for the morphogenesis and maintenance of hair follicles. Curr Biol. 2006;16:1041–1049.
- Yi R, Fuchs E. MicroRNA-mediated control in the skin. Cell Death Differ. 2010;17:229–235.
- Yi R, Poy MN, Stoffel M, et al. A skin microRNA promotes differentiation by repressing ‘stemness’. Nature. 2008;452:225–229.
- Yi R, O’carroll D, Pasolli HA, et al. Morphogenesis in skin is governed by discrete sets of differentially expressed microRNAs. Nat Genet. 2006;38:356–362.
- Mardaryev AN, Ahmed MI, Vlahov NV, et al. Micro-RNA-31 controls hair cycle-associated changes in gene expression programs of the skin and hair follicle. FASEB J. 2010;24:3869–3881.
- Bostjancic E, Glavac D. Importance of microRNAs in skin morphogenesis and diseases. Acta Dermatovenerol Alp Pannonica Adriat. 2008;17:95.
- Duverger O, Morasso MI. To grow or not to grow: hair morphogenesis and human genetic hair disorders. Semin Cell Dev Biol. 2014;25–26:22–33.
- Rippa A, Terskikh V, Nesterova A, et al. Hair follicle morphogenesis and epidermal homeostasis in we/we wal/wal mice with postnatal alopecia. Histochem Cell Biol. 2015;143:481–496.
- Liu G, Liu R, Li Q, et al. Identification of microRNAs in wool follicles during anagen, catagen, and telogen phases in Tibetan sheep. Plos One. 2013;8:e77801.
- Kelly PA, Ali S, Rozakis M, et al. The growth hormone/prolactin receptor family - recent progress in hormone research. Recent Prog Horm Res. 1991;48:123.
- Cassy S, Charlier M, Bélair L, et al. Developmental expression and localization of the prolactin receptor (PRL-R) gene in ewe mammary gland during pregnancy and lactation: estimation of the ratio of the two forms of PRL-R messenger ribonucleic acid. Biol Reprod. 1998;58:1290–1296.
- Nixon AJ, Ford CA, Wildermoth JE, et al. Regulation of prolactin receptor expression in ovine skin in relation to circulating prolactin and wool follicle growth status. J Endocrinol. 2002;172:605–614.
- Mccloghry CE, Hollis DE, Foldes A, et al. The effects of exogenous melatonin and prolactin on wool follicle development in ovine foetal skin grafts. Aust J Agric Res. 1993;44:993–1002.
- Gentile P, Garcovich S, Bielli A, et al. The effect of platelet-rich plasma in hair regrowth: a randomized placebo-controlled trial. Stem Cells Transl Med. 2015;4:1317–1323.
- González R, Moffatt G, Hagner A, et al. Platelet-derived growth factor signaling modulates adult hair follicle dermal stem cell maintenance and self-renewal. NPJ Regen Med. 2017;2.
- Botchkareva NV, Ahluwalia G, Shander D. Apoptosis in the hair follicle. J Invest Dermatol. 2006;126:258–264.
- Folgueras AR, Guo X, Pasolli HA, et al. Architectural niche organization by LHX2 is linked to hair follicle stem cell function. Cell Stem Cell. 2013;13:314–327.