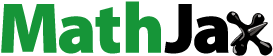
ABSTRACT
The antioxidant activity of Petasites japonicus flower buds cultivated in Tokushima, Japan, was examined in vitro and in vivo. The flower bud extracts were assayed using either oxygen radical absorbance capacity or 1,1-diphenyl-2-picrylhydrazyl (DPPH) radical scavenging activity. Antioxidants in the 80% ethanol extract were investigated using online high-performance liquid chromatography-DPPH and were identified as caffeic acid, 3-O-caffeoylquinic acid, fukinolic acid, 3,4-di-O-caffeoylquinic acid, 3,5-di-O-caffeoylquinic acid, and 4,5-di-O-caffeoylquinic acid using liquid chromatography–mass spectrometry. Fukinolic acid was the most active compound based on its activity and abundance. Administering the extracts orally to ICR mice prior to iron injection significantly suppressed plasma thiobarbituric acid reactive substance (TBARS) production. Moreover, TBARS and triglyceride concentrations in the plasma of C57BL/6 mice fed with a high fat diet were also significantly decreased by the extract. The results suggest that antioxidative compounds in P. japonicus can be used in the management of oxidative stress.
Graphical abstract
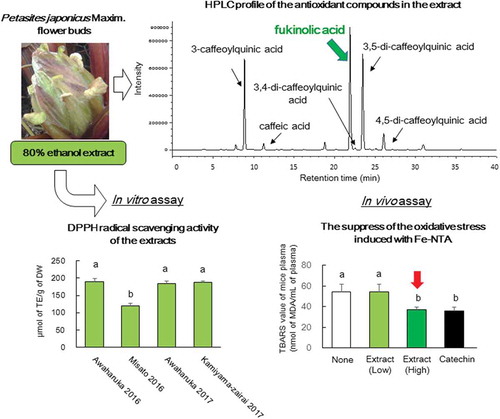
In vitro and in vivo evaluation of the antioxidant activity of Petasites japonicus Maxim. flower buds extracts
The flower bud of Petasites japonicus (Siebold et Zucc.) Maxim. is one of the edible parts of wild plants harvested in spring, in addition to bracken, osmund, and fatsia sprout. P. japonicus is cultivated in Aichi, Gunma, Osaka, and Fukuoka and a variety of “Aichi-wase” is widely distributed in Japan. The flower buds of “Aichi-wase” are large and vertically elongated; therefore, they are not considered as high-quality parts [Citation1]. Tokushima prefecture is a major production area for P. japonicus and its flower bud. The products are shipped to markets in the Keihanshin region. In Tokushima, three P. japonicus varieties, namely “Misato,” “Awaharuka,” and “Kamiyama-zairai,” are cultivated. Misato is pinkish on the lower part of the stem, and its edible stalk has high commercial value [Citation2]. Awaharuka has been cultivated for its high-quality flower buds, which have a suitable shape, and it has tightly closed petals. In addition, supplying the buds across the demand period from March to April is profitable after an extended harvest period from December to late March [Citation1]. Kamiyama-zairai is another variety of the wild type that is harvested in the Kamiyama area of Tokushima prefecture for its edible stalks.
P. japonicus has been used as a traditional Chinese medicine for the treatment of several conditions, including allergic rhinitis in East Asia countries such as China and Japan, for a long time. P. hybridus from Europe and America has been reported to influence seasonal allergic rhinitis [Citation3,Citation4]. Several studies on the antiallergenic properties of Petasites have been reported worldwide [Citation3–Citation6]. Furthermore, polyphenols in plant are reported to be associated with several bioactivities. The first report on polyphenol compounds from P. japonicus was by Sakamura S et al. [Citation7]. In addition, the antioxidant activity of polyphenol compounds has been investigated using in vitro assays on flavonoids such as quercetin glucosides [Citation8–Citation10] and kaempferol glucoside [Citation10], phenylpropanoids such as caffeic acids [Citation8,Citation9], petasiformin-A [Citation11], and fukinolic acid [Citation9,Citation10], which were identified as antioxidant compounds on the basis of 1,1-diphenyl-2-picrylhydrazyl (DPPH) radical scavenging activity or online 2,2′-azino-bis-3-ethylbenzthiazoline-6-sulfonic acid assay. However, most of these reports are regarding leaves and roots. To the best of our knowledge, there is little study that explores analytical research on flower buds [Citation8]. Moreover, no information on the antioxidant compounds of the above varieties produced in Tokushima is currently available. With regard to oxidative stress, the neuroprotective effects and antioxidative effects of petaslignolide-A or the butanol extract from the leaves of P. japonicus challenged with kainic acid have been reported in vivo in mouse brain [Citation12]. In addition, antioxidant activities of the methanol extract of P. japonicus Max. have been demonstrated in monosodium L-glutamate-challenged mice [Citation13]. However, systematic studies on the antioxidant activity of P. japonicus buds both in vitro and in vivo remain underreported.
In the present study, the antioxidant properties of the flower buds of different varieties of P. japonicus collected in Tokushima, namely “Awaharuka,” “Misato,” and “Kamiyama-zairai,” were evaluated in vitro. Subsequently, compounds with antioxidant activity in the 80% ethanol extracts from the flower buds were identified and quantified. The antioxidant properties of the extracts prepared from flower buds of “Awaharuka” were examined in vivo using iron (Fe)-induced acute oxidative injury in an animal model. Moreover, the effects of the extracts on biochemical markers of mice fed with normal or high-fat diets to induce obesity for 16 weeks, as models of chronic disorders, were assessed.
Materials and methods
Plant and authentic compounds
The flower buds of three varieties of P. japonicus (Misato, Awaharuka, and Kamiyama-zairai) were grown in Tokushima prefectural farms in January and February in 2016 and 2017. The fresh harvested flower buds of P. japonicus were immediately freeze-dried and crushed to powder using an Iwatani Millser IFM-800 DG (Iwatani Co., Saitama, Japan). These powders were stored at −30°C until subsequent experiments and analyses.
The following compounds were purchased for use in the identification of antioxidant components. Caffeic acid (CA) and 3-O-caffeoylquinic acid (3-CQA or chlorogenic acid) were obtained from Tokyo Kasei Co., Ltd. (Tokyo, Japan), 3,4-di-O-caffeoylquinic acid (3,4-diCQA), 3,5-di-O-caffeoylquinic acid (3,5-diCQA), and 4,5-di-O-caffeoylquinic acid (4,5-diCQA) were purchased from Wuhan ChemFaces Biochemical Co., Ltd. (Hubei, China). The five compounds were used as control standards for the preparation of calibration curves. High-performance liquid chromatography (HPLC)-grade acetonitrile and formic acid were purchased from Merck KGaA (Darmstadt, Germany) and Wako Pure Chemical Industries, Ltd. (Osaka, Japan). 6-Hydroxy-2, 5, 7, 8-tetramethylchroman-2-carboxylic acid (trolox) was purchased from Sigma-Aldrich Co. (St. Louis, MO, USA). DPPH was purchased from Wako Pure Chemical Industries, Ltd. (Osaka, Japan). (+)-Catechin was obtained from Cayman Chemical Company (Ann Arbor, MI, USA). All the other chemicals were of the highest commercially available grades.
Animals
Experimental mice were purchased from Charles River Laboratories Japan Inc. (Kanagawa, Japan). Male ICR mice aged 6 weeks were used to evaluate the antioxidant activity of extracts from flower buds against Fe-induced oxidative stress. Male C57BL/6 mice aged 5 weeks were used to evaluate the effects of the extracts on biochemical markers in mice fed with normal and high-fat diets. Mice were placed in clean and dry plastic cages under a 12-h light/12-h dark cycle at 25°C ± 2°C and 45–55% relative humidity in an animal house. MF were used for a normal diet and HFD-60 (60% kcal from fat) (Oriental Yeast Co., Tokyo, Japan) was used a high-fat diet. The experiments were approved by the Institutional Animal Ethics Committee and were performed according to the Animal Experiment Guidelines of Tokushima Bunri University (permission No. 15–6).
Preparation of extracts from flower buds of P. japonicus
Samples for lipophilic and hydrophilic oxygen radical absorbance capacity (L-ORAC and H-ORAC) assays were prepared according to the method by Watanabe et al. [Citation14]. Briefly, extracts from lyophilized P. japonicus flower buds were obtained using pressurized liquid extraction and hexane-dichloromethan (1:1) at 80°C. Hexane-dichloromethane extracts were dried under a nitrogen gas flow and redissolved in 5 mL of dimethyl sulfoxide. The resulting DMSO solution was diluted with 7% (w/v) randomly methylated cyclodextrin (Junsei Chemical, Co., Ltd., Tokyo, Japan). The extract was used for L-ORAC assay. Subsequently, methanol:distilled water:acetic acid (90:9.5:0.5, by volume) was added to the solid residue and extracted at 70°C for the H-ORAC assay. The extracts were stored at −80°C, and then thawed prior to measurements.
Samples for the DPPH assays and the identification and quantification of antioxidant compounds were prepared as follows. Eighty percent ethanol was added to 1 g of dried flower bud powder in a volume equivalent to 10 times the volume of dried flower bud powder weight, shaken on a shaking water bath (Personal-11, TAITEC Co., Ltd., Saitama, Japan) at 150 rpm for 30 min at 37°C, and then centrifuged at 12,000 rpm for 20 min at 4°C using a centrifuge (MX-307, TOMY SEIKO, Co., Ltd., Tokyo, Japan). After centrifugation, the flower buds were extracted two more times using the above method. Finally, the supernatants obtained after extraction for three times were pooled and adjusted to 0.1 mg powder/mL with 80% ethanol. For in vivo experiments, a total of 100 g of dry powder of flower buds was used for extraction. The supernatants obtained after 10 cycles of scale-up extractions were pooled to obtain a large amount of the sample. Ethanol in the supernatant was evaporated under reduced pressure at 40°C, and the concentration of supernatant was adjusted to 20 mg powder/mL with distilled water.
L-ORAC and H-ORAC assays
The antioxidant effects of P. japonicus flower bud extracts were evaluated using L-ORAC and H-ORAC assays as described by Watanabe et al. [Citation15,Citation16]. Briefly, an L-ORAC assay was performed on a multidetection microplate reader (SynergyTM HTX, BioTek Instruments, Inc., Tokyo, Japan), an incubator, and two injection pumps. The temperature of the incubator was set at 37°C. The sample, a blank, and trolox calibration solutions (each 35 µL) were diluted with PBS and transferred into 96-well microplates in duplicate and mixed with 115 μL of 110.7 nM fluorescein in PBS. After 10 min of incubation at 37°C, 50 μL of 31.7 mM AAPH was added to each well. Fluorescence intensity in each cycle was recorded at 485/528 nm (emission/excitation) and monitored every 2 min, with 60 cycles for 120 min. The calibration curves comprised various concentrations of trolox from 20 to 60 μM (coefficients of determination: R2 = 0.9943). The results were calculated on the basis of the differences in net area under the curve among the blank, samples, and standard solutions. Final ORAC values were expressed as μmol of trolox equivalents (TE)/100 g dry weight and μmol of TE/mM.
H-ORAC was performed as described above, except for different conditions with L-ORAC assay: 115 μL of 110.7 nM fluorescein, 50 μL of 31.7 mM AAPH, and 45 cycles for 90 min.
Measurement of DPPH radical scavenging activity
DPPH radical scavenging assay for the extracts and authentic standards were performed as described by Shimamura et al. [Citation17]. Briefly, 50 μL of each extract solution was mixed with 200 μL of 0.1 M Tris-HCl buffer (pH 7.4). Subsequently, 250 μL of 0.2 mM DPPH solution was added to obtain a final concentration of 0.1 mM DPPH. The reaction was allowed to proceed for 30 min at room temperature. The absorbance of the solutions was measured at 520 nm. The absorbance of the control was obtained by replacing the sample with methanol. Trolox was used as a control standard. Scavenging activity was calculated using the following formula:
HPLC analysis
Jasco HPLC (Jasco Corp., Tokyo, Japan) equipped with two PU-980 intelligent pumps, a DG-980-50 degasser, a CO-2060 plus column oven, an AS-4050 autosampler, an MD-2018 photodiode array detector (PDA) detector, and a ChromNAV v.2 data acquisition system was used for HPLC analyses.
The flower bud extracts prepared as previously described were filtered using a 0.45-μm Millex-LG filter (Millipore Co., MA, USA), and HPLC-PDA analysis was performed using a TSKgel ODS-80Ts column (5 μm, i.d., 4.6 × 250 mm) (Tosoh Co., Tokyo, Japan). The temperature of the column was maintained at 40°C. A spectrum was detected in the 200−700 nm range. Injection volumes of the extracts were set at 20 μL. Compounds were eluted using a gradient mobile phase comprising (A) 0.1% trifluoroacetic acid and (B) 80% acetonitrile/0.1% trifluoroacetic acid at a flow rate of 1 mL/min. The compounds were eluted on the basis of a linear gradient. The gradient was programmed as follows: initial 15% B; 15–35% B for 0–30 min; 35–80% B for 30–35 min; and 80% B isocratic for 5 min. The separated compounds were quantified at 325 nm.
The identities of major antioxidant compounds were determined on the basis of the congruence of retention times (Rt) and ultraviolet-visible (UV-vis) spectra and compared with those of pure authentic standards. To determine the quantities of these compounds, calibration curves were plotted for the compounds of interest using the peak area obtained from 31.25 to 500 µM of the standard solutions. Authentic 3-CQA, CA, 3,4-diCQA, 3,5-diCQA, and 4,5-diCQA were used as compounds 1, 2, 4, 5, and 6, respectively. A commercial compound corresponding to compound 3 could not be purchased. Therefore, the calibration curve of 3,4-diCQA, which has two molecules of CA and is similar to Rt, was used as a substitute. The standard solutions exhibited linear relationships between peak area and the amounts injected, with R2 ranging from 0.9951 to 0.9992. Quantification of each component was performed using the calibration curves, and the contents were expressed on the basis of mg/g dry weight.
HPLC-DPPH method
Antioxidant compounds were investigated using the online HPLC-DPPH method as previously described by Masuda et al. [Citation18]. Briefly, the extracts were prepared at 200 μg/mL in methanol, 120 μL of 10 mM DPPH solution was added to equal volumes of the extract solutions, and the mixtures were vigorously shaken and reacted at room temperature for 5 min in the dark. After reaction, the mixtures were filtered using 0.45-μm Millex-LG filters and subjected to HPLC analysis as described above. Methanol was used instead of the DPPH solution as the blank. Authentic compounds were also quantified using 10 mM solutions. Antioxidant activity was evaluated with a reduced rate as follows:
Ultraperformance liquid chromatography (UPLC)–diode array detector (DAD)–electrospray ionization (ESI)–mass spectrometry (MS) analysis
The 80% ethanol extracts from flower buds prepared as described previously were diluted with mobile phase A in the ratio of 1:1 and filtered using 0.2-μm Millex-LG filters and a UPLC-MS analysis was performed. An Acquity UPLC system coupled to a DAD detector and Xevo TQD (double quadrupole analyzer) mass spectrometer (Waters, Milford, MA, USA) equipped with Z-spray ESI source, operating in positive and negative modes, was employed for direct injection analyses. The analyses were performed using an Acquity UPLC HSS T3 column (1.8 μm, i.d. 2.1 × 100 mm) (Waters, Milford, MA, USA). Antioxidant compounds were eluted using a gradient mobile phase comprising (A) 0.1% formic acid and (B) 80% acetonitrile/0.1% formic acid at a flow rate of 0.3 mL/min and a column temperature of 40°C. The antioxidants were analyzed on the basis of a linear gradient. The gradient was programmed as follows: initial 10% B; 10–30% B for 0–10 min; 30–95% B for 10–12 min; and 95% B isocratic for 3 min. Detection wavelength was set in the 200–600 nm range. All injected solutions were stored at 4°C in an autosampler, and the injection volume was set at 10 µL. The ionization working conditions for the Xevo TQD mass spectrometer were set as follows: capillary voltage, 1 kV; collision energy, 30 V; cone gas flow rate, 0.5 mL/min; desolvation temperature, 400°C; and desolvation gas flow rate, 650 L/h. Data acquisition was performed using MassLynx™ v 4.1 (Waters, Milford, MA, USA). The peaks of compounds 1–6, excluding that of compound 3, were identified by matching Rt, UV-vis spectra, and mass spectral data with those of corresponding standards. However, identification of peaks of compound 3 was achieved by comparing Rt with tandem MS (MS/MS) spectra data from Human Metabolome Database [Citation19] and with those from previous reports [Citation20,Citation21] because a commercial standard corresponding to compound 3 could not be obtained.
Experiment with Fe-induced oxidation model animal
The antioxidant activities of the flower bud extracts were evaluated using mouse models through Fe-nitrilotriacetate (Fe-NTA)-induced renal oxidative injury. The mice were acclimatized to laboratory conditions for 1 week prior to experiments. Further, they were divided into four experimental groups (n = 4) as follows: a negative control, distilled water (NC); low-dose extract, extract equivalent to 4 g powder/kg body weight (Ex-L); high-dose extract, extract equivalent to 8 g powder/kg body weight (Ex-H); and a positive control, 0.25 mmol of (+)-catechin/kg body weight (PC). The animals were kept on fasting for 12 h prior to and during the experiments.
Mice were orally administered with test solutions 15 min prior to intraperitoneal injection of 10 mg of Fe/kg body weight. Finally, they were sacrificed after 60 min of Fe-NTA treatment, and their blood was collected in tubes treated with heparin. Plasma was obtained by centrifugation at 3,000 rpm for 10 min at 4°C, aliquoted, and frozen at −80°C until analysis. The amount of thiobarbituric acid reactive substances (TBARS), an indicator for lipid peroxidation in plasma, were measured as previously described by Ohkawa et al., 1979 [Citation22], and the data were expressed as nmol of malondialdehyde (MDA)/mL plasma. Before above-mentioned experiments, changes in TBARS values after treatment with or without Fe-NTA were assessed using the mice administered distilled water prior to the treatment.
Experiment with C57BL/6 mice fed with normal and high-fat diets
The mice were fed with MF diets and divided into four experimental groups (n = 8) as follows: normal diet with access to water (ND), normal diet with access to the extract (ND-Ex.), high-fat diet with access to water (HFD), and high-fat diet with access to the extract (HFD-Ex.). After 1 week, the diets of the high-fat diet group were switched from MF diet to HFD-60 diet. Subsequently, 1% extracts, which were equivalent to the dry bud powder weight diluted with sterilized water, were administered to C57BL/6 mice that were ad libitum fed with MF or HFD-60 diet for 16 weeks with water bottles. Sterilized water without the extract was also administered as a control. The diets and drinks comprising the extracts were exchanged with fresh ones every 3 days and administered to the mice, and the changes in weights and volumes were measured per cage. The bodyweight of every individual mouse was measured every week. The mice were kept on overnight fasting prior to the experiment. Further, their blood was collected by scarification, and the plasma was obtained as described previously. Subsequently, their kidneys and livers were removed, rinsed with physiological saline solution, and wiped with a filter paper, and their weights were subsequently measured.
TBARS and biochemical markers of plasma assay
TBARS assays were performed as described previously by Ohkawa et al. [Citation22], with minor modifications. Briefly, 25 μL of 8.1% sodium dodecyl sulfate and 200 μL of 0.05 N HCl were added to 20 μL of the plasma or standard or distilled water in a tube. After vortexing, the tubes, 66 μL of 0.67% 2-thiobarbituric acid (TBA) dissolved in 0.025 N NaOH or 0.025 N NaOH without TBA was added and heated at 95°C for 30 min. After the reaction was stopped on ice for 5 min, the tubes were centrifuged at 3,000 rpm for 10 min, and absorbance of the supernatants was measured at 532 nm using a spectrophotometer (Ultraspec 3100 pro, Amersham Pharmacia Biotech Inc. Uppsala, Sweden) using distilled water as the blank. Tetramethoxy-propane was used as an external standard in the 3.125–200 nmol/mL range, and lipid peroxide concentrations were expressed as nmol MDA/mL plasma.
Plasma glucose, triglyceride, total cholesterol, and high-density lipoprotein (HDL)-cholesterol concentrations were enzymatically quantified using Glucose CII Test (Wako Pure Chemical Industries, Ltd., Osaka, Japan), Triglyceride E-Test Wako (Wako Pure Chemical), Cholesterol E-Test Wako (Wako Pure Chemical), and HDL-Cholesterol E-test (Wako Pure Chemical), respectively. Low-density lipoprotein (LDL)-cholesterol concentrations were calculated using the Friedewald formula [Citation23] as follows:
Statistical analysis
Data were presented as mean ± standard deviation (SD) of three determinations, and as mean ± SD of four or eight mice for the in vitro and in vivo assays, respectively. Statistical analyses were performed using the Tukey–Kramer multiple comparison post hoc test following one-way ANOVA. Student’s t-test was performed to compare the two groups. A P value of <0.05 was considered statistically significant. The results were calculated using IBM SPSS Statistics v.24 (IBM Corp., Armonk, NY, USA).
Results and discussion
Antioxidant activity of flower bud extracts
The antioxidant activities of the flower bud extracts were evaluated using L-ORAC, H-ORAC, and DPPH radical scavenging assays (). Harvested Awaharuka exhibited the highest total ORAC values among three cultivars in both years. Conversely, Misato exhibited the lowest total ORAC values (). Most ORAC values were attributed to H-ORAC among all the samples. The results indicate that antioxidants in the extracts were hydrophilic compounds. The trends in the results of the DPPH radical scavenging assay were consistent with those of the ORAC assay (). Quercetin glucosides [Citation8,Citation10], CAs [Citation8], CQA and diCQAs [Citation9,Citation10], and fukinolic acid [Citation9,Citation10], which were identified as the antioxidant compounds in P. japonica and its sub-species, were hydrophilic. Therefore, the compounds can be associated with the H-ORAC values and DPPH radical scavenging activity.
Figure 1. Antioxidant activity of the flower bud extracts of different P. japonicus varieties produced in Tokushima.
The extracts from Awaharuka 2016, Misato 2016, Awaharuka 2017, and Kamiyama-zairai 2017 were assayed. Total ORAC values from L-ORAC (closed square) and H-ORAC (open square) (a) and DPPH radical scavenging activity (b). Values were expressed as mean ± SD of three determinations, and different letters indicate significant differences among extracts, p< 0.05 (Tukey–Kramer multiple-range test).
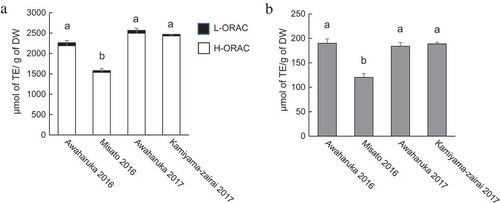
HPLC analysis of the extracts and antioxidant compound identification using HPLC-DPPH analysis
HPLC analysis of the compounds in the extracts was performed (). The HPLC profiles of the untreated extracts from P. japonicus Awaharuka buds were similar to those of the other two varieties, Misato and Kamiyama-zairai. Several peaks were detected in the extracts and six peaks of among them were labeled as peaks 1–6, as shown in . Maximal absorption of those compounds including compound 3 in the peak 3 was observed at 324 or 328 nm on the basis of the UV-vis spectral analysis (), which corresponded to the λ-max of CQA and diCQA [Citation10], thereby suggesting the presence of CQA in the compounds. Several authentic compounds related to CQA, including CA, 3-CQA, 3,4-diCQA, 3,5-diCQA, and 4,5-diCQA, were also subjected to HPLC analysis, and the peaks of the compounds were compared with the Rt and UV-vis spectra of peaks 1–6, as shown in . The Rt and UV-vis spectra of peaks 1, 2, 4, 5, and 6 were identical to those of authentic 3-CQA, CA, 3,4-diCQA, 3,5-diCQA, and 4,5-diCQA, respectively. Although peak 3 was a main compound in the extract and its Rt was similar to that of 3, 4-diCQA, it did not coincide with any authentic compounds including 3, 4-diCQA.
Figure 2. Antioxidant activity of the flower bud extracts of P. japonicus based on the online HPLC-DPPH method.
Antioxidant compounds in the 80% ethanol extracts from Awaharuka were investigated using the online HPLC-DPPH method. HPLC profiles of compounds treated with DPPH or without DPPH (a). UV-vis spectra of six major peaks and tentative commercial compounds (b). The compounds in the extract and authentic compounds were separated on a TSK ODS 80T column and monitored at 325 nm.
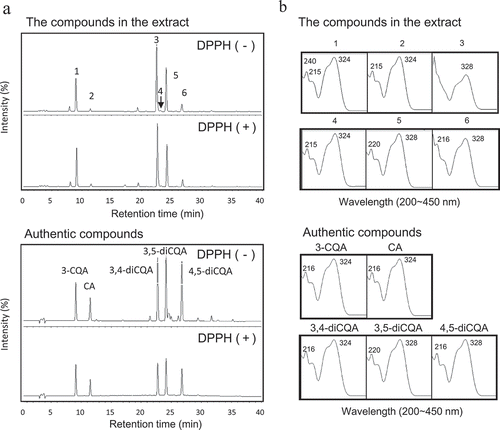
The peak areas of peaks 1–6 decreased after DPPH treatment, as shown by the online HPLC-DPPH analysis, compared with those of peaks untreated with DPPH in . The results imply that antioxidant compounds were contained in the peaks. Among the peaks, peak 3 exhibited the highest antioxidant activity in the extracts, as shown in , followed by peaks 5 and 6. The antioxidant activity of peak 4 could not be calculated because the peak area in the extract was too small. In another experiment, the antioxidant activities of each fraction of the extract corresponding to the peaks also showed a trend similar to that of the online HPLC-DPPH method. The inhibition (%) of each fraction containing peaks 1–6 were: 21.5%, 16.7%, 53.2%, 7.0%, 41.5%, and 10.6%, respectively. In addition, DPPH treatment also decreased the peak areas of five authentic compounds, and the diCQAs particularly exhibited the antioxidant activity ( and ). Therefore, the compounds are also candidate antioxidant compounds corresponding to peaks 1, 2, 4–6, in the flower bud extracts.
Table 1. DPPH radical scavenging activity based on six major peaks in the extracts of P. japonicus (a) and five authentic compounds (b) in . Antioxidant activity was examined using the online HPLC-DPPH method.
Identification of antioxidant compounds by MS and MS/MS analysis
To identify the antioxidant compounds in the peaks detected in , further UPLC-ESI-MS analysis of each peak was performed. The mass data for each peak are summarized in (each mass data; and Supplemental Figures 1–6). Molecular mass ions [M + H]+ of compounds 1–6 were 355.2, 181.1, 435.3, 517.3, 517.3, and 517.3, respectively. Molecular mass ions [M–H]– of compounds 1–6 were 353.3, 179.1, 433.2, 515.4, 515.4, and 515.4, respectively. Authentic compounds 3-CQA, CA, 3,4-diCQA, 3,5-diCQA, and 4,5-diCQA were also subjected to MS analysis, and their mass ions were compared. Compounds 1, 2, 4, 5, and 6 were identified as 3-CQA, CA, 3,4-diCQA, 3,5-diCQA, and 4,5-diCQA, respectively. Among them, compounds 4, 5, and 6 were found to be derivatives of 3-CQA, which has two CA molecules binding to quinic acid. The compounds are structural isomers of each other.
Table 2. UPLC-MS data of the antioxidant compounds detected and estimated in authentic compounds in .
Figure 3. Spectra data of UPLC-MS and MS/MS analysis of peak 3.
UV-vis spectrum (a) and MS spectra with ES negative (b) and positive mode (c). MS/MS spectra data showing the molecular ion mass of a parent ion as 435.2 with ES positive mode at 30 V (d) and as 433.3 with ES negative mode at 30 V (e).
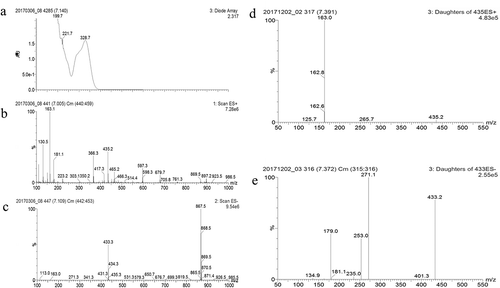
Compound 3 exhibited the highest antioxidant activity and was subjected to MS and MS/MS. The molecular mass ions of compound 3 in positive and negative modes were observed at m/z 435.3 [M+H]+ and 433.2 [M–H]–, respectively (, ). This suggested that compound 3 has a molecular mass of 434. An ion at m/z 867.5 was considered as a dimer ion 867.5 [2M−H]− of 433.2 [M−H]− formed during the electrospray process. In addition, the [M–H]− of 433.3, corresponding to the parent ion of peak 3, produced fragment ions with m/z of 271.1, 253.0, 179.0, and 134.9 following MS/MS analysis in negative mode (). The fragment ions corresponded to [fukinolic acid–caffeoyl–H]−, [fukinolic acid–CA–H]−, [CA–H]−, and [CA–CO2–H]−, respectively. Moreover, a fragment ion m/z of 163.0 [CA–H2O+H]+ as a fragment from the parent ion with [M+H]+ of 435.2 was observed in positive mode (). The UV-vis spectrum () and MS/MS fragment patterns were consistent with the fukinolic acid data reported by Wang et al. [Citation20], He et al. [Citation21], and Human Metabolome Database Version 4 [Citation19]. In another experiment, compound 3 isolated from the extract was hydrolyzed under an acidic condition, which resulted in the formation of CA (data not shown). Fukinolic acid exists in Petasites species with a molecular mass of 434, and it comprises the CA molecule. Fukinolic acid is also reported to be an antioxidant [Citation9,Citation10] and has a higher antioxidant activity than chlorogenic acid [Citation9]. Therefore, compound 3 was considered to be fukinolic acid. Because there are no commercial fukinolic acid products available, fukinolic acid was identified using MS/MS analysis.
Some functions of fukinolic acid have been identified in vitro and through studies using cells, such as the inhibition of NO production [Citation9], antiEV-A71 [Citation24], and anti-inflammatory activities [Citation20]. The radical scavenging activity of fukinolic acid is lower than that of epigallocatechin gallate, which is a strong antioxidant; however, it is higher than that of 3-CQA on the basis of a cell assay.
Contents of antioxidant compounds in the extracts
Contents of the flower bud extracts of the three varieties are shown in . The concentration ratios of CQAs was similar among all the extracts, and fukinolic acid was present in the highest concentration, whereas 3,5-diCQA and 3-CQA had the second or third highest concentration. The total content of antioxidant compounds was highest in Awaharuka. In addition, the concentrations of the antioxidants were highly correlated with antioxidant activity (). This observation is widespread in several foods, and therefore, it is suggested that the compounds were closely related to their antioxidant activity. In addition, the compounds detected in the extracts were similar to those reported for P. japonicus leaves and/or stem [Citation6,Citation7,Citation9]; however, it differed from the flower buds of subsp. gigantea Kitam [Citation8]. Fukinolic acid was not detected in the flower buds of gigantea Kitam, although quercetin glucosides and rutin were detected, which were not detected in the present study as well as in some previous studies [Citation6,Citation7,Citation9]. In addition, Choi et al. characterized the polyphenols in the leaves, stems, and roots of Korean P. japonicus [Citation25] and reported diCQA and naringenin hexoside as major compounds in three tissues. Fukinolic acid was not detected in the sample. The differences in the polyphenol composition may depend on the variant. However, Choi et al. performed the identification of the compounds in the Korean P. japonicus by MS/MS analysis. Although 433 [M–H]− of naringenin hexoside is the same as that of fukinolic acid and the MS/MS spectra data is also similar, the difference was not clearly described. Therefore, the possibility that fukinolic acid has been identified as naringenin hexoside cannot be denied. However, it is unclear whether the reason for the difference in components is due to differences in cultivated land or varieties.
Table 3. Concentrations of antioxidant compounds in the flower bud extracts of various P. japonicus varieties produced in Tokushima.
Effect on antioxidant activity of the flower bud extract in ICR mice
The plasma TBARS values of oxidative stress in the mouse model treated with Fe-NTA are illustrated in . TBARS value was increased approximately 6.5 times in the Fe-NTA-treated group compared with that in the untreated group (). The TBARS values following oral administration of high-dose extracts (8 g dry powder base/kg) prior to the injection of Fe-NTA decreased by 31.1% compared with the control group, which is similar that observed in the catechin-treated group (33.6% reduction), thus indicating that the flower bud extracts have antioxidative effects under acute oxidative stress conditions (). Plasma TBARS values of mice fed with diets comprising P. japonicus leaf extracts decreased [Citation13]; in addition, the butanol fraction in these extracts also reduced TBARS value in brain tissue [Citation12]. However, Han et al. have reported an increase in hepatic TBARS values following diets with 5% acetone extract of P. japonicus leaf powder, as revealed by the presence of pyrrolizidine alkaloids (PA) [Citation26]. Therefore, considering that a high amount of antioxidants were required to suppress the acute reaction, the amount of the toxic compound present in the P. japonicus flower bud extracts should be considered.
Figure 4. Plasma TBARS values in the oxidative stress mouse model treated with Fe-NTA.
Changes in TBARS values after treatment with Fe-NTA (a); effects of sample extract on TBARS values (b). In experiment (a), 7-week-old male ICR mice were orally administered distilled water 15 min prior to intraperitoneal injection of Fe-NTA. After 60 min, the Fe-NTA-treated and Fe-NTA-untreated mice were sacrificed, and their plasma TBARS values were measured. In experiment (b), mice were administered distilled water (NC), low and high-dose extracts (Ex-L and Ex-H; 4 and 8 g dry powder base/kg, respectively), and catechin 0.25 mmol/kg and then treated with Fe-NTA. Data are expressed as mean ± SD of four mice. Significant differences were detected by Student’s t-test and Tukey–Kramer multiple-range test at p < 0.05.
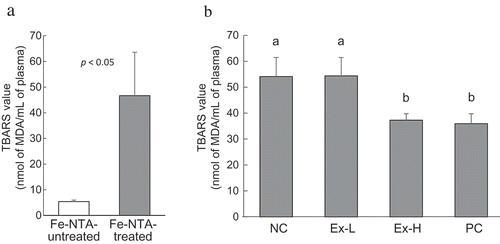
Effect on antioxidant activity of flower bud extracts in C57BL/6 mice fed with high-fat diets
The effects of the flower bud extracts on chronic disorders such as obesity were examined in mice fed with high-fat diets. As shown in , the extracts did not influence body weight or weight gain in mice fed with the normal or high-fat diet and had access to the extracts for 15 weeks. In addition, compared with water, the extracts showed no significant influences on the liver and kidney weights of the mice fed with either diet. Although the food intake was higher in the mice fed with high-fat diets and extracts than in those with no access to the extract, no significant difference was observed in weight gain compared with the control group. While energy expenditure is reportedly upregulated by flavonoids such as quercetin [Citation27], quercetin concentrations were very low in the flower bud extracts prepared in the present study. Moreover, it has been reported that CA and 3-CQA improve body weight, lipid metabolism, and obesity-related hormone levels in mice fed with high-fat diets [Citation28]. However, the extracts did not reduce the body weights of mice in term of the concentrations used in the present study. The difference between the results of previous reports and our study can be due to varying amounts of food intake or differences in animal species; C57BL/6 mice in this work intake approximately 4 times more energy than ICR mice used in the previous report.
Table 4. Body and tissue weights and food and extract intakes in mice fed with normal and high-fat diets.
Ingestion of the extract did not affect the plasma TBARS values in C57BL/6 mice fed with normal diet. However, plasma TBARS values of C57BL/6 mice fed with high-fat diets were considerably low when the mice ingested extracts (). In addition, triglyceride concentrations in the plasma of mice fed with high-fat diets with the bud extract decreased, thereby indicating the potential antiobesity effects of the flower bud extracts. Watanabe et al. have reported that the administration of diets comprising P. japonicus ethanol extracts resulted in a decrease in weight gain, visceral fat accumulation, plasma cholesterol, and glucose concentrations in mice fed with a high-fat diet [Citation29]. Although they hypothesized that the associated compounds were quercetin glucosides, CA, and lignans in P. japonicus, they were not studied in detail.
Table 5. Plasma TBARS and biochemical markers in mice fed with normal and high-fat diets.
To examine the safety of such compounds, e.g., PA, the liver and kidney weights in the mice were compared. As described above, the tissue weights were not difference and a disorder of appearance was also not observed. PA are toxic and can cause liver damage and cancer [Citation30–Citation32]. Several types of PA have been identified, and they are mainly found in plant families such as Asteraceae, Aabaceae, and Oraginaceae. Although the concentrations of PA are not adequate to cause acute poisoning in most cases, PA are frequently consumed in quantities that exceed the maximum daily intake recommended by authorities, which can be a contributing factor to chronic syndromes. Recently, concerns regarding PA have increased in Japan. The concentrations of PA in P. japonicus buds range from 2.1 to 330 mg/kg fresh weight, which is higher than those in the stalk and leaf [Citation33]. PAs in P. japonicus mainly comprise petasitenine, neopetasitenine, and senkirukin, and their molecular weights are 381, 423, and 365, respectively. In the present study, scanning of the MW of the above PA was performed with LC-MS analyses of the extracts. Although the corresponding MS signals were detected, the concentrations were too limited to be quantified, as shown in a previous report [Citation33]. Therefore, the intake of such extracts may be considered safe for humans. However, attention must be paid to the use of large amounts of the extract at once for individuals, particularly for patients with diseases, pregnant women, or children.
The concentrations of PA can be decreased to 20% using a combination of boiling and simmering in tap water for 20–22 h, thereby indicating that PA have high hydrophilicity and can be lost in the tap water in this process [Citation33]. Therefore, before consuming such flower buds or their extracts to benefit from the antioxidative properties of the compounds, the concentrations of PA should be reduced by prior soaking of the flower buds in water. Consequently, the residual quantities of bioactive compounds must be evaluated after the boiling and simmering treatments in the next plan.
In the present study, we evaluated the antioxidant activity of extracts of P. japonicus flower buds harvested in Tokushima prefecture and identified the antioxidant compounds. The extracts, after their oral administration, showed antioxidant activity in an Fe-induced oxidative injury animal model. Furthermore, the long-term intake of the flower bud extracts in obese mice decreased the plasma TBARS values and triglyceride concentrations, with no toxic symptoms. The P. japonicus flower bud extracts can be a useful bioresource for the production of functional ingredients.
Author contributions
M. Hiemori-Kondo (primary author of the study) designed the study, collected and interpreted test data, and drafted the manuscript. M. Nii collected the plant sample and performed the ORAC assay.
Supplemental_figure_6.pdf
Download PDF (126.6 KB)Supplemental_figure_5.pdf
Download PDF (127.9 KB)Supplemental_figure_4.pdf
Download PDF (133 KB)Supplemental_figure_3.pdf
Download PDF (129.4 KB)Supplemental_figure_2.pdf
Download PDF (133.7 KB)Supplemental_figure_1.pdf
Download PDF (306.5 KB)Acknowledgments
This work was supported by the 2016 Regional Revitalization Grant, Agriculture, Forestry and Fisheries Open Innovation Promotion Project “Search and display support for highly functional agricultural products.” We would also like to thank N. Yamashita, H. Uehara and D. Shinya for their assistance in animal experiments.
Disclosure statement
No potential conflict of interest was reported by the authors.
Supplementary material
Supplementary data for this article can be accessed here.
Additional information
Funding
References
- Takagi K, Kosumi J, Takeuchi T, et al. Breeding of a new fuki (Petasites japonicus (Sieb. et Zucc.) Fr. Schmidt) cultivar for fukinotou, “AWAHARUKA”. Bull Tokushima Agric For Fish Technol Support Cent. 2014;1:1–6. Japanese.
- Takagi K, Kosumi J. Breeding of a new fuki (Petasites japonicus (Sieb. et Zucc.) Fr. Schmidt) cultivar for yama-buki, “MISATO”. Bull Tokushima Agric For Fish Technol Support Cent. 2005;2:23–28. Japanese.
- Lee DK, Carstairs IJ, Haggart K, et al. Butterbur, a herbal remedy, attenuates adenosine monophosphate induced nasal responsiveness in seasonal allergic rhinitis. Clin Exp Allergy. 2003;33:882–886.
- Schapowal A. Randomised controlled trial of butterbur and cetirizine for treating seasonal allergic rhinitis. BMJ. 2002;324:144–146.
- Brattstrom A. A newly developed extract (Ze 339) from butterbur (Petasites hybridus L.) is clinically efficient in allergic rhinitis (hay fever). Phytomedicine. 2003;10:50–52.
- Shimoda H, Tanaka J, Yamada E, et al. Anti type I allergic property of Japanese butterbur extract and its mast cell degranulation inhibitory ingredients. J Agric Food Chem. 2006;54:2915–2920.
- Sakamura S, Yoshihara T, Toyoda K. The constituents of Petasites japonicus: structures of fukic acid and fukinolic acid. Agric Biol Chem. 1973;37:1915–1921.
- Matsuura H, Amano M, Kawabata J, et al. Isolation and measurement of quercetin glucosides in flower buds of Japanese butterbur (Petasites japonicus subsp. gigantea Kitam.). Biosci Biotechnol Biochem. 2002;66:1571–1575.
- Watanabe S, Hashimoto K, Tazaki H, et al. Radical scavenging activity and inhibition of macrophage NO production by fukinolic acid, a main phenolic constituent in Japanese butterbur (Petasites japonicus). Food Sci Technol Res. 2007;13:366–371.
- Kim SM, Kang SW, Jeon JS, et al. Rapid identification and evaluation of antioxidant compounds from extracts of Petasites japonicus by hyphenated-HPLC techniques. Biomed Chromatogr. 2012;26:199–207.
- Lin CH, Li CY, Wu TS. A novel phenylpropenoyl sulfonic acid and a new chlorophyll from the leaves of Petasites formosanus KITAMURA. Chem Pharm Bull. 2004;52:1151–1152.
- Cui HS, Kim MR, Sok DE, et al. Protection by petaslignolide A, a major neuroprotective compound in the butanol extract of Petasites japonicus leaves, against oxidative damage in the brains of mice challenged with kainic acid. J Agric Food Chem. 2005;53:8526–8532.
- Park CH, Kim MY, Sok DE, et al. Butterbur (Petasites japonicus Max.) extract improves lipid profiles and antioxidant activities in monosodium L-glutamate-challenged mice. J Med Food. 2010;13:1216–1223.
- Watanabe J, Oki T, Takebayashi J, et al. Extraction efficiency of hydrophilic and lipophilic antioxidants from lyophilized foods using pressurized liquid extraction and manual extraction. J Food Sci. 2014;79:C1665–C1671.
- Watanabe J, Oki T, Takebayashi J, et al. Improvement and interlaboratory validation of the lipophilic oxygen radical absorbance capacity: determination of antioxidant capacities of lipophilic antioxidant solutions and food extracts. Anal Sci. 2016;32:171–175.
- Watanabe J, Oki T, Takebayashi J, et al. Method validation by interlaboratory studies of improved hydrophilic oxygen radical absorbance capacity methods for the determination of antioxidant capacities of antioxidant solutions and food extracts. Anal Sci. 2012;28:159–165.
- Shimamura T, Matsuura R, Tokuda T, et al. Comparison of conventional antioxidant assays for evaluating potencies of natural antioxidants as food additives by collaborative study. J Jpn Soc Food Sci. 2007;54:482–487. Japanese.
- Masuda T, Inouchi T, Fujimoto A, et al. Radical scavenging activity of spring mountain herbs in the Shikoku mountain area and identification of antiradical constituents by simple HPLC detection and LC-MS methods. Biosci Biotechnol Biochem. 2012;76:705–711.
- Human metabolome database (HMDB) version 4 [Internet]. Canada: Reagents of The Metabolomics Innovation Centre (TMIC); 2018 [cited 2019 May 20]. Available from: http://www.hmdb.ca/spectra/ms_ms/164449
- Wanga ZY, Wanga Q, Hanb YQ, et al. Bioactivity-based UPLC/Q-TOF/MS strategy for screening of anti-inflammatory components from Cimicifugae Rhizoma. Chin Chem Lett. 2017;28:476–481.
- He K, Pauli GF, Zheng B, et al. Cimicifuga species identification by high performance liquid chromatography-photodiode array/mass spectrometric/evaporative light scattering detection for quality control of lack cohosh products. J Chromatogr A. 2006;1112:241–254.
- Ohkawa H, Ohishi N, Yagi K. Assay for lipid peroxides in animal tissues by thiobarbituric acid reaction. Anal Biochem. 1979;95:351–358.
- Friedewald WT, Levy RI, Fredrickson DS. Estimation of the concentration of low-density lipoprotein cholesterol in plasma, without use of the preparative ultracentrifuge. Clin Chem. 1972;18:499–502.
- Ma Y, Cong W, Huang H, et al. Identification of fukinolic acid from Cimicifuga heracleifolia and its derivatives as novel antiviral compounds against enterovirus A71 infection. Int J Antimicrob Agents. 2019;53:128–136.
- Choi JY, Desta KT, Saralamma VVG, et al. LC–MS/MS characterization, anti-inflammatory effects and antioxidant activities of polyphenols from different tissues of Korean Petasites japonicus (Meowi). Biomed Chromatogr. 2017;31:1–9.
- Han KH, Sekikawa M, Shimada K, et al. Japanese butterbur (Petasites japonicus) leaves increase hepatic oxidative stress in male rats. Biosci Biotechnol Biochem. 2012;76:2026–2031.
- Kawser Hossain M, Abdal Dayem A, Han J, et al. Molecular mechanisms of the anti-obesity and anti-diabetic properties of flavonoids. Int J Mol Sci. 2016;17:569–600.
- Cho AS, Jeon SM, Kim MJ, et al. Chlorogenic acid exhibits anti-obesity property and improves lipid metabolism in high-fat diet-induced-obese mice. Food Chem Toxicol. 2010;48:937–943.
- Watanabe T, Hata K, Hiwatashi K, et al. Suppression of murine preadipocyte differentiation and reduction of visceral fat accumulation by a Petasites japonicus ethanol extract in mice fed a high-fat diet. Biosci Biotechnol Biochem. 2010;74:499–503.
- Hirono I, Mori H, Yamada K, et al. Carcinogenic activity of petasitenine, a new pyrrolizidine alkaloid isolated from Petasites japonicus Maxim. J Natl Cancer Inst. 1977;58:1155–1157.
- Hirono I, Haga M, Fujii M, et al. Induction of hepatic tumors in rats by senkirkine and symphytine. J Natl Cancer Inst. 1979;63:469–472.
- Chen T, Mei N, Fu PP. Genotoxicity of pyrrolizidine alkaloids. J Appl Toxicol. 2010;30:183–196.
- Ministry of Agriculture, Forestry and Fisheries. Survey of the content of pyrrolidine alkaloids in the domestic butterbur (Japanese) [Internet]; 2018 Aug 31 [cited 2019 May 20]. Available from: http://www.maff.go.jp/j/press/syouan/nouan/180831.html.