ABSTRACT
In this study, we examined the mechanism of Flavone of Hippophae (H-flavone) in regulating macrophage foaming and atherosclerosis (AS) plaque formation. H-flavone treatment increased the secretion of C1q/tumor necrosis factor-related proteins 6 (CTRP6) in Ox-LDL-treated mouse peripheral blood macrophage cells (PBMC) and significantly reduced the percentage of cholesteryl ester (CE) in PBMC. Additionally, H-flavone suppressed Ox-LDL-induced cell foaming and the production of inflammatory cytokines through upregulating CTPR6 expression. Next, we further validated the inhibitory effect of H-flavone on plaque formation and inflammation in a mouse AS model. A substantial reduction in the secretion of inflammatory cytokines was observed in apoE-/- mice by H-flavone. Immunohistochemistry and Oil Red O staining results showed that H-flavone suppressed macrophage infiltration and the development of AS plaque. These effects were more pronounced in early administration. Our results suggest that H-flavone effectively inhibits macrophage foaming, inflammation and vascular plaque formation by upregulating CTRP6 and may be used to reduce AS risk.
Graphical abstract

H-flavone suppresses macrophage foaming by upregulating CTPR6 in vitro. H-flavone inhibits macrophage infiltration and AS plaque development in vivo.
Atherosclerosis (AS) is a chronic inflammatory process of the arterial wall characterized by the cumulation of lipids and fibrous elements in the large arteries. A triggering event for this process is the accumulation of low-density lipoprotein (Ox-LDL) in which macrophages immersing in the endothelium, phagocytosing lipids, producing a number of proinflammatory molecules, and ultimately forming “foam cells”. The death of foam cells leads to plaque formation and even the onset of acute coronary syndrome (ACS) [Citation1,Citation2]. Several evidences reveal that macrophages are participated in modulating lipid metabolism and inflammatory responses. Their biological functions in vascular plaque formation play a key role in the occurrence and development of AS [Citation3,Citation4].
Recent studies have shown that novel metabolic regulators, the C1q/tumor necrosis factor-related proteins (CTRP1-15) are highly conserved adiponectin paralogs and suppress lipid accumulation and promote macrophage polarization toward an anti-inflammatory phenotype [Citation5–Citation7]. Specially, CTRP6 regulates adipogenesis [Citation8], fat oxidation [Citation9], fibrogenesis [Citation10] and induces the expression of IL-10 in monocytic cells [Citation11]. A recent study has suggested that CTRP9 exerted an atheroprotective effect by altering multiple pathological processes involved in AS, such as inflammation, foam cell formation, endothelial dysfunction and insulin resistance [Citation12]. Furthermore, CTRP9 could significantly reduce oxLDL-induced TNF-a and MCP-1 expression by suppressing NF-κB phosphorylation [Citation13]. However, the exact mechanism of CTRP6 regulating lipid metabolism of AS development and expression of inflammatory factors remains largely unknown.
Seabuckthorn (Hippophae rhamnoides L. Elaeagnaceae; SL) is a hardy bush, which is belonged to the Elaeagnaceae family and has unique medicinal value. SL contains various chemical compounds including tocopherols, carotenoids, anthocyanins lipids, ascorbic acid and flavonoids [Citation14]. Flavone of Hippophae (H-flavone) exhibits many beneficial properties. Studies have shown that H-flavone is capable of lowering the risk of cardiovascular diseases and cancers [Citation15,Citation16], as well as exerting antioxidant and immunoregulation properties [Citation17–Citation19]. A recent study showed that pentamethyl quercetin, a polymethoxylated flavonoid in SL induced adiponectin expression by activating PPARγ [Citation20]. The protective mechanism of H-flavone on AS remains unknown yet.
In view of the above, the present investigation was undertaken to evaluate the mechanism of H-flavone in regulating macrophage foaming, inflammation and vascular plaque formation in vivo and vitro. We provide here the first evidence that H-flavone would inhibits macrophage foaming and AS plaque formation by modulating the expression level of CTRP6.
Materials and methods
Cell culture and foam
Primary Mouse peripheral blood macrophage cells (PBMC) were isolated from buffy coats derived from healthy mice. Mouse PBMC were grown in RPMI-1640 medium (HyClone, Salt Lake City, UT) supplemented with 10% fetal bovine serum at a humidified incubator (7% CO2, 37°C). Logarithmic growth phase cells were seeded at a concentration of 5 × 105 cells/mL and treated with RPMI-1640 (containing 0.5% DMSO) medium containing 10 µg/mL ox-LDL (Solarbio, Beijing, China; no. H7950) for 24 h to induce foaming.
Plant material
Fruits and leaves of seabuckthorn were collected and dried under shade. The powdered leaves and whole fruits material were degreased with petroleum ether and extracted with 65% ethanol at the temperature of 80°C. The extraction was repeated 3 times (1 h for each time) and the ratio of material to solvent 1/8 (W/V). X-5 resins were used to refining flavone. The dilution of the extract was then carried out in sterile saline containing 0.1% Tween-80.
Flow cytometric analysis
Mouse PBMC were washed with PBS and incubated with anti-CD68 antibody (1:100, MCA1957, Bio-Rad, Hercules, CA, USA) for 30 min on ice. After rinsing with ice cold PBS for 3 times, cells were incubated with Alexa Fluor® 488 Conjugated Rat Anti-Mouse Antibodies (Invitrogen, Carlsbad, CA, USA) for 15 min. Flow cytometric measurements were performed with an Epics Elite flow cytometer (Beckman).
RNA extraction and quantitative Real-time transcription PCR (RT-qPCR)
Total RNA was extracted from the treated macrophages with TRIzol reagent (Invitrogen, Carlsbad, CA, U.S.A.). First-strand cDNA was synthesized from 4 µg of total RNA. The sequences for sense (S) and antisense (AS) primers are as follows: CTRP6-S, 5′-CCC TGC GTG GCA TCT ACT TC-3′, CTRP6-AS, 5′-GCC GCT GAA GGT GAT GTA GGT′. RT-qPCR was conducted using SYBR Premix Ex Taq™ (Takara, Dalian, China) at 95°C for 1 min, followed by 35 cycles of 95°C for 20 sec and 58°C for 1 min in the ABI StepOnePlus Real-time PCR system. The relative fold changes in mRNA expression were calculated using the 2−ΔΔCT method.
Animals and treatment
The apoE (apoE-/-) deficient mice can develop severe hypercholesterolemia and spontaneously form AS plaques with similar distribution and pathological features to human AS plaques. The apoE-/- mice were (n = 18) purchased from the Beijing Laboratory Animal Research Center (BLARC), China. This study was approved by the Ethics Committee of the First Affiliated Hospital of Xi’an Jiaotong University and carried out according to the guidelines of the Care and Use of Laboratory Animals. The mice were housed in an environmentally controlled room (20°C, 45–60% humidity, and a 12 h light/dark cycle) and were allowed free access to high fat diet (containing 21% fat and 0.15% cholesterol) and water. The mice were divided into three groups containing six mice each. After 6 weeks of birth, the mice were placed on the study diets. H-flavone was dissolved in sterile saline containing 0.1% Tween-80, the concentration of H-flavone was 75 mg/kg administered orally with the help of gastric canula. The experimental group was fed H-flavone (75 mg/kg/d) while the control group was maintained on saline containing 0.1% Tween-80. The early administration group began to administer H-flavone at 6 weeks of age for 6 weeks, and the late administration group was administered with H-flavone at 12 weeks of age for 6 weeks. The mice were sacrificed at 18 weeks of age, and the venous plexus blood was taken before the mice were sacrificed. The value of serum total cholesterol (TC), triglyceride (TG) and low-density lipoprotein (LDL) were measured by using blood biochemical automatic analyzer (Beckman).
Immunohistochemistry
Formalin-fixed, paraffin-embedded tissue-blocks of mice aortic roots were serially sectioned and the thickness was 6 µm. Sections were stained by immunohistochemistry (IHC). Immunohistochemical stains were performed by using anti-CD68 antibody (1:2000, MCA1957, Bio-Rad, Hercules, CA, USA).
Oil Red O staining
The mice aortic ascending, arch and thoracic aorta were longitudinally incised, fixed in 1% formalin, and stained with Oil Red O. Images were acquired using an inverted microscope (IX70, Olympus) and were scanned to calculate the percentage of damage area.
Enzyme-linked immunosorbent assay (ELISA)
Mouse PBMC were either untreated or exposed to 10 µg/mL Ox-LDL in the presence or absence of H-flavone. The apoE-/- mice were fed H-flavone in early and late stages. Protein levels of monocyte chemotactic protein-1(MCP-1; PMJE00, R&D Systems, Minneapolis, MN, USA), hypersensitive C-reactive protein (hs-CRP; DY1707, R&D Systems, Minneapolis, MN, USA), TNF-α (PMTA00B, R&D Systems, Minneapolis, MN, USA), IL-1β (PMLB00C, R&D Systems, Minneapolis, MN, USA) and IL-6 (PM6000B, R&D Systems, Minneapolis, MN, USA) in the media or serum were measured by ELISA kits following the manufacture’s protocol.
Statistical analysis
The data are expressed as means ± SEM and each experiment were performed in triplicate in this study. After the homogeneity test for variance, comparisons between groups were performed by one-way analysis of variance (ANOVA) using SPSS 22.0 software, and then post hoc test was determined by LSD test. A significant difference was indicated when the p-value < 0.05.
Result
Effects of H-flavone on mouse PBMC CTRP6 expression and lipid metabolism
To assess the role of H-flavone on CTRP6 expression and lipid metabolism in peripheral blood macrophage cells (PBMC), mouse PBMC were isolated from the blood of healthy mice and cultivated with RPMI-1640 to induce differentiation into mature macrophages, with the purity of which over 94.6% as determined by flow cytometry ()). Levels of CTRP6 in PBMC, Ox-LDL-induced macrophage cells, and cell culture supernatant were examined by RT-qPCR and ELISA. We found CTRP6 was reduced after PBMC were treated with Ox-LDL (). H-flavone dramatically increased CTRP6 expression when the additional amount of H-flavone was 0.2 mg/mL and 1.0 mg/mL (). The degree of cell foaming was measured by the percentage of cholesteryl ester (CE). After the induction of PMBC with 10 µg/mL Ox-LDL, the percentage of intracellular CE was increased ()), while H-flavone markedly attenuated this induction ()).
Figure 1. Effects of H-flavone on mouse PBMC CTRP6 expression and foaming. Primary mouse peripheral blood macrophages (PBMC) were treated with H-flavone (0.2mg/mL or 1 mg/mL), or Lipitor (40 µg/mL), in the presence of 10 µg/mL Ox-LDL. (a) The purity of mouse macrophage was evaluated by flow cytometric analysis using an antibody to CD68 (specific to macrophages). Real-Time reverse transcription PCR (RT-qPCR) (b) and enzyme-linked immunosorbent assay (ELISA) (c) was performed to assess the expression of CTRP6 in mouse PBMC, Ox-LDL-induced foam cells, and cell culture supernatant in the presence and absence of H-flavone. (d) The percentage of cholesterol ester (CE) was represented the degree of PBMC foaming. Lipitor is a drug for treatment of atherosclerosis as a positive control. The columns were presented as the mean ± SEM (n ≥ 3). *p < 0.05 (vs. untreated PBMC). #p < 0.05 (vs. PBMC treated with Ox-LDL alone). &p < 0.05 (vs. PBMC treated with Ox-LDL and 0.2 mg/mL H-flavone). N.S.p > 0.05 (vs. untreated PBMC).
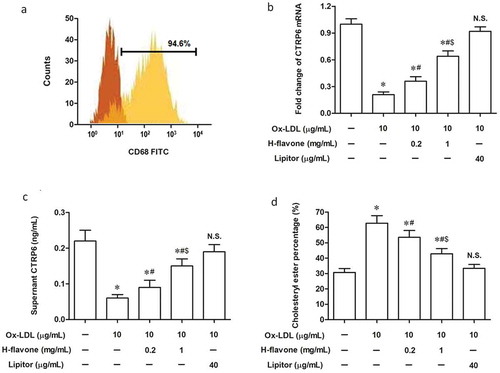
H-flavone inhibits the production of inflammatory cytokines
It has been recognized that H-flavone has immunomodulating activities [Citation21]. Thus, we further investigated the inflammatory cytokines in Ox-LDL-induced foam cell culture supernatant in the presence or absence of H-flavone. Secretion of pro-atherogenic factors, including TNF-α, MCP-1, IL-1β, and IL-6 were markedly amplified by Ox-LDL, whereas Ox-LDL-induced inflammatory cytokine production was significantly weakened after the treatment with H-flavone ().
Figure 2. H-flavone facilitates the production of inflammatory cytokines.
Primary mouse PBMC were treated with H-flavone (0.2mg/mL or 1 mg/mL), or Lipitor (40 µg/mL), in the presence of 10 µg/mL Ox-LDL. Protein levels of TNF-α (a), MCP-1 (b), IL-1β (c), and IL-6 (d) were detected by enzyme-linked immunosorbent assay (ELISA). Lipitor is a drug for treatment of atherosclerosis as a positive control. The columns were presented as the mean ± SEM (n ≥ 3). *p < 0.05 (vs. untreated PBMC). #p < 0.05 (vs. PBMC treated with Ox-LDL alone). &p < 0.05 (vs. PBMC treated with Ox-LDL and 0.2 mg/mL H-flavone). N.S.p > 0.05 (vs. untreated PBMC).
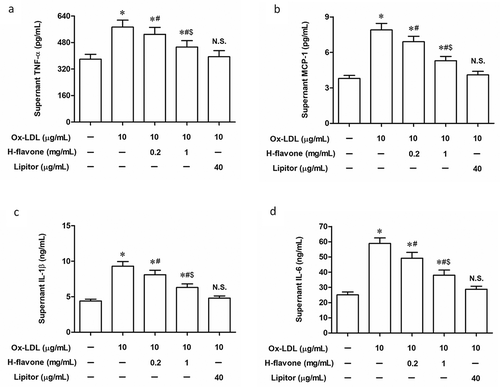
Ox-LDL-induced cell foaming and inflammation are inhibited by H-flavone via regulating CTPR6 expression
Studies have shown that CTRP6 regulates inflammation by targeting macrophages [Citation22]. Therefore, we hypothesized that H-flavone inhibited foam cell formation and reduced cellular inflammation by regulating intracellular CTPR6 expression. PBMC were treated with Ox-LDL, H-flavone, CTRP6 recombinant protein (rCTRP6) or/and CTRP6 neutralizing antibody (anti-CTRP6) for 24 hours. As expected, Ox-LDL-induced PBMC treated with H-flavone and rCTRP6 secreted the same elevated amount of CTRP6 ()). However, after H-flavone-treated PBMC were affected by anti-CTRP6, CTRP6 expression was reduced to a level comparable to the control ()). Additionally, the percentage of intracellular CE was decreased when Ox-LDL-induced PBMC were treated with H-flavone or rCTRP6 ()), anti-CTRP6 inhibited the reduction of CE in PBMC induced by H-flavone at the same time ()). In accordance, secretion of TNF-α, MCP-1, IL-1β, and IL-6 were markedly decreased in Ox-LDL-induced PBMC treated with H-flavone or rCTRP6, whereas anti-CTRP6 rescued the inflammatory cytokine decreasing caused by H-flavone ().
Figure 3. Ox-LDL-induced foaming and inflammation were inhibited by H-flavone via regulating CTPR6 expression.
Mouse PBMC were treated with Ox-LDL (10 µg/mL), H-flavone (1 mg/mL), CTRP6 recombinant protein (rCTRP6; 0.25 µg/mL) or/and CTRP6 neutralizing antibody (anti-CTRP6; 0.5 µg/mL) for 24 hours. (a) Content of CTRP6 in cell culture supernatant. (b) The percentage of cholesterol ester (CE) was represented the degree of PBMC foaming. Protein levels of TNF-α (c), MCP-1 (d), IL-1β (e), and IL-6 (f) were detected by ELISA. The columns were presented as the mean ± SEM (n ≥ 3). *p < 0.05 (vs. PBMC treated with Ox-LDL alone). N.S.p > 0.05 (PBMC treated with Ox-LDL and H-flavone vs. PBMC treated with Ox-LDL and rCTRP6 or PBMC treated with Ox-LDL, H-flavone and anti-CTRP6 vs. PBMC treated with Ox-LDL alone).
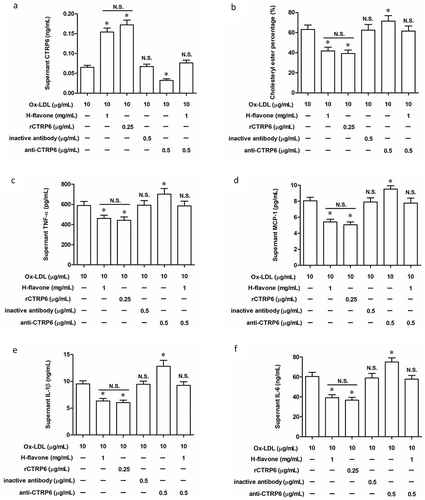
H-flavone reduces serum total cholesterol (TC), triglyceride (TG) and low-density lipoprotein (LDL) levels and inhibits the production of inflammatory cytokines in vivo
We then studied the effects of H-flavone on AS biochemical markers and inflammatory responses in AS model mice. After using H-flavone to feed the mice in the sixth week (early administration) and the twelfth week (late administration) of birth, serum content of CTPR6 was obviously increased compared to the model group ()). Before given H-flavone, serum levels of triglyceride (TG), total cholesterol (TC) and low-density lipoprotein (LDL) consistently were much higher in the normal chow diet-fed group (). Interestingly, serum TG, TC and LDL levels in the mice fed H-flavone were significantly reduced, and the early feeding effect of H-flavone was more pronounced (). Moreover, a substantial reduction in the secretion of inflammatory cytokines, including hs-CRP, TNF-α, IL-1β, and IL-6 levels, was observed in AS model mice after they were treated with H-flavone (). The above results demonstrated that both late and early administration with H-flavone reduced serum TG, TC and LDL levels and inhibited inflammation in AS model mice and these effects were more potent in early administration.
Figure 4. H-flavone reduces serum total cholesterol (TC), triglyceride (TG) and low-density lipoprotein (LDL) levels and inhibits the production of inflammatory cytokines in vivo.
To establish AS model mice, the apoE-/- mice were allowed free access to high fat diet (containing 21% fat and 0.15% cholesterol) and water. After 6 weeks of birth, the mice were placed on the study diets. The experimental group was fed H-flavone (75mg/kg/d) while the control group were fed normal saline. The early administration group began to administer H-flavone at 6 weeks of age for 6 weeks, and the late administration group was administered with H-flavone at 12 weeks of age for 6 weeks. (a) Serum levels of CTRP6. (b) Serum levels of TG. (c) Serum levels of TC. (d) Serum levels of LDL. Protein levels of hs-CRP (e), TNF-α (f), IL-1β (g), and IL-6 (h) were detected by ELISA. The columns were presented as the mean ± SEM (n = 10). *p < 0.05 (vs. untreated model). #p < 0.05 (vs. late administration).
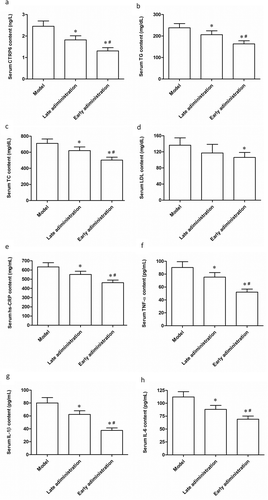
H-flavone suppresses macrophage infiltration and the development of AS plaque
The proportion of lesion area containing CD68+ macrophages was markedly decreased in late and early administration group compared with the AS model mice (), top panels). Specially, the lesion area in the early H-flavone treatment group almost disappeared completely (), top panels). Oil Red O staining showed that the Oil Red O positive cells in the H-flavone treatment group were significantly reduced compared with the model group (), top panels). Furthermore, macrophage infiltration index ()) and percentage of damage area ()) of the administration with H-flavone was lower than that of the AS model. Similarly, early treatment group showed the lowest macrophage infiltration index ()) and percentage of damage area ()).
Figure 5. H-flavone suppresses macrophage infiltration and the development of AS plaque.
To establish AS model mice, the apoE-/- mice were allowed free access to high fat diet (containing 21% fat and 0.15% cholesterol) and water. After 6 weeks of birth, the mice were placed on the study diets. The experimental group was fed H-flavone (75mg/kg/d) while the control group were fed normal saline. The early administration group began to administer H-flavone at 6 weeks of age for 6 weeks, and the late administration group was administered with H-flavone at 12 weeks of age for 6 weeks. (a) Representative micrographs of aortic roots stained with anti-macrophage CD68 antibody (top panels are magnified 100 times) and atherosclerotic plaque Oil Red O staining (under panels are magnified 40 times). (b) Macrophage infiltration index. (c) Percentage of damage area. The experiments were repeated three times. The columns were presented as the mean ± SEM (n = 10). *p < 0.05 (late administration vs. untreated model or early administration vs. late administration). #p < 0.05 (vs. late administration).
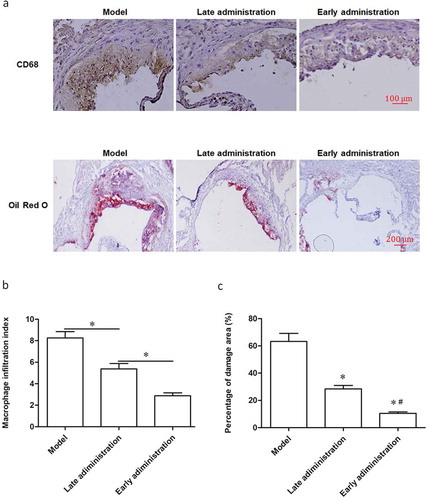
Discussion
H-flavone has become the subject of considerable attention, mainly because of its beneficial effects for cardiovascular diseases and cancers [Citation15,Citation16]. Here, we focused on the anti-inflammatory and anti-atherogenic mechanisms of H-flavone. The results of the present study indicated that H-flavone had efficacy against macrophage foaming, inflammatory, and vascular plaque formation in AS. H-flavone contains a variety of beneficial ingredients, mainly including quercetin, kaempferol and isorhamnetin [Citation23,Citation24]. It has been reported that quercetin was effective to regulate the atherosclerotic inflammatory process by inhibiting Ox-LDL induced endothelial leukocyte adhesion [Citation25]. On the other hand, kaempferol showed anti-atherosclerotic effect by modulating the expression of inflammatory molecules, such as E-selectin (E-sel), intercellular adhesion molecule-1 (ICAM-1), vascular cell adhesionmolecule-1 (VCAM-1) and MCP-1 [Citation26]. A emerging study revealed that isorhamnetin inhibited atherosclerotic plaque development by PI3K/AKT activation and HO-1induction [Citation27]. Our results were consistent with previous studies which showed multiple factors in H-flavone contributed to lowering inflammatory responses and development of AS. Using the AS model mice (apoE-/- mice fed with high-fat diet), we found that both short-term (6 weeks) and long-term (12 weeks) with H-flavone dramatically reduced serum TC, TG and LDL-C accumulation. Furthermore, immunochemistry and Oil Red O staining of H-flavone-treated aortas revealed that H-flavone lowered the macrophage infiltration index and percentage of damage area. Simultaneously, H-flavone reduced the expression of specific inflammatory factors implicated in atherogenesis. Herein, H-flavone has the potential to prevent onset of pathologies associated with AS.
In the present study, we further found that H-flavone markedly inhibited macrophage foaming, inflammatory responses and vascular plaque formation through increasing CTPR6 expression in vitro and in vivo models. H-flavone dramatically increased CTRP6 expression both in vivo and in vitro. Recent studies indicate that many members of the CTRP family can affect both metabolic and cardiovascular functions [Citation28–Citation30]. CTRP6 intimately associated with type Ⅰ diabetes, vitiligo, adipose production tumor neovascularization and hepatocellular carcinoma [Citation31–Citation34]. Researches indicated that CTRP6 was highly expressed in adipose tissue [Citation35]. Similarly, CTRP6 mediated the ectopic lipogenesis through AdipoR1/Erk/PPARγ signaling pathway in myoblasts. CTRP6 could attenuate cardiac fibrosis via inhibiting myofibroblast differentiation [Citation36]. Several studies demonstrated that markers at the CTRP6 locus were associated with type I diabetes [Citation31,Citation32]. Emerging literature suggested that CTRP6 induced the expression of IL-10 in monocytic cells [Citation11]. Our data firstly showed that high expression of CTRP6 attenuated Ox-LDL-induced mouse PBMC foaming. In addition, H-flavone-activated CTRP6 could reduce the expression of inflammatory factors, including TNF-α, MCP-1, hs-CRP, IL-1β, and IL-6, suggesting that CTRP6 inhibited the inflammatory response of macrophages.
In summary, this study demonstrates that H-flavone effectively inhibits macrophage foaming, inflammation and reduced the risk of vascular plaque formation by upregulating the expression of CTRP6. H-flavone may be used to treat and prevent AS based on its substantial effects of anti-inflammatory and hypolipidemia.
Disclosure statement
No potential conflict of interest was reported by the authors.
Additional information
Funding
References
- Hansson GK, Libby P. The immune response in atherosclerosis: a double-edged sword. Nat Rev Immunol. 2006;6(7):508–519.
- Weber C, Zernecke A, Libby P. The multifaceted contributions of leukocyte subsets to atherosclerosis: lessons from mouse models. Nat Rev Immunol. 2008;8(10):802–815.
- Mcgettrick AF, O’Neill LA. How metabolism generates signals during innate immunity and inflammation. J Biol Chem. 2013;288(32):22893–22898.
- Bensinger SJ, Tontonoz P. Integration of metabolism and inflammation by lipid-activated nuclear receptors. Nature. 2008;454(7203):470–477.
- Schäffler A, Buechler C. CTRP family: linking immunity to metabolism. Trends Endocrinol Metab. 2012;23(4):194–204.
- Yi W, Sun Y, Yuan Y, et al. C1q/tumor necrosis factor-related protein-3, a newly identified adipokine, is a novel antiapoptotic, proangiogenic, and cardioprotective molecule in the ischemic mouse heart. Circulation. 2012;125(25):3159–3169.
- Wei Z, Seldin MM, Natarajan N, et al. C1q/tumor necrosis factor-related protein 11 (CTRP11), a novel adipose stroma-derived regulator of adipogenesis. J Biol Chem. 2013;288(15):10214–10229.
- Wu WJ, Mo DL, Zhao CZ, et al. Knockdown of CTRP6 inhibits adipogenesis via lipogenic marker genes and Erk1/2 signalling pathway. Cell Biol Int. 2015;39(5):554–562.
- Lee W, Kim MJ, Park EJ, et al. C1qTNF-related protein-6 mediates fatty acid oxidation via the activation of the AMP-activated protein kinase. FEBS Lett. 2010;584(11):2491.
- Fan RH, Zhu XM, Sun YW, et al. CTRP6 inhibits fibrogenesis in TGF-Î21-stimulated human dermal fibroblasts. Biochem Biophys Res Commun. 2016;475(4):356–360.
- Kim MJ, Lee W, Park EJ, et al. C1qTNF-related protein-6 increases the expression of interleukin-10 in macrophages. Mol Cells. 2010;30(1):59–64.
- Yu X-H, Zhang D-W, Zheng X-L, et al. C1q tumor necrosis factor-related protein 9 in atherosclerosis: mechanistic insights and therapeutic potential. Atherosclerosis. 2018;276:109–116.
- Zhang P, Huang C, Li J, et al. Globular CTRP9 inhibits oxLDL-induced inflammatory response in RAW 264.7 macrophages via AMPK activation. Mol Cell Biochem. 2016;417(1–2):67–74.
- Guliyev VB, Gul M, Yildirim A. Hippophae rhamnoides L.: chromatographic methods to determine chemical composition, use in traditional medicine and pharmacological effects. J Chromatogr B Analyt Technol Biomed Life Sci. 2004;812(1):291–307.
- Carl G, Cecilia WN, Patrick A, et al. Antiproliferative effects of sea buckthorn (Hippophae rhamnoides L.) extracts on human colon and liver cancer cell lines. Food Chem. 2010;120(4):1004–1010.
- Basu M, Prasad R, Jayamurthy P, et al. Anti-atherogenic effects of seabuckthorn (Hippophaea rhamnoides) seed oil. Phytomedicine. 2007;14(11):770–777.
- Geetha S, Sai RM, Mongia SS, et al. Evaluation of antioxidant activity of leaf extract of Seabuckthorn (Hippophae rhamnoides L.) on chromium(VI) induced oxidative stress in albino rats. J Ethnopharmacol. 2003;87(2):247–251.
- Michel T, Destandau E, Elfakir C. Evaluation of a simple and promising method for extraction of antioxidants from sea buckthorn (Hippophaë rhamnoides L.) berries: pressurised solvent-free microwave assisted extraction. Food Chem. 2011;126(3):1380–1386.
- Zeb A. Anticarcinogenic potential of lipids from Hippophae–evidence from the recent literature. Asian Pac J Cancer Prev Apjcp. 2006;7(1):32.
- Chen L, He T, Han Y, et al. Pentamethylquercetin improves adiponectin expression in differentiated 3T3-L1 cells via a mechanism that implicates PPARγ together with TNF-α and IL-6. Molecules. 2011;16(7):5754.
- Geetha S, Sai RM, Singh V, et al. Anti-oxidant and immunomodulatory properties of seabuckthorn (Hippophae rhamnoides)–an in vitro study. J Ethnopharmacol. 2002;79(3):373–378.
- Lei X, Seldin MM, Little HC, et al. C1q/TNF-related protein 6 (CTRP6) links obesity to adipose tissue inflammation and insulin resistance. J Biol Chem. 2017;292(36):14836.
- Yang F, Quan J, Zhang TY, et al. Multidimensional counter-current chromatographic system and its application. J Chromatogr A. 1998;803(1–2):298.
- Li X, Kong L, Liu H. Advances on effective compositions of Seabuckthorn (Hippophae rhamnoides L.). J Jilin Agric Univ. 2007;29(2):162–167.
- Bhaskar S, Sudhakaran PR, Helen A. Quercetin attenuates atherosclerotic inflammation and adhesion molecule expression by modulating TLR-NF-κB signaling pathway. Cell Immunol. 2016;310:131–140.
- Kong L, Luo C, Li X, et al. The anti-inflammatory effect of kaempferol on early atherosclerosis in high cholesterol fed rabbits. Lipids Health Dis. 2013;12(1):115.
- Luo Y, Sun G, Dong X, et al. Isorhamnetin attenuates atherosclerosis by inhibiting macrophage apoptosis via PI3K/AKT activation and HO-1 induction. Plos One. 2015;10(3):e0120259.
- Ouchi N, Walsh K. Cardiovascular and metabolic regulation by the adiponectin/C1q/tumor necrosis factor-related protein family of proteins. Circulation. 2012;125(25):3066–3068.
- Hou M, Liu J, Liu F, et al. C1q tumor necrosis factor-related protein-3 protects mesenchymal stem cells against hypoxia- and serum deprivation-induced apoptosis through the phosphoinositide 3-kinase/Akt pathway. Int J Mol Med. 2014;33(1):97–104.
- Chavali VR, Sommer JR, Petters RM, et al. Identification of a promoter for the human C1Q-tumor necrosis factor-related protein-5 gene associated with late-onset retinal degeneration. Invest Ophthalmol Vis Sci. 2010;51(11):5499–5507.
- Eyre S, Hinks A, Bowes J, et al. Overlapping genetic susceptibility variants between three autoimmune disorders: rheumatoid arthritis, type 1 diabetes and coeliac disease. Arthritis Res Ther. 2010;12(5):R175–R175.
- Wallis RH, Wang K, Marandi L, et al. Type 1 diabetes in the BB rat: a polygenic disease. Diabetes. 2009;58(4):1007.
- Jin Y, Birlea SA, Fain PR, et al. Variant of TYR and autoimmunity susceptibility loci in generalized vitiligo. N Engl J Med. 2010;362(18):1686.
- Takeuchi T, Adachi Y, Nagayama T. Expression of a secretory protein C1qTNF6, a C1qTNF family member, in hepatocellular carcinoma. Anal Cell Pathol. 2016;34(3):113–121.
- Wong GW, Krawczyk SA, Kitidis-Mitrokostas C, et al. Molecular, biochemical and functional characterizations of C1q/TNF family members: adipose-tissue-selective expression patterns, regulation by PPAR-gamma agonist, cysteine-mediated oligomerizations, combinatorial associations and metabolic functions. Biochem J. 2008;416(2):161–177.
- Lei H, Wu D, Wang JY, et al. C1q/tumor necrosis factor-related protein-6 attenuates post-infarct cardiac fibrosis by targeting RhoA/MRTF-A pathway and inhibiting myofibroblast differentiation. Basic Res Cardiol. 2015;110(4):1–17.