ABSTRACT
Collagen-derived dipeptide prolyl hydroxyproline (Pro-Hyp) is involved in the proliferation and differentiation of various types of cultured cells. To elucidate the mechanism underlying Pro-Hyp actions during osteoblast differentiation, we hypothesized that proteins binding to Pro-Hyp serve to mediate cellular signaling, affecting Runx2 expression. Recently, we performed the characterization of Foxg1, that it enhances Runx2 expression in the presence of Pro-Hyp. Our findings indicate that Pro-Hyp directly binds to the Foxg1 recombinant protein, which leads to the structural alteration of the Foxg1 protein. In addition, Foxg1 appears to interact with Runx2 in the absence of Pro-Hyp, with Pro-Hyp disrupting the interaction between Foxg1 and Runx2. Collectively, our results indicate that the Pro-Hyp bound Foxg1 alters the structured conformation of Foxg1, resulting in conformational changes that lead to dissociation from Runx2. These novel findings suggest that during osteoblast differentiation, Pro-Hyp mediates Runx2 activity though directly binding to Foxg1 and increases Runx2 expression.
Abbreviations: CPT: collagen peptide; GST: Glutathione S-transferase; PAGE: Polyacrylamide gel electrophoresis; PCR: Polymerase chain reaction; prolyl hydroxyproline: Pro-Hyp
Graphical abstract
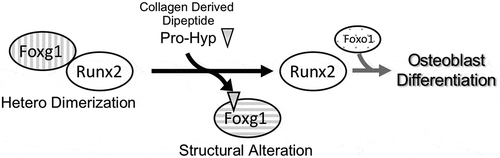
Pro-Hyp bound Foxg1 appears to dissociate Runx2 from the Foxg1 complex.
Collagen contains approximately 90% of the organic compound in the osseous tissue and is one of the important components of osseous tissue [Citation1]. Osseous tissue is maintained by remodeling with osteoblasts and osteoclasts, while collagen is synthesized using osteoblasts as the main component of bone matrix protein [Citation2,Citation3]. In osteoclasts, old collagen is degraded into collagen peptide (CPT) by enzymatic cleavage of the bone matrix [Citation4,Citation5]. One of the major components of CPT is Pro-Hyp, which is characterized as mostly abundant dipeptides in collagen. Moreover, Pro-Hyp which known to possess physiological functions, appear in blood at high concentrations after oral ingestion of collagen hydrolysate [Citation6,Citation7]. In addition, it has been reported that Hyp-Gly and Glu-Hyp-Gly detected in the blood after oral ingestion of fish collagen hydrolysate [Citation8]. Collagen peptide promotes proliferation and differentiation on various types of cultured cell, including osteoblasts [Citation9], chondrocytes [Citation10,Citation11], and fibroblasts [Citation12]. We previously demonstrated that Pro-Hyp promotes the differentiation of osteoblasts by increasing Runx2 mRNA expression. In addition, Runx2/Cbfa1 is an important transcription factor necessary for osteoblast differentiation and bone formation [Citation13]. Indeed, Runx2 binds to the promoter region of genes such as Col1a1, osteocalcin, and osterix [Citation14].
Forkhead box g1 (Foxg1; formerly known as BF-1, Brain Factor 1) is a transcriptional regulatory factor belonging to the forkhead protein family and has a forkhead type DNA binding region at the center [Citation15]. In the molecular structure of Foxg1, proline- and histidine-rich regions and poly glutamine repeat sequences are present on the N-terminal side and the serine-rich region on the C-terminal side [Citation16]. Foxg1 is also important for the formation of the cerebral cortex and inner ear [Citation17–Citation19]. Previously, we have focused on osteoblast differentiation, and although Foxg1 inhibits osteoblast differentiation [Citation20], we have found that Foxg1 is required for alkaline phosphatase (ALP) activity and the gene expressions of master genes, such as osteocalcin, Col1α1, and osterix, during osteoblast differentiation [Citation21].
Foxg1 is structurally and functionally highly conserved to Foxo1, which acts as a Runx2 hetero-dimer partner. Interestingly, it has recently been reported that the binding between Foxo1 and synthetic compounds having a N-heterocycle are important in regulating gluconeogenesis related gene expression in primary hepatocytes [Citation22]. Foxo1 directly interacts with Runx2 and up-regulates these target genes. Thus, we hypothesized that Foxg1 directly binds to Pro-Hyp and leads to conformational changes of the Foxg1 complex formation. Finally, we examined the interaction between Foxg1 and Runx2 involving Pro-Hyp binding.
In this study, we have performed recombinant binding assays with Pro-Hyp and Foxg1, and revealed the Pro-Hyp binding region on Foxg1. In addition, using GST pull-down assays, Pro-Hyp bound Foxg1 appears to dissociate Runx2 from the Foxg1 complex. Collectively, our novel findings indicate that Pro-Hyp promotes Runx2 mediated osteoblast differentiation through directly binding to Foxg1.
Materials and methods
cDNA plasmids
Foxg1 and Runx2 mouse cDNA were amplified using polymerase chain reaction (PCR) from single strand cDNA obtained from mouse brain and the mouse osteoblastic cell line MC3T3-E1. Full-length mFoxg1 cDNA were subcloned into the bacterial expression vector pGEX4T3 as the GST-Foxg1 fusion protein as previously described [Citation20]. The GST-Foxg1 expression plasmids expressing the truncated forms of Foxg1 (Foxg1A: amino acids 1–171, Foxg1B: amino acids 172–263, Foxg1C: amino acids 264–375, Foxg1D: amino acids 376–481) were subcloned into pGEX4T3 through PCR using mFoxg1-pGEX4T3 as a template. The primer sequences are listed in . The Foxg1A region contains a poly-Q sequence, while the Foxg1B region contains a DNA binding domain. The Foxg1D region contains the lysine-specific demethylase 5B-binding region. To generate the protein expression vector without the GST tag (pFex), we reconstructed pFex by polymerase chain reaction using the pGEX4T3 vector as a template. The Foxg1 and Runx2 cDNA fragments were inserted into the EcoR1 sites of the pFex vector, respectively. All sequences were verified using automatic DNA sequencing.
Table 1. Primer sequences for subcloning.
Recombinant proteins
The prepared plasmids were transformed into the BL21 strain and the transformed bacteria were cultured at 37°C until the OD 600 had reached 0.6. IPTG (0.4 mM) was added and the culturing continued for 3 h in LB-medium at 28°C (shaking at 180 rpm). Bacterial lysates containing the proteins were prepared by sonication in PBSP buffer containing 137 mM NaCl, 10 mM Na2HPO4, 2.7 mM KCl, 1.8 mM KH2PO4, 10% Glycerol, 1 mM DTT, protease inhibitor tablets, and 1 mM PMSF, and centrifuged at 7500 rpm for 5 m for collection of the GST fusion protein. The expression of recombinant proteins was confirmed using Western blot analysis.
Western blot analysis
Recombinant proteins were separated on 4–20% (wt/vol) gradient polyacrylamide gels by SDS-polyacrylamide gel electrophoresis (PAGE) and transferred to PVDF membranes by using trans blot turbo (BIO-RAD). Membranes were incubated for 1 h in TBS (50 mM Tris-HCl, 138 mM NaCl, 2.7 mM KCl) -Tween 20 containing 5%(wt/vol) nonfat dry milk, and then exposed to a 1:1,000 dilution of rabbit polyclonal antiserum anti-Foxg1 (Abcam, catalog no. ab18259) or anti-Runx2 (Cell signaling, catalog no.84865) at room temperature for 1 h. The membranes were washed and incubated with 1:2,000 anti-rabbit IgG (H + L) conjugated to HRP for 45 m at room temperature, and the blots were developed using the ECL plus Western blot analysis detection system. In addition, the Anti-GST antibody conjugated with HRP (GE Health care, catalog no. RPN1236) was used for the detection of the GST fusion protein (1:5000).
Magnetic beads pull-down assay
Pro-Hyp-Gly five times (Pro-Hyp-Gly)5 NH2- linked magnetic beads or Glu-Hyp-Gly five times (Glu-Hyp-Gly)5 NH2- linked magnetic beads or control magnetic beads (6µl) (TAMAGAWA seiki Co.,Ltd) were equilibrated with binding buffer (150 mM KCl, 10 mM HEPES (pH = 7.8), 1 mM EDTA, 10% Glycerol, 1 mM DTT, Protease inhibitor tablets) and incubated with 2 mg of Foxg1 full-length protein or Foxg1 truncated mutants recombinant proteins at 4°C for overnight with occasional agitation. After being washed three times with binding buffer, bound proteins were eluted with SDS-PAGE sample buffer and were subjected to SDS-PAGE and Western blot analysis. To define specific binding to Pro-Hyp, excess amounts of free Pro-Hyp or Pro-Pro (10 mM, 100 mM) or Hyp-Gly (5 mM, 50 mM) were used for the analysis. Hyp-Gly could not dissolve at a concentration of 1M, therefore the competition assay was performed 5 mM and 50 mM that half the amount of other peptides.
Glutathione S-transferase (GST) pull-down assays
The GST-Foxg1 recombinant protein was purified with Glutathione Sepharose 4B Beads (GE Health care). Purified GST-Foxg1 was incubated with tag-free Runx2 recombinant protein on ice for 3 h. Foxg1-Runx2 complexes were precipitated with Glutathione Sepharose 4B Beads. The beads were then washed using PBSP buffer as previously described. The presence of Runx2 in the precipitates was detected using Western blot analysis with the rabbit anti-Runx2 antibody (Cell signaling, catalog no.84865)
Protease digestion assays
The plasmid Foxg1-pGEX4T3 or pFex-Runx2 was used to synthesize the GST-Foxg1 or pFex-Runx2 recombinant protein in E. coli. The purified GST-Foxg1 or pFex-Runx2 recombinant protein was subsequently aliquoted into 20 µL volumes; 30 µL of PBS with or without Pro-Hyp were then added. These mixtures were incubated for 10 m at room temperature, separated into 25 µL aliquots, and 5 µL of solubilized trypsin (wako 202-15951) was added. Protease digestion was allowed to proceed for 1 m at 37°C. The reaction was then terminated by the addition of 10 µL of sample buffer and boiling for 5 m. SDS-PAGE and Western blot analyses were then performed using the rabbit anti-Foxg1 antibody (abcam ab18259) or rabbit anti-Runx2 antibody (cell signaling 8486S).
Results and discussion
To demonstrate whether Pro-Hyp directly binds to Foxg1, we prepared the recombinant Foxg1 protein and (Pro-Hyp-Gly) 5 linked magnetic beads or (Glu-Hyp-Gly)5 linked magnetic beads or control magnetic beads for the binding assay. The magnetic beads were linked to (Pro-Hyp-Gly)5 or (Glu-Hyp-Gly)5 of the N terminus. We considered that Pro-Hyp linked magnetic beads could not detect Foxg1 bound to Pro-Hyp, as molecular size of Pro-Hyp is very small. For this reason, to make it easy to detection sensitivity of Pro-Hyp bound Foxg1, we used (Pro-Hyp-Gly)5 linked magnetic beads as positive control beads. In addition, considering that repeated binding of Pro-Hyp causes steric hindrance, Gly which is low molecule weight and neutral amino acid was inserted between Pro-Hyp and Pro-Hyp. We used (Glu-Hyp-Gly)5 magnetic beads as negative control beads. Because Glu-Hyp-Gly which is similar to Pro-Hyp-Gly was reported as a biologically active substance [Citation23]. To confirm binding specificity to Pro-Hyp, free Pro-Hyp or Pro-Pro was added at a concentration of 10 mM or 100 mM to perform the competition assay. And free Hyp-Gly was added at a concentration of 5 mM or 50 mM to perform the competition assay. The binding between Foxg1 and (Pro-Hyp-Gly)5 magnetic beads dissociation was dependent on Pro-Hyp concentration (), lanes 2, 3 and 4). In addition, the binding between Foxg1 and (Pro-Hyp-Gly)5 magnetic beads dissociation was occurred at only high concentration of Pro-Pro (), lanes 6, 7 and 8). Whereas, the binding between Foxg1 and (Pro-Hyp-Gly)5 magnetic beads dissociation wasn’t dependent on Hyp-Gly concentration (), lanes 10, 11and 12) On the other hand, no binding between Foxg1 and the (Glu-Hyp-Gly)5 magnetic beads was observed in the absence or presence of Pro-Hyp (), lane 2, 3 and 4). Foxg1 didn’t bind to control magnetic beads (data not shown). These data indicate that Pro-Hyp directly binds to Foxg1 and Pro-Pro partially binds to Foxg1.
Figure 1. Foxg1 binding to (Pro-Hyp-Gly)5 magnetic beads in a Pro-Hyp specific manner. (a) Competition assay. Foxg1 extracts were mixed with (Pro-Hyp-Gly)5 magnetic beads. At that time, an excess amount of Pro-Hyp or Pro-Pro was added at a concentration of 10 mM (lanes 3 and 7), and 100 mM (lanes 4 and 8), Hyp-Gly was added at a concentration of 5 mM (lane 11), 50 mM (lane 12) and a competition inhibition assay was performed followed by Western blot analysis for Foxg1. Pro-Hyp, Pro-Pro or Hyp-Gly was not added to lanes 2 or 6 or 10. The equivalent of 0.1 µg of Foxg1 protein extracts were used as input (lanes 1, 5 and 9). Detailed methods are described in this report. (b) Competition assay. Foxg1 extracts were mixed with (Glu-Hyp-Gly)5 magnetic beads. At that time, an excess amount of Pro-Hyp was added at a concentration of 10 mM (lane 3), 100 mM (lane 4), and a competition inhibition assay was performed followed by Western blot analysis for Foxg1. Pro-Hyp was not added to lane 2. The equivalent of 0.1 µg of Foxg1 protein extracts were used as input (lane 1). Detailed methods are described in this report.
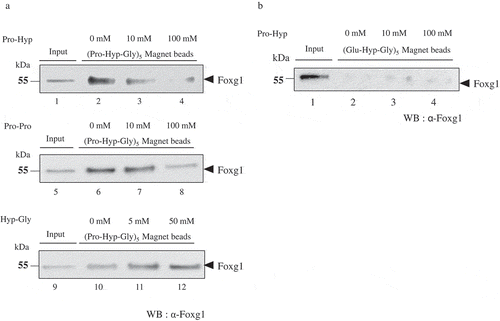
To characterize the Pro-Hyp binding domain in the Foxg1 protein, we next prepared the truncated mutants. Due to the recognition site of available Foxg1 antibodies for the GST-fused Foxg1, truncated proteins were adapted as shown in ). Each truncated mutant of the Foxg1 recombinant protein was combined and incubated with (Pro-Hyp-Gly)5 magnetic beads or control magnetic beads. The anti-GST antibody was used for Western blot analysis to detect each Foxg1 truncated mutant. As a result, both GST-Foxg1A and GST-Foxg1B appear to bind to (Pro-Hyp-Gly)5 magnetic beads () lanes 3 and 6). However, no observation of the binding against GST-Foxg1C or GST-Foxg1D was detected () lanes 8, 9, 11, 12, 14, and 15). These results clearly demonstrate that Pro-Hyp binds to both the Foxg1A and B region (amino acids 1–172, 173–263)
Figure 2. A and B region of Foxg1 binding to (Pro-Hyp-Gly)5 magnetic beads. (a) Schematic showing the region structure of Foxg1 and the truncated mutants. The amino acid numbers of each mutant are labeled. Foxg1 was deleted as follows (Foxg1-A: amino acid 1–171, Foxg1-B: amino acid 172–263, Foxg1-C: amino acid 264–374, and Foxg1-D: amino acid 375–481) and GST tag was added, and E. coli produced a recombinant protein. We produced various GST-Foxg1 truncated mutants (GST-Foxg1-A 43 kDa (lane 2) GST-Foxg1-B 36 kDa (lane 3) GST-Foxg1-C 37 kDa (lane 4) GST-Foxg1-D 36 kDa (lane 5) and GST 25 kDa (lane 1) followed by Western blot for GST (Figure 2(a)). (b) Binding assay. The Foxg1 truncated mutants or only GST tag was mixed with control magnet beads (lanes 2, 5, 8, 11, 14) or (Pro-Hyp-Gly)5 magnetic beads (lanes 3, 6, 9, 12, 15). They were then analyzed using Western blot analysis using the anti-GST antibody. Foxg1 and GST protein extracts were used as the input (lanes 1, 4, 7, 10, 13). Detailed methods are described in this report.
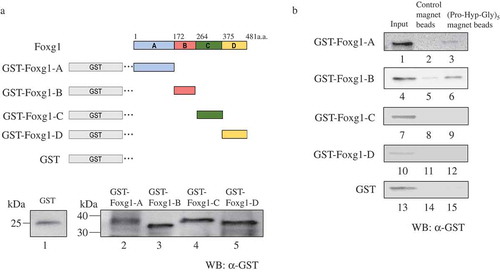
To clarify the Foxg1 conformation changes with or without Pro-Hyp, we examined the differential sensitivity of trypsin as a protease digestion assay. The GST-Foxg1 protein or GST-Foxg1 with Pro-Hyp was then subject to trypsin digestion into the GST-Foxg1 protein with or without Pro-Hyp, and the digested products were detected using Western blot analysis. As shown in , in the absence of Pro-Hyp, GST-Foxg1 indicated a high sensitivity to trypsin (, lane 2). In the presence of Pro-Hyp, however, trypsin resistant 50 kDa and 30 kDa Foxg1 fragments were observed (, lane 4). Indeed, these fragments indicated that the C-terminal region was in Foxg1, since it was used for the antibody to detect Foxg1 amino acid 400 to the C-terminals. Runx2 indicated a high sensitivity to trypsin in the absence or presence of Pro-Hyp (data not shown). It is suggested that Pro-Hyp hasn’t trypsin inhibitory activity. Therefore, these data suggest that Pro-Hyp binding alters the Foxg1 structural conformation or Pro-Hyp binds Foxg1 at basic amino acid which trypsin cleavages. Taken together with the previous result shown in ), we considered that Pro-Hyp binds to the DNA binding region of Foxg1, with the resulting conformational change of Foxg1 or steric effects of Pro-Hyp on Foxg1 having the possibility to change the DNA affinity of Foxg1.
Figure 3. Pro-Hyp induces a Foxg1 conformational change. Purified GST-Foxg1 fusion protein was incubated at 37°C for two consecutive 10 m and 1 m periods of time. For the first period, Pro-Hyp was added to 0.1 mM, respectively, to lanes 3 and 4. An equal volume of water was added to lanes 1 and 2. For the second period, trypsin was added to 0.1 µg. Foxg1 was digested with trypsin and analyzed by Western blot analysis (lanes 2 and 4). Detailed methods are described in this report.
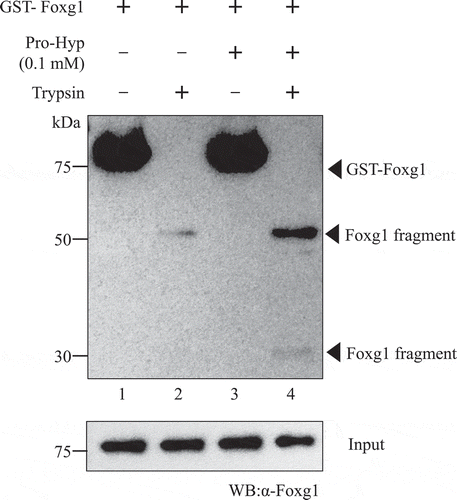
Based on previously reported results, Foxo1 directly interacts with Runx2 during osteoblast differentiation [Citation24]. Finally, we examined the interaction between Foxg1 and Runx2 with Pro-Hyp binding. As shown by GST pull-down assay in ), the recombinant Runx2 full-length protein and the GST-Foxg1 protein were prepared from E.coli. GST-Foxg1 strictly interacted with Runx2 in the absence of Pro-Hyp (), lane 4). Surprisingly, the interaction of GST-Foxg1 with Runx2 was dissociated in the presence of Pro-Hyp (), lane 5). These results suggest that Pro-Hyp prevents the interaction between Foxg1 and Runx2. In HaCaT cells, Foxg1 interacts with Foxo1, negatively regulating the transcriptional activity of p21Cip1 [Citation25]. The Interaction of Runx2 with Foxo1 induces the expression of osteocalcin, which is the target gene of Runx2 [Citation14]. We previously clarified that Pro-Hyp induces Runx2 to mediate osteoblast differentiation [Citation26]. Altogether, we determined that Pro-Hyp mediated the prevention of the interaction between Foxg1 and Runx2 and may contribute to the strength of the interaction between Runx2 and Foxo1. These bindings may lead to osteoblast differentiation.
Figure 4. Pro-Hyp inhibits the interaction of Foxg1 with Runx2. (a) Runx2 recombinant protein was produced by E. coli (lane 1). GST-Foxg1 recombinant protein was produced by E. coli (lane 2). (b) Runx2 was incubated with glutathione sepharose beads containing bacterially expressed GST or GST-Foxg1, these proteins, and then analyzed using Western blot analysis using the Runx2 antibody. A mixture of Runx2 and GST (lanes 2 and 3) or GST-Foxg1 (lanes 4 and 5) proteins was precipitated by glutathione 4B beads. At that time, Pro-Hyp was added at a concentration of 0.1 mM (lanes 3 and 5). Lanes 2 and 4 were not administered Pro-Hyp. The Runx2 protein extract was used as the input (lane 1). Detailed methods are described in this report.
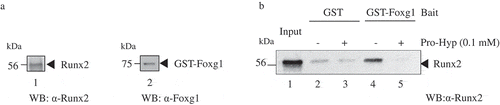
Focusing on the metabolites of CPT, few reports exist indicating that collagen-derived dipeptides are transported subcellularly by a peptide transporter [Citation27,Citation28]. In addition, cyclic glycine-proline binds to IGF1 and leads to the regulation of the interaction between IGF1 and the IGF binding protein [Citation29]. These results consider that Pro-Hyp is imported subcellularly through the peptide transporter and exists as the Pro-Hyp binding protein in the subcellular region.
Runx2 binds to the 360–456 amino acid residue in the Foxo1 protein [Citation14]. This region has high homology with Foxg1 on the C-terminal side. Collectively, these reports expect that the C-terminal region of Foxg1 is critical for the interaction with Runx2. This study clarified that Pro-Hyp binding to Foxg1 leads to the structural alteration of the C-terminal region in Foxg1. On the other hand, the N-terminal of Foxg1 could not be observed (data not shown). Taken together, the structural alteration of the C-terminal of Foxg1, induced by Pro-Hyp binding to the DNA binding region of Foxg1, may trigger the dissociation of the interaction between Foxg1 and Runx2. Although, it is unclear whether physiological cellular concentration of Pro-Hyp in osteoblastic cells. Although, Pro-Hyp previously reported to be detected in the blood after the oral ingestion of collagen hydrolysate (Cmax = 60.65 ± 5.74 µM) [Citation6]. Moreover, oral ingested [14C] Pro-Hyp is distributed in bone, cartilage and skin [Citation30]. We have previously reported that 0.1 − 1 mM of Pro-Hyp induces differentiation of MC3T3-E1 which is osteoblastic cells [Citation26]. Accordingly, Pro-Hyp was used at a concentration of 0.1mM in this paper. However, we can not discuss these Pro-Hyp effects are able to occur in physiological or pharmacological conditions. In conclusion, we considered that Pro-Hyp prevents the interaction between Foxg1 and Runx2 and may be part of the osteoblast differentiation mechanism.
Author contribution
Conceptualization: YK, YO, AM and HM
Investigation: KN
supervision: JS and HM
Writing the original draft: KN
All authors reviewed and approved the final manuscript.
Acknowledgments
The authors wish to acknowledge Airplants Bio co Ltd for technical advice on experiments.
Disclosure statement
No potential conflict of interest was reported by the authors.
References
- Phillip HW, Leroy K. The quantitative relationship of urinary peptide hydroxyproline excretion to collagen degradation. J Clin Invest. 1969;48:277–284.
- Liza JR, Nicola CP. Cellular and molecular mechanisms of bone remodeling. J Biol Chem. 2010;285:25103–25108.
- Karsenty G. Transcriptional control of skeletogenesis. Annu Rev Genomics Hum Genet. 2008;9:183–196.
- Adrian E, Ellen HF, Stephen EG, et al. Toward a molecular understanding of skeletal development. Cell. 1995;80:371–378.
- Nathalie O, Danielle JB, Zena W. Matrix remodeling during endochondral ossification. Trends Cell Biol. 2004;14:86–93.
- Ichikawa S, Morifuji M, Sato K, et al. Hydroxyproline-containing dipeptides and tripeptides quantified at high concentration in human blood after oral administration of gelatin hydrolysate. Int J Food Sci Nutr. 2010;61:1–9.
- Yazaki M, Ito Y, Yamada M, et al. Oral ingestion of collagen hydrolysate leads to the transportation of highly concentrated Gly-Pro-Hyp and its hydrolyzed form of Pro- Hyp into the bloodstream and skin. J Agric Food Chem. 2017;65:2315−2322.
- Taga Y, Kusubata M, Ogawa-Goto K, et al. Highly accurate quantification of hydroxyproline-containing peptides in blood using a protease digest of stable isotope-labeled collagen. J Agric Food Chem. 2014;62:12096–12102.
- JunLi L, Bing Z, Shu JS, et al. Bovine collagen peptides compounds promote the proliferation and differentiation of MC3T3-E1 pre- osteoblasts. PLoS One. 2014;9:e99920.
- Ohara H, Iida H, Takeuchi Y, et al. Effects of Pro-Hyp, a collagen hydrolysate-derived peptide, on hyaluronic acid synthesis using in vitro cultured synovium cells and oral ingestion of collagen hydrolysates in a guinea pig model of osteoarthritis. Biosci Biotechnol Biochem. 2010;17:2096–2099.
- Nakatani S, Mano H, Sampei C, et al. Chondroprotective effect of the bioactive peptide prolyl-hydroxyproline in mouse articular cartilage in vitro and in vivo. Osteoarthritis Cartilage. 2009;17:1620–1627.
- Ohara H, Ichikawa S, Matsumoto H, et al. Collagen-derived dipeptide, proline-hydroxyproline, stimulates cell proliferation and hyaluronic acid synthesis in cultured human dermal fibroblasts. J Dermatol. 2010;37:330–338.
- Komori T, Yagi H, Nomura S, et al. Targeted disruption of Cbfa1 results in a complete lack of bone formation owing to maturational arrest of osteoblasts. Cell. 1997;89:755–764.
- Shengyong Y, Haiyan X, Shibing Y, et al. Foxo1 mediates insulin-like growt factor 1 (IGF1)/insulin regulation of osteocalcin expression by antagonizing Runx2 in osteoblasts. J Biol Chem. 2011;286:19149–19158.
- Klaus HK, Walter K, Daniel EM. Unified nomenclature for the winged helix/forkhead transcription factors. Gene Dev. 2000;14:142–146.
- Nicholas B, Cathal S, Nicola I. Comparative evolutionary analysis of the FoxG1 transcription factor from diverse vertebrates identifies conserved recognition sites for microRNA regulation. Dev Genes Evol. 2007;217:227–233.
- Shouhong X, Carlos AS, Gabriela B, et al. Winged helix transcription factor BF-1 is essential for the development of the cerebral hemispheres. Neuron. 2000;14:1141–1152.
- Kumamoto T, Toma K, Gunadi, et al. Foxg1 coordinates the switch from nonradially to radially migrating glutamatergic subtypes in the neocortex through spatiotemporal repression. Cell Rep. 2013;3:931–945.
- Chan HH, Antonio S, Eseng L, et al. Foxg1 is required for proper separation and formation of sensory cristae during inner ear development. Dev Dyn. 2009;238:2725–2734.
- Adekunle M, Yummy N, Preethi G, et al. FOXG1 is overexpressed in hepatoblastoma. Hum Pathol. 2007;38:400–409.
- Kimira Y, Oodaira H, Nomura K, et al. Collagen-derived dipeptide prolyl- hydroxyproline promotes osteogenic differentiation through Foxg1. Cell Mol Biol Lett. 2017;27:22–27.
- Fanny L, Rebecca AH, Daniel L, et al. Selective inhibition of FOXO1 activator/repressor balance modulates hepatic glucose handling. Cell. 2017;171:824–835.
- Iwai K, Zhang Y, Saiga-Egusa A, et al. Blood concentration of food-derived peptides following oral intake of chicken collagen hydrolysate and its angiotensin-converting enzyme inhibitory activity in healthy volunteers. Food Sci Technol Res. 2009;56:326–330.
- Cristina CT, Yuexun L, Lwin MT, et al. Foxo1, a novel regulator of osteoblast differentiation and skeletogenesis. J Biol Chem. 2010;285:31055–31065.
- Joan S, Hong-Van L, Lijian S, et al. Integration of smad and forkhead pathways in the control of neuroepithelial and glioblastoma cell proliferation. Cell. 2004;117:211–223.
- Kimira Y, Ogura K, Taniuchi Y, et al. Collagen-derived dipeptide prolyl-hydroxyproline promotes differentiation of MC3T3-E1 osteoblastic cells. Biochem Biophys Res Commun. 2016;453:498–501.
- Kitakaze T, Sakamoto T, Kitano T, et al. The collagen derived dipeptide hydroxyprolyl-glycine promotes C2C12 myoblast differentiation and myotube hypertrophy. Biochem Biophys Res Commun. 2007;478:1292–1297.
- Misako AI, Dale L, Ming ZF, et al. Transport of a tripeptide, Gly-Pro-Hyp, across the porcine intestinal brush-border membrane. J Pept Sci. 2007;13:468–474.
- Jian G, Peter G, Panzao Y, et al. Cyclic glycine-proline regulates IGF-1 homeostasis by altering the binding of IGFBP-3 to IGF-1. Sci Rep. 2014;4:4388.
- Kawaguchi T, Nanbu NP, Kurokawa M. Distribution of prolylhydroxyproline and its metabolites after oral administration in rats. Biol Pharm Bull. 2012;35:422–427.