ABSTRACT
To elucidate the role of POU2F2 (POU class 2 homeobox 2) in fracture healing, 30 rats with femoral fracture were randomly grouped into three groups: FF group, LV-POU2F2 group and LV-scramble group. Rats were injected with PBS, lentivirus expressing POU2F2 or scramble lentivirus once a week for 4 weeks. Results showed that overexpressing of POU2F2 promoted fracture healing and callus growth. Besides, overexpressing of POU2F2 promoted protein and mRNA expression of Col10a1, Runx2, Osterix, and Osteocalcin. High Mobility Group AT-hook 1 (HMGA1) is a non-histone protein participating in chromatin remodeling of cells. Western blotting manifested HMGA1/Wnt/β-catenin pathway was activated in POU2F2 group. Moreover, in-vitro study of hMSCs cells supported the above data. In conclusion, POU2F2 promotes fracture healing via activating the HMGA1/Wnt/β-catenin signaling pathway.
Graphical abstract
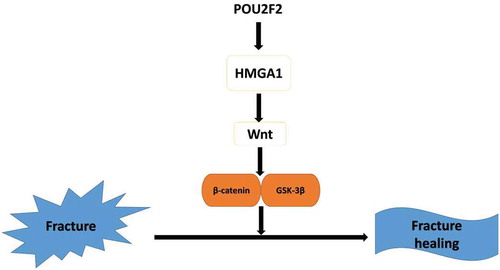
Overexpressing of POU2F2 accelerating fracture healing via regulating HMGA1/Wnt/β-catenin signaling pathway
Fracture healing is a complex physiological procedure, involving various intracellular and extracellular signaling pathways, which occur in different stages of fracture healing of different parts of bones [Citation1]. Fracture healing can be divided into direct healing and indirect healing. Direct healing is not common in patients, and it only occurs when the fracture ends have anatomical reduction, no gap exists between fracture ends and internal fixation is very strong. When the fracture ends do not have an anatomical reduction, a gap exists between fracture ends, or internal fixation is not very strong, indirect healing happens, and it is the most common type of fracture healing in patients [Citation2]. Different biomechanical environments can cause different healing environments of fracture sites, thus leading to different types of fracture healing.
Despite of the progress made in molecular biology and medical engineering, the concrete molecular mechanisms of fracture healing have not been clearly elucidated. The mortality rate of multiple-trauma patients has significantly reduced resulting from the continuous development of medical science. However, the incidence of un-union fracture healing increases as the number of multiple-trauma patients increases [Citation3]. In all fracture patients, the incidence of nonunion fracture healing remains about 10–20% [Citation4]. The incidence of delay fracture healing and un-union fracture healing is about 10–20% of long bone fracture, and it is the main reason leading to the morbidity and disability of patients [Citation5]. Therefore, how to efficiently promote fracture healing remains a huge challenge for orthopedists.
POU2 is the second category of POU family, including Oct-1, Oct-2, and Oct-11. Among them, POU2F2 (POU class 2 homeobox 2) is more widely known as Oct-2(Octamer-binding protein 2). In human, POU2F2 gene is located on chromosome 19, including 15 exons. Via different ways of post-transcriptional splicing, POU2F2 gene can encode 3 similar OCT isoforms [Citation6]. By specifically identifying the 5ʹ-ATTTGCAT-3ʹ region of DNA of cells or pathogens, POU2F2 can regulate the expression of target genes [Citation7–Citation9]. Bioinformatic analysis reveals that the expression of POU2F2 is decreased in nonunion skeletal fractures, suggesting that it be closely related to fracture healing [Citation10]. Therefore, we want to focus on the effect of POU2F2 in fracture healing in our research, and explore the related mechanisms.
HMG (high mobility group protein) is a kind of protein family including various non-histone proteins participating chromatin remodeling of cells, and they are named as they can move fast in PAGE gel. HMG includes HMGA1 and HMGA2 according to different encoded genes. Via its specific functional AT-hook region, HMGA1 can modify the binding sequences and accelerate various DNA-dependent effects, such as transcription, replication, recombination, and repair [Citation11]. According to previous reports, POU2F2 regulates the expression of HMGA1 [Citation8], and HMGA1 participates in abundant cellular biological processes, including cell cycle [Citation12,Citation13], cell differentiation [Citation14], cell apoptosis [Citation15] and cell metabolism [Citation16], which are all key steps during skeletal fracture healing. Above all, we put forward the hypothesis that the POU2F2/HMGA1 may play a vital role in fracture healing.
In this paper, we explore the influence of POU2F2 on skeletal fracture healing as well as related molecular mechanisms. The purpose of this research is to make up for deficiencies of POU2F2 in fracture healing and provide novel treatments and research directions for accelerating fracture healing, decreasing delay fracture healing or un-union fracture healing.
Materials and methods
Cell culture
hMSCs cell line was obtained from ATCC. Cells were cultured in Alpha Modified Eagle’s Medium (Gibco) with 10% FBS (Gibco) and 1% penicillin and streptomycin (Gibco). The cells were cultured under the environment of 5% CO2 and 95% humidity.
Lentiviral production and transfection
The lentiviral vector carrying the POU2F2 gene (LV-POU2F2) and the scramble lentiviral vector were obtained from Genepharma (Shanghai, China). The lentiviral vectors were transfected into hMSCs cells with 5μg/mL polybrene (Genepharma, Shanghai, China).
Animals and grouping
This study has received approval of the Animal Ethics Committees of the second affiliated hospital of Nanjing Medical University (Ethical approval number, 2018–16). In this research, thirty 12-week-old male Sprague-Dawley rats were bought from the SLAC Laboratory Animal Co., Ltd. (Shanghai, China). First, we performed a femoral fracture at the right lower limb of all 30 rats. After anesthetization using 3% pentobarbital sodium (0.1g/kg) (Sigma-Aldrich, St. Louis, MO, USA), the middle limb was transected. Then, the fracture was fixed with a bone nail. On the next day, we verified the success of modeling via X-ray examination. All the 30 rats were then divided randomly into three groups (10 rats in each group): FF group, LV- POU2F2 group and LV-scramble group. For each group, after the establishment of a femoral fracture, the rats were injected with 10μL of PBS, lentivirus expressing POU2F2 (1 × 109 UT/mL) and scramble lentivirus (1 × 109 UT/mL) at the fracture site each week, respectively. Lentivirus expressing POU2F2 and scramble lentivirus were obtained from GenePharma Co., Ltd. (Shanghai, China).
X-ray and micro-CT images of fracture callus formation
At each week after the operation, a digital X-ray machine (52 kV, 4.5 mAs, 0.5 s) was used to obtain images of the fracture site of rats. Afterward, images of callus were scored for analysis: 1, no obvious callus; 2, slight intramembranous ossification; 3, hard callus without bridging of the fracture gap, fracture line is apparent; 4, hard callus with bridging of the fracture gap, fracture gap is noticeable; 5, unclear boundary between the newly formed hard callus and existing cortical bone; and 6, remodeling [Citation17]. The above radiographic score was assessed by two orthopedic surgeons independently. Moreover, the external callus width (CW) was evaluated on the basis of digitized lateral X-ray images. Namely, CW = maximum outer diameter of the external mineralized callus – width of cortical bone.
In addition, a micro-CT machine (PerkinElmer, Waltham, MA, USA) was used to obtain images of the fractured femurs. Analyze 12.0 software (PerkinElmer) was used to evaluate the callus volume.
HE and safranin-O/Fast Green staining
After the last scan of X-ray machine, the rats were sacrificed and bone callus was obtained. Then, bone callus was fixed in 10% formaldehyde solution for 24 h, demineralized in 5% EDTA-2Na for 14 d, dehydrated via ethanol, cleared via xylene, embedded in paraffin, and sliced into 5-μ m-thick sections. The sections were used for H&E as well as Safranin-O/Fast Green staining according to manufacturers’’ protocols. Finally, an optical microscope (Olympus, Beijing, China) was used to observe the sections.
RT‐qPCR
After obtainment, bone callus was put in liquid nitrogen. Trizol (Invitrogen Inc, Carlsbad, CA, USA) was used to extract total RNA of bone callus. After quantification, RNA was reverse transcribed into cDNA using the kit from Takara (Dalian, China). PCR reaction was then performed using the PCR kit (Takara, Dalian, China) according to manufacturers’ instructions. β-actin was used as an internal reference. Primes used in the research were shown in .
Table 1. Primer sequences for RT‐qPCR.
Western blot
After bone callus was obtained, they were lysed by tissue lysis buffer (Sigma‑Aldrich, USA) with protease inhibitors (Merck, Germany). After quantification via BCA method, proteins were separated by polyacrylamide gel and then transferred to PVDF membrane. Afterward, the membrane was blocked with 10% none-fat milk at room temperature for 1 h, and then incubated with the primary antibody at 4 °C overnight. On the following day, after washing with TBST for three times, the membrane was incubated with a secondary antibody at room temperature for 1 h. Finally, protein bands were detected via ECL system (Millipore, USA). Primary antibodies including anti-HMGA1 (ab129153), anti-Col10a1 (ab58632), anti-Runx2 (ab76956), anti-Osterix (ab94744), anti-Osteocalcin (ab236048) and anti-wnt10b (ab70816) were obtained from Abcam Techonology (USA). Primary antibodies including anti-p-GSK-3β (#5558), anti-GSK-3β (#12,456), anti-β-catenin (#2009), anti-p-β-catenin (#8480) and anti-GAPDH (#5174) were obtained from Cell Signaling Technology (USA).
Statistical analysis
SPSS13.0 software was used in the research for data analysis. Every experiment was repeated three times, and data were presented as mean ± SD. ANOVA analysis with a Bonferroni test was used for comparison between three or more than three groups. A p < 0.05 was regarded as statistically significant.
Results
Overexpressing of POU2F2 promotes fracture healing and callus growth
According to the X-ray images, at the 14 d after operation, the fracture gap was still obvious in all three groups. However, larger callus volumes were seen in the LV-POU2F2 group compared to PBS group and LV-scramble group ()). At the 7 d after the operation, CW in three groups showed no significant difference, but later at the 21 d after operation, LV-POU2F2 group had a dramatically higher CW valve than the other two groups ()). Moreover, the results of radiographic score evaluated by X-ray images demonstrated that LV-POU2F2 group had higher scores than PBS group and LV-scramble group at the 14, 21 and 28 d after the operation ()).
Figure 1. X-ray images of the fracture healing.
(a) Representative X-ray images of the fractures on post-operative 14 d. (b) Quantitative analysis of the CW on post-operative 7 and 21 d. (c) Bone formation in X-ray images was assessed on postoperative 7, 14, 21 and 28 d, using a radiographic score. n = 10. Statistical significance was shown as **p < 0.01.
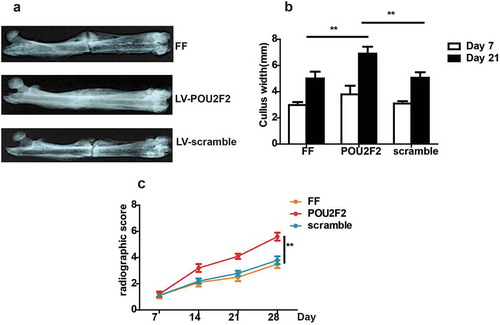
At the 14 d after the operation, bone callus was also evaluated via a micro-CT scanning, and results showed that LV-POU2F2 group had lager bone callus than the other two groups ()). Moreover, three groups had no significant differences of TV (tissue volume), but BV (bone volume) and BV/TV were higher in LV-POU2F2 group than the other two groups (,)).
Figure 2. Micro-CT images of the fracture healing.
(a) Representative Micro-CT images of the fractured femur on post-operative 14 d. (b and c) TV and BV of the callus, and BV/TV on post-operative 14 d was quantified. n = 10. Statistical significance was shown as *p < 0.05, **p < 0.01. CT, computed tomography; BV, bone volume; TV, total volume.
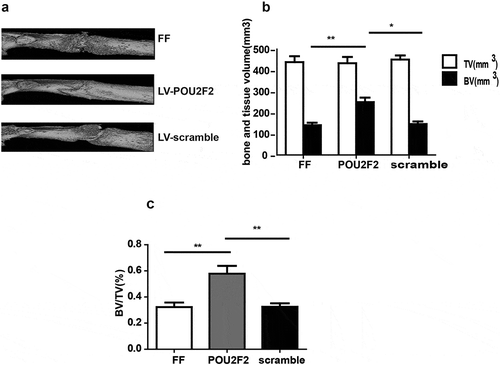
Overexpressing of POU2F2 promotes the reconstruction of fracture in rats
Next, fracture healing, as well as callus growth, were detected via Safranin-O/Fast green and HE staining (). At the 28 d after operation, as showed by Safranin-O/Fast green staining, the callus was in the reconstruction stage with various relic woven bones in both FF and LV-scramble groups. However, in the LV-POU2F2 group, the bone presented repatency and there existed fewer relic woven bones. In the meantime, as showed by HE staining, the callus was in the reconstruction stage with various relic woven bones in both FF and LV-scramble groups. However, in the LV-POU2F2 group, the bone presented repatency with more compact and regular shapes and there existed fewer relic woven bones. Results from Safranin-O/Fast green and HE staining demonstrated that overexpressing of POU2F2 promoted the reconstruction of fracture in rats.
Overexpressing of POU2F2 promotes expression of cartilage and bone-related markers
At first, we detected RNA expression of POU2F2 via RT‐qPCR in callus tissues. As showed in ), LV-POU2F2 group had increased POU2F2 expression compared to the other two groups, while the FF group and LV-scramble group showed no difference in POU2F2 expression. Next, RT-qPCR and Western blot were used to detect the expression of cartilage and bone-related markers (–)). Results demonstrated that LV-POU2F2 group had increased mRNA and protein expression of Col10a1, Runx2, Osterix, as well as Osteocalcin, compared to the other two groups (all p < 0.05). The above results draw a conclusion that overexpressing of POU2F2 accelerates fracture healing in rats.
Figure 4. Overexpressing of POU2F2 increases expression of Col10a1, Runx2, Osterix, and Osteocalcin.
(a) POU2F2 expression was higher in callus tissues of LV-POU2F2 group at mRNA (by RT-qPCR). (b) Overexpressing of POU2F2 increased mRNA expression of Col10a1, Runx2, Osterix, and Osteocalcin. (c) Protein bands of Col10a1, Runx2, Osterix, Osteocalcin and GAPDH in each group. (d) Overexpressing of POU2F2 increased protein levels of Col10a1, Runx2, Osterix, and Osteocalcin. n = 10. Statistical significance was shown as *p < 0.05, **p < 0.01.
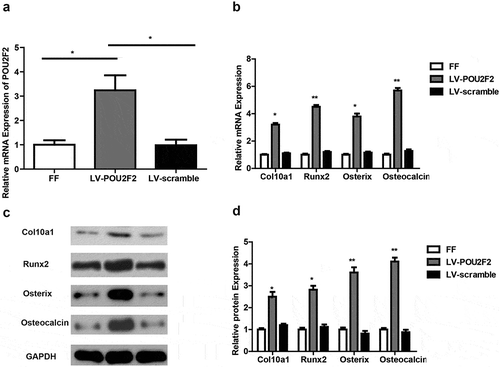
Overexpressing of POU2F2 activates the HMGA1/Wnt/β-catenin signaling pathway
According to previous reports, POU2F2 regulates the expression of HMGA1 [Citation8]. Moreover, HMGA1 can amplify the Wnt/β-catenin signaling pathway [Citation18]. In order to explore the related mechanism how POU2F2 can modulate fracture healing, we examined whether up-regulating POU2F2 can affect the expression of HMGA1 and the Wnt/β-catenin signaling pathway via western-blot (,)). Results showed that up-regulating POU2F2 increased HMGA1 expression and activated the Wnt/β-catenin signaling pathway, as showed by increased protein expression of Wnt10b and p-β-catenin/β-catenin but decreased p-GSK-3β/GSK-3β (all p < 0.05). However, no obvious difference was seen in FF group and LV-POU2F2 group.
Figure 5. Overexpressing of POU2F2 activates the HMGA1/Wnt/β-catenin signaling pathway.
(a) Protein bands of HMGA1, Wnt10b, p-β-catenin, β-catenin, p-GSK-3β, GSK-3β and GAPDH in each group. (b) Overexpressing of POU2F2 increased protein expression of HMGA1, Wnt10b, p-β-catenin, and p-GSK-3β. n = 10. Statistical significance was shown as *p < 0.05, **p < 0.01.
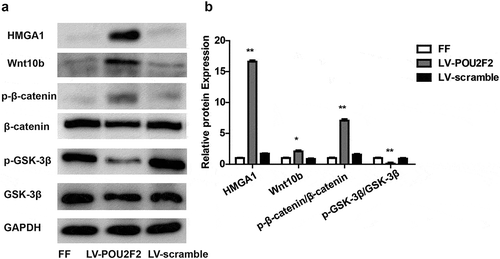
Overexpressing of POU2F2 activates the HMGA1/Wnt/β-catenin signaling pathway in vitro
Next, in vitro experiments were performed to re-prove the above result. hMSCs cell was cultured and transfected with lentivirus overexpressed POU2F2 or scramble lentivirus, then Western blot was performed to detect the HMGA1/Wnt/β-catenin signaling pathway. Results demonstrate that overexpression of POU2F2 also increased HMGA1 expression and activated the Wnt/β-catenin signaling pathway in vitro (,)).
Figure 6. Overexpressing of POU2F2 activates the HMGA1/Wnt/β-catenin signaling pathway in hMSCs cells.
(a) Protein bands of HMGA1, Wnt10b, p-β-catenin, β-catenin, p-GSK-3β, GSK-3β and GAPDH in each group. (b) Overexpressing of POU2F2 increased protein expression of HMGA1, Wnt10b, p-β-catenin, and p-GSK-3β. Statistical significance was shown as *p < 0.05, **p < 0.01.
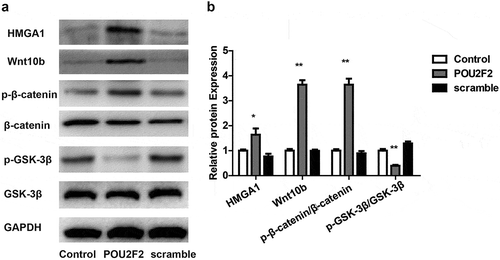
Discussion
Fracture healing is a complicated and well-organized process involving a variety of biological mechanisms. Many factors influence fracture healing including blood supply of the fracture ends, numbers of bone cells, activities of immune cells, effects of cytokines, and so on [Citation19]. Currently, many experts focus on the research of signaling pathways and cytokines for regulating fracture healing [Citation20]. Although enough research has been made in morphology of fracture healing, until now the molecular mechanism of fracture healing is still not clear.
In the past, it was reported that POU2F2 was only found in B cells as well as B-cell-derived tumor cells [Citation21–Citation23]. Recently, POU2F2 has also been reported to promote metastasis of none-B-cell- derived tumor. In gastric cancer, patients with positive POU2F2 had decreased overall survival. POU2F2 promoted gastric cancer metastasis by a positive regulation of ROBO1 [Citation24]. In the first place, it was reported that the expression of POU2F2 was decreased in nonunion fracture bone. Then, in the current study, results demonstrated that overexpressing of POU2F2 accelerates bone repatency as well as callus reconstruction, leading to a conclusion that overexpressing of POU2F2 accelerates fracture healing. Meanwhile, data in this research demonstrated that overexpressing of POU2F2 up-regulated mRNA and protein expressions of Col10a1, Runx2, Osterix as well as Osteocalcin. Runx2 can regulate chondrocyte hypertrophy [Citation25] via targeting and regulating the expression of genes like Col10a1 to achieve successive chondrocyte maturation [Citation26]. Moreover, it is reported that Runx2 and Osterix can affect the osteogenic differentiation of mesenchymal pluripotent cells as well as the expression of many bone marker genes [Citation27]. It has been previously shown that LncRNA KCNQ1OT1 may play a key role in osteoblastic differentiation via increasing the expressions of Runx2, Osterix, and Osteocalcin [Citation28]. The above reports indicate that high expressions of Col10a1, Runx2, Osterix as well as Osteocalcin are associated with bone formation and fracture healing. As a result, we conclude that overexpressing of POU2F2 plays a key role in accelerating fracture healing via up-regulating Col10a1, Runx2, Osterix as well as Osteocalcin.
The HMGA (High Mobility Group A) protein family belongs to the same High Mobility Group superfamily of HMGN proteins [Citation29]. Despite distinct families differing in functions and structural domains, all HMG proteins lack any obvious specificity for DNA consensus sequences and compete with different mechanisms with histone H1 for DNA binding, thus negatively influencing the formation of higher-order chromatin structures [Citation30]. HMGA proteins have been demonstrated to be key actors in the process of epithelial-to-mesenchymal transition (EMT), cell motility, and invasion [Citation31–Citation34]. As POU2F2 has been reported to regulate the expression of HMGA1 [Citation8], we detect the expression of HMGA1 in callus of fracture bones. Results showed overexpressing of POU2F2 promoted the expression of HMGA1, suggesting that POU2F2 may regulate fracture healing via targeting HMGA1.
During the process of skeletal growth and fracture healing, various signaling pathways participate in chondrocyte differentiation, thus tightly regulating the pace of cartilage and bone formation. For example, Ihh [Citation35], Pth [Citation36], Bmp [Citation37], and Wnt/β-catenin [Citation38] signaling pathways all have influences on the processes of cartilage and bone formation. According to our research, POU2F2 regulated HMGA1 expression in fracture bones, and HMGA1 can amplify the Wnt/β-catenin signaling pathway [Citation18], so we next detected the status of Wnt/β-catenin pathway in fracture bones. Western-blot assay demonstrated that overexpressing of POU2F2 increased protein levels of Wnt10b, increased the level of p-β-catenin/β-catenin but decreased the level of p-GSK-3β/GSK-3β. Meanwhile, in-vitro study showed that overexpression of POU2F2 increased HMGA1 expression and activated the Wnt/β-catenin signaling pathway in hMSCs cells. According to previous research, Wnt/β-catenin axis plays an important part in osteogenic differentiation, and ablation of β-catenin in osteoblasts inhibits osteoblasts differentiation [Citation39,Citation40]. Together, the above reports conformed to the current study, namely, POU2F2 accelerates fracture healing via activating the Wnt/β-catenin axis.
Above all, these data supports that overexpressing of POU2F2 accelerates fracture healing by activating the HMGA1/Wnt/β-catenin signaling pathway. Our research has provided a theoretical foundation for regulating POU2F2 to accelerate fracture healing, and offered novel thoughts and methods for fracture treatment.
Author contribution
The manuscript was written through contributions of all authors. All authors have given approval to the final version of the manuscript.
Availability of data and materials
All data generated or analyzed during the present study are included in this published article.
Disclosure statement
No potential conflict of interest was reported by the authors.
References
- Einhorn TA, Gerstenfeld LC. Fracture healing: mechanisms and interventions. Nat Rev Rheumatol. 2015;11:45–54.
- O’Keefe RJ. Fibrinolysis as a target to enhance fracture healing. N Engl J Med. 2015;373:1776–1778.
- Chandra A, Lan S, Zhu J, et al. Epidermal growth factor receptor (EGFR) signaling promotes proliferation and survival in osteoprogenitors by increasing early growth response 2 (EGR2) expression. J Biol Chem. 2013;288:20488–20498.
- Berasain C, Avila MA. Amphiregulin. Semin Cell Dev Biol. 2014;28:31–41.
- Griffin XL, Costa ML, Parsons N, et al. (2011) Electromagnetic field stimulation for treating delayed union or non-union of long bone fractures in adults, The Cochrane database of systematic reviews, CD008471.
- Hatzopoulos AK, Stoykova AS, Erselius JR, et al. Structure and expression of the mouse Oct2a and Oct2b, two differentially spliced products of the same gene. Development. 1990;109:349–362.
- Tang H, Sharp PA. Transcriptional regulation of the murine 3ʹ IgH enhancer by OCT-2. Immunity. 1999;11:517–526.
- Chiefari E, Arcidiacono B, Possidente K, et al. Transcriptional regulation of the HMGA1 gene by octamer-binding proteins Oct- 1and Oct-2. PloS One. 2013;8:e83969.
- Kang SM, Tsang W, Doll S, et al. Induction of the POU domain transcription factor Oct- 2during T-cell activation by cognate antigen. Mol Cell Biol. 1992;12:3149–3154.
- Zhang J. Bioinformatics analysis of novel transcription factors and related differentially regulated modules in non-union skeletal fractures. J Back Musculoskelet Rehabil. 2018;31:623–628.
- Chiappetta G, Avantaggiato V, Visconti R, et al. High level expression of the HMGI (Y) gene during embryonic development. Oncogene. 1996;13:2439–2446.
- Fedele M, Fusco A. Role of the high mobility group A proteins in the regulation of pituitary cell cycle. J Mol Endocrinol. 2010;44:309–318.
- Schuldenfrei A, Belton A, Kowalski J, et al. HMGA1 drives stem cell, inflammatory pathway, and cell cycle progression genes during lymphoid tumorigenesis. BMC Genomics. 2011;12:549.
- Giannini G, Kim CJ, Di Marcotullio L, et al. Expression of the HMGI(Y) gene products in human neuroblastic tumours correlates with differentiation status. Br J Cancer. 2000;83:1503–1509.
- Sgarra R, Diana F, Rustighi A, et al. Increase of HMGA1a protein methylation is a distinctive characteristic of leukaemic cells induced to undergo apoptosis. Cell Death Differ. 2003;10:386–389.
- Ha TK, Her NG, Lee MG, et al. Caveolin-1 increases aerobic glycolysis in colorectal cancers by stimulating HMGA1-mediated GLUT3 transcription. Cancer Res. 2012;72:4097–4109.
- Murata K, Ito H, Yoshitomi H, et al. Inhibition of miR-92a enhances fracture healing via promoting angiogenesis in a model of stabilized fracture in young mice. J Bone Miner Res. 2014;29:316–326.
- Xian L, Georgess D, Huso T, et al. HMGA1 amplifies Wnt signalling and expands the intestinal stem cell compartment and Paneth cell niche. Nat Commun. 2017;8:15008.
- Nelson FR, Brighton CT, Ryaby J, et al. Use of physical forces in bone healing. J Am Acad Orthop Surg. 2003;11:344–354.
- Crockett JC, Rogers MJ, Coxon FP, et al. Bone remodelling at a glance. J Cell Sci. 2011;124:991–998.
- Saito M, Tanaka S, Mori A, et al. Primary gastric Hodgkin’s lymphoma expressing a B-Cell profile including Oct- 2and Bob-1 proteins. Int J Hematol. 2007;85:421–425.
- Robinson AR, Kwek SS, Kenney SC. The B-cell specific transcription factor, Oct-2, promotes Epstein-Barr virus latency by inhibiting the viral immediate-early protein, BZLF1. PLoS Pathog. 2012;8:e1002516.
- Garcia-Cosio M, Santon A, Martin P, et al. Analysis of transcription factor OCT.1, OCT.2 and BOB.1 expression using tissue arrays in classical Hodgkin’s lymphoma. Mod Pathol. 2004;17:1531–1538.
- Wang SM, Tie J, Wang WL, et al. POU2F2-oriented network promotes human gastric cancer metastasis. Gut. 2016;65:1427–1438.
- Takeda S, Bonnamy JP, Owen MJ, et al. Continuous expression of Cbfa1 in nonhypertrophic chondrocytes uncovers its ability to induce hypertrophic chondrocyte differentiation and partially rescues Cbfa1-deficient mice. Genes Dev. 2001;15:467–481.
- Zheng Q, Zhou G, Morello R, et al. Type X collagen gene regulation by Runx2 contributes directly to its hypertrophic chondrocyte-specific expression in vivo. J Cell Biol. 2003;162:833–842.
- Lee CH, Huang YL, Liao JF, et al. Ugonin K promotes osteoblastic differentiation and mineralization by activation of p38 MAPK- and ERK-mediated expression of Runx2 and osterix. Eur J Pharmacol. 2011;668:383–389.
- Gao X, Ge J, Li W, et al. LncRNA KCNQ1OT1 promotes osteogenic differentiation to relieve osteolysis via Wnt/beta-catenin activation. Cell Biosci. 2018;8:19.
- Bustin M. Revised nomenclature for high mobility group (HMG) chromosomal proteins. Trends Biochem Sci. 2001;26:152–153.
- Catez F, Yang H, Tracey KJ, et al. Network of dynamic interactions between histone H1 and high-mobility-group proteins in chromatin. Mol Cell Biol. 2004;24:4321–4328.
- Pegoraro S, Ros G, Piazza S, et al. HMGA1 promotes metastatic processes in basal-like breast cancer regulating EMT and stemness. Oncotarget. 2013;4:1293–1308.
- Thuault S, Valcourt U, Petersen M, et al. Transforming growth factor-beta employs HMGA2 to elicit epithelial-mesenchymal transition. J Cell Biol. 2006;174:175–183.
- Morishita A, Zaidi MR, Mitoro A, et al. HMGA2 is a driver of tumor metastasis. Cancer Res. 2013;73:4289–4299.
- Sgarra R, Pegoraro S, Ros G, et al. High Mobility Group A (HMGA) proteins: molecular instigators of breast cancer onset and progression. Biochim Biophys Acta Rev Cancer. 2018;1869:216–229.
- Vortkamp A, Pathi S, Peretti GM, et al. Recapitulation of signals regulating embryonic bone formation during postnatal growth and in fracture repair. Mech Dev. 1998;71:65–76.
- Ellegaard M, Jørgensen NR, Schwarz P. Parathyroid hormone and bone healing. Calcif Tissue Int. 2010;87:1–13.
- Tsuji K, Bandyopadhyay A, Harfe BD, et al. BMP2 activity, although dispensable for bone formation, is required for the initiation of fracture healing. Nat Genet. 2006;38:1424–1429.
- Huang Y, Zhang X, Du K, et al. Inhibition of beta-catenin signaling in chondrocytes induces delayed fracture healing in mice. J Orthop Res. 2012;30:304–310.
- Zhang W, Xue D, Yin H, et al. Overexpression of HSPA1A enhances the osteogenic differentiation of bone marrow mesenchymal stem cells via activation of the Wnt/beta-catenin signaling pathway. Sci Rep. 2016;6:27622.
- Kubota T, Michigami T, Ozono K. Wnt signaling in bone. Clin Pediatr Endocrinol. 2010;19:49–56.