ABSTRACT
Penicillium marneffei is a thermally dimorphic fungus that causes penicilliosis, and become the third-most-common opportunistic fungal infection in immunocompromised patients in Southeast Asia. Azoles and amphotericin B have been introduced for the treatment, however, it is important to investigate possible mechanisms of azole resistance for future treatment failure. We identified 177 putative MFS transporters and classified into 17 subfamilies. Among those, members of the Drug:H+ antiporter 1 subfamily are known to confer resistance to antifungals. Out of 39 paralogs, three (encoded by PmMDR1, PmMDR2, and PmMDR3) were heterologously overexpressed in S. cerevisiae AD∆ conferred resistance to various drugs and compounds including azoles, albeit to different degrees. PmMDR1-expressing strain showed resistance to the broadest range of drugs, followed by the PmMDR3, and PmMDR2 conferred weak resistance to a limited range of drugs. We conclude that PmMDR1 and PmMDR3, may be able to serve as multidrug efflux pumps.
Graphical abstract
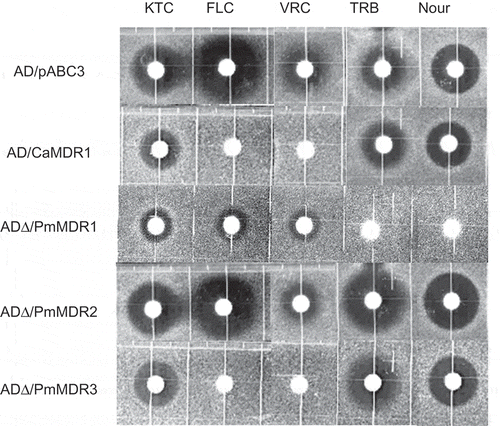
Two MSF transporters of Penicillium marneffei, PmMdr1p and PmMdr3p, showed the efflux pump functions to different antifungals.
Penicillium marneffei (teleomorph: Talaromyces marneffei) is the only thermally dimorphic fungus in the genus Penicillium [Citation1]. This fungus can switch cell shape from mycelium at 25°C to yeast form at 37°C [Citation2]. This transition is considered to be a pathogenisis factor, given that yeast cells are capable of evading the host immune system [Citation2]. The opportunistic infections caused by this species are common in immunocompromised patients with HIV infections and even in non-HIV-infected patients who have other immunocompromising conditions [Citation3,Citation4]. The resulting systemic infection, called penicilliosis, causes symptoms such as anemia and fever, which are similar to those observed in disseminated cryptococcosis [Citation5]. Penicilliosis has become endemic in tropical Asia in recent years, rating as the third-most-common opportunistic infection after tuberculosis and cryptococcosis [Citation6]. Penicilliosis causes high mortality rates in immunocompromised patients, with death rates of 20.7% and 29.4% among HIV- and non-HIV-infected patients (respectively) in Thailand [Citation5], and mortalities of 24.3% and 50.6% among patients who were treated or untreated with antifungals (respectively) in China [Citation7]. Combination therapy with amphotericin B followed by itraconazole or voriconazole is effective for penicilliosis [Citation8]; however, prolonged prophylaxis with azoles might select for the emergence of drug-resistant strains [Citation9].
Various mechanisms are involved in azole resistance. Overexpression of drug efflux pumps that catalyze efflux of a broad range of cytotoxic substrates is a major mechanism for multidrug resistance (MDR) [Citation10]. MDR pumps can transport substrates constituting a wide range of structurally and functionally unrelated compounds, including xenobiotics that are usually not present in the cell’s natural environment. By expelling these compounds, cells maintain intracellular concentrations of xenobiotics below a toxic level [Citation10]. To date, MDR transporters have been identified in three kingdoms (eukaryotes, prokaryotes, and archaea) and have been classified as belonging to either of two types of transport systems, the ATP-binding cassette (ABC) superfamily and the major facilitator superfamily (MFS). ABC transporters are usually arranged in two-times-two topology, comprising two transmembrane domains (TMDs) and two nucleotide-binding domains (NBDs) [Citation11]; examples include Candida albicans CDR1 and CDR2 [Citation12–Citation15], C. glabrata CDR1 [Citation16] and Cryptococcus neoformans AFR1 [Citation17]. In P. marneffei, 46 ABC transporters were identified in our previous work, including PmABC1, which was characterized as a multidrug efflux pump [Citation18].
MFS transporters are powered by the electrochemical proton motive force and usually consist of 12 or 14 transmembrane segments (TMSs) with intervening intracellular and extracellular loops [Citation11]. According to the transporter classification database (TCDB, www.tcdb.org, June 2019), the MFS family contains 88 subfamilies, including sugar porters (SP), drug:H+ antiporter 1 (DHA1) proteins, drug:H+ antiporter 2 (DHA2) proteins and anion-cation symporters (ACS) [Citation19]. In some fungi, the SP subfamily is functionally diverse, serving primarily as transporters of glucose, but also exhibiting the ability to transport galactose, quinate, myoinositol, lactose, maltose, and α-glucosides [Citation20].
Most of the MFS transporters implicated in MDR belong to the DHA1 subfamily, members of which are present in all kingdoms [Citation20]. Twelve DHA1 transporters from budding yeast, Saccharomyces cerevisiae, have been implicated in MDR, including ScTPO1 to ScTPO4, ScQDR1 to ScQDR3, ScAQR1, ScDTR1, ScHOL1, ScFLR1, and ScYHK8 [Citation21–Citation30]. C. albicans encodes 18 DHA1 transporters in total, but only seven of these proteins (CaMDR1, CaFLU1, CaNAG3, CaNAG4, CaQDR1, CaQDR2, and CaQDR3) confer MDR [Citation30–Citation33]. Four of the 10 DHA1 transporters encoded by C. glabrata (CgAQR1, CgTPO3, CgQDR2, CaFLR1, and CaFLR2) [Citation34–Citation37] and one of the nine transporters encoded by C. neoformans (CNA07070) also have been characterized as conferring MDR [Citation38]. In the genome DNA analyzes of other fungal species, multiple members of the DHA1 subfamily have been identified, including 28 in C. parapsilosis, 17 in C. lusitaniae, 18 in C. tropicalis, 31 in C. guilliermondii and 54 in Aspergillus fumigatus, but none of these candidates has been characterized as conferring MDR [Citation38].
Given that fungal DHA1 transporters are known to act as MDR proteins, identification and characterization of DHA1-subfamily transporters in emerging fungal species such as P. marneffei are crucial to addressing the possible need for counter-measures against antifungal resistance. P. marneffei encodes 256 putative MFS transporters, including 39 members of the DHA1 subfamily that possess 12 TMSs each. In the present study, we classified MFS transport proteins in P. marneffei. Among these members of the DHA1 subfamily, we selected for further evaluation three candidate proteins (PmMDR1, PmMDR2, and PmMDR3) that exhibited high amino acid identity to ScTPO1, ScTPO2, ScTPO3, or CgTPO3; these Saccharomyces and Candida paralogs that have been shown to possess wide substrate specificities. The open reading frames (ORFs) encoding the PmMDR1, PmMDR2 and PmMDR3 proteins were cloned and subjected to heterologous overexpression in a transporter-deficient strain of S. cerevisiae (ADΔ) [Citation39] to analyze potential contributions to MDR. Indeed, all three PmMDR proteins conferred MDR at various levels when produced in S. cerevisiae ADΔ. To our knowledge, this work represents the first report of DHA1-subfamily transporters of P. marneffei that provide resistance to a wide range of drugs, including azoles, polyene and antimalarial.
Materials and methods
Strains and culture conditions
The fungal strains used in this study are listed in . S. cerevisiae AD∆ was grown in yeast extract peptone dextrose medium (YPD) containing 1% w/v yeast extract (Nacalai Tesque, Kyoto, Japan), 2% w/v BactoTM peptone (Difco Laboratories, MI, USA), and 2% w/v glucose with or without 2% w/v agar at 30°C. AD∆ uracil prototrophic transformants were selected for URA+ on yeast complete supplement medium without uracil (CSM-URA) agar containing 2% w/v glucose, 0.67% w/v yeast nitrogen base (YNB) without amino acids (Difco), 0.077% CSM (Funakoshi, Tokyo, Japan), and 2% w/v agar. For the liquid micro-dilution assay, the pH of CSM was adjusted by adding 10 mM MES (Dojindo, Tokyo, Japan) and 20 mM HEPES (Nacalai tesque). Escherichia coli DH5α (Agilent Technologies, CA, USA) was grown in Luria-Bertani Broth (LB) containing 1% w/v tryptone (Nacalai Tesque), 0.5% w/v yeast extract (Nacalai Tesque), and 0.5% w/v NaCl (Nacalai Tesque). E. coli transformants were selected on LB agar (2% w/v) supplemented with 150 μg/mL ampicillin for selection of plasmids.
Table 1. Yeast strains used in this study.
Drugs and compounds
The antifungals and xenobiotics used in this study were purchased from the following sources: fluconazole (FLC), ketoconazole (KTC), and terbinafine (TRB), from LKT Laboratories, Inc. (St. Paul, MN, USA); itraconazole (ITC), miconazole (MCZ), rhodamine 6 G (R6G), latrunculin A (LAT A), cerulenin (CER), cytochalasin D (Cyt D), nigericin (NIG), quinidine (Quin), mecophenolic acid (MPA), and amphotericin B (AMB) from Wako (Osaka, Japan); nystatin (Nys), rhodamine 123 (R123), spermine (SPM), spermidine (SPD), and putrescine (PUT) from Sigma-Aldrich, (St. Louis, MO, USA); cycloheximide (CHX) and geneticin (G418) from Nacalai Tesque; voriconazole (VRC) from Tocris Bioscience (Bristol, United Kingdom); 5-flucytosine (5-FC) from Tokyo Chemical Industry Co., Ltd. (Tokyo, Japan); nourceothricin (Nour) from Jena Bioscience GmbH (Jena, Germany); and posaconazole (POS) from Tokyo Chemical Industry Co., Ltd. Micafungin (MFG) was kindly provided by Astelas Pharma, Inc. (Tokyo, Japan).
Identification of MFS transporter homologs
All putative transporters of the MFS superfamily encoded by P. marneffei strain 18224 were recovered from NCBI GenBank (www.ncbi.nlm.nih.gov, October 2016) and their amino acid sequences were classified into subfamilies using BLASTP from TCDB [Citation19]. Similarity of DHA1-subfamily proteins of P. marneffei to S. cerevisiae TPO1, TPO2 and TPO3 was determined using the NCBI BLASTP program (www.blast.ncbi.nlm.nih.gov/Blast.cgi); proteins with the highest identity to the S. cerevisiae proteins were selected for further analysis [Citation40].
The amino acid sequences of the previously characterized DHA1 transporters from S. cerevisiae, C. albicans, C. glabrata and C. neoformans, along with those of the three selected P. marneffei proteins, were aligned using clustal omega (www.ebi.ac.uk) [Citation41]. These sequence data have been submitted to the GenBank databases under accession number MN396869, MN396870, and MN396871, for PmMDR1, PmMDR2, and PmMDR3, respectively.
Isolation of total RNA from P. marneffei
Total RNA was extracted from yeast-form cells of P. marneffei strain 60996 [Citation42]. Cells were grown in 200 mL of YPD in the absence and presence of 0.001 or 0.002 μg/mL ITC at 37°C for 72 h, harvested by filtering the cell suspension using an aspirator (As One, Tokyo, Japan), and disrupted in the presence of liquid nitrogen using a Process Homogenizer PH91 (SMT Company, Tokyo, Japan). Freeze-dried disrupted samples were resuspended in 8 mL TES buffer (1% SDS, 5 mM EDTA, and 10 mM Tris-HCl pH 7.4) and 8 mL acid phenol:chloroform:isoamyl alcohol (25:24:1). The cell suspension was incubated at 65°C for 1 h, vortexing vigorously for 10 sec at 15-min interval, and cooled on ice for 5 min. The extracted cells were subjected to centrifugation at 25,000 x g for 5 min at 4°C. RNA was precipitated from the resulting supernatant by adding 1 mL sodium acetate and 4 mL 99.5% ethanol, and the mixture was subjected to centrifugation at 25,000 x g for 10 min at 4°C. The resulting pellet (total RNA) was stored at – 80°C until further use.
Quantification of PmMDR1, PmMDR2, and PmMDR3 mRNA by real-time reverse transcription PCR (RT-PCR)
The total RNA containing the entire mRNA population from P. marneffei strain 60996 was used as the RNA template to synthesize first-strand cDNA copies using the reverTra ace® qPCR RT master mix with gDNA remover (Toyobo, Osaka, Japan). The qRT-PCR was performed using a StepOne RT PCR system (Applied Biosystems, Foster City, CA, USA). Reactions were performed as 15-µL volumes of mixtures containing THUNDERBIRD SYBR qPCR mix (Toyobo) with primer pairs PMAA_034610_F1 and PMAA_034610_R for PmMDR1, PMAA_080940_F and PMAA_080940_R for PmMDR2, and PMAA_067100.1231F and PMAA_067100_Rnew for PmMDR3 (Table S1).
Construction of S. cerevisiae AD∆ strains overexpressing PmMDR1, PmMDR2, and PmMDR3
The high-fidelity KOD polymerase (Toyobo) or Q5 polymerase (New England Biolabs, MA, USA) was used for polymerase chain reaction (PCR) amplification. Although S. cerevisiae AD∆ has been shown to recognize and process C. krusei introns [Citation43], the same is not the case for P. marneffei introns [Citation18]. P. marneffei cDNA therefore was used to clone PmMDR1 and PmMDR2, whereas genomic DNA (gDNA) was used for the cloning of PmMDR3, which has no introns.
The PmMDR1 ORF (1.6 kb) was PCR amplified from cDNA using a primer pair consisting of PMAA_34610_FP.pdr5 and PMAA_34610_RP.pgk1. These primers were designed to amplify the PmMDR1 ORF flanked by upstream end of the PDR5 promotor and beginning of PGK1 terminator (; Table S1). However, no E. coli transformants were obtained when attempting to clone this amplicon, presumably indicating toxicity of a gene product encoded by this amplicon. Therefore, a transformation cassette was prepared by PCR amplification, a DNA fragment containing the PDR5 promoter region (1.1 kb) was amplified using primers Asc1_for and PDR5up_RP.034610. A second fragment containing the downstream end of the PDR5 gene, the PGK1 terminator and the URA3 marker (1.7 kb) was amplified using the primers PGK1_FP_034610 and Asc1_rev. These three fragments were purified and used directly for transformation of S. cerevisieae AD∆ using the LiAc method ().
Figure 1. Cloning strategy for PmMDR1, PmMDR2 and PmMDR3 in Saccharomyces cerevisiae ADΔ using the yeast membrane protein hyperexpression system.The transformation cassette consists of a 1.1-kb DNA fragment (upstream region of PDR5), open reading frame (ORF) to be cloned, and a 1.7-kb DNA fragment (PGK1 terminator, URA3 marker and downstream end of the PDR5 ORF (ds)). The PmMDR1, PmMDR2, and PmMDR3 ORFs were PCR amplified using primer sets as shown in the figure and Supplementary Table S1, employing either cDNA (PmMDR1 and PmMDR2) or genomic DNA (PmMDR3) as templates. For cloning of PmMDR2 and PmMDR3 the amplified ORF was inserted in plasmid pABC3 between the PacI and NotI sites and then amplified in E. coli DH5α; the transformation cassette then was excised from the newly constructed plasmid by AscI digestion. For cloning of PmMDR1, the ORF was directly used for transformation with the 1.1-kb and 1.7-kb DNA fragments, which were PCR amplified using pABC3 as template and primer pairs of AscI_for and primer C (PDR5up_RP.034610) and AscI_rev and primer D (PGK1_FP_034610) (Lamping et al., 2007). For each of the 3 PmMDR genes, the resulting transformation cassette was integrated into the S. cerevisiae AD∆ chromosome at the PDR5 locus by homologous recombination. Primers used for the individual genes were as follows (A and B, respectively): for PmMDR1, PMAA_034610_F1 and PMAA_034610_R; for PmMDR2, PMAA_080940-FP and PMAA_080940-RP; for PmMDR3, Fwd-067100-MFS 0984413–1 and Rev-067100-MFS 0984287–1.
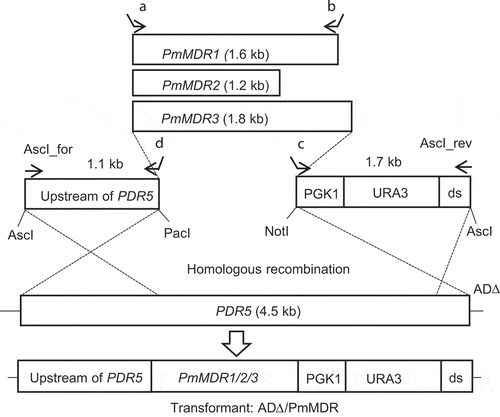
The PmMDR2 ORF (1.2 kb) was PCR amplified from cDNA with the primers PMAA_080940-FP and PMAA_080940-RP, while the PmMDR3 ORF (1.8 kb) was PCR amplified from gDNA with the primers Fwd-067100-MFS0984413-1 and Rev-067100-MFS 0984287–1. These primer pairs contained essential elements for cloning purposes, as described previously [Citation43] (; Table S1). The resulting PmMDR2 and PmMDR3 ORFs were inserted (separately) into the pABC3 plasmid at PacI and NotI sites [Citation39]. Transformation cassettes were obtained from plasmids pABC3::PmMDR2 and pABC3::PmMDR3 by AscI (New England Biolab) digestion; the resulting fragment were used (separately) to transform AD∆ using the Li acetate method (). In each case, the intention was that the MDR transformation cassette was integrated into the chromosome of AD∆ at the ScPDR5 locus by homologous recombination. Uracil prototrophic colonies were selected on CSM-URA agar plates and recovered after 3 days of incubation at 30°C. Transformants with the expected gene structures were identified by screening via DNA sequencing and colony PCR, which confirmed integration at the expected sites upstream and downstream of the PDR5 locus.
Yeast strains constitutively overexpressing PmMDR1, PmMDR2 and PmMDR3 were designated as ADΔ/PmMDR1, ADΔ/PmMDR2 and ADΔ/PmMDR3, respectively.
Agarose diffusion assay
Cells were grown in CSM at 30°C for 16 h and diluted into 1 mL fresh CSM to yield an optical density (at 600 nm; OD600nm) of 0.35. A portion of this cell suspension (800 µL) was added to 20 mL of melted CSM agarose (0.6% w/v) at 48°C, and the mixture then was poured immediately on top of CSM agarose base medium (20 mL) in a rectangular plate. Sterile Whatman paper discs (6 mm in diameter; Advantage, Toyo Roshi Kaisha, Ltd., Japan) containing xenobiotics were placed on the CSM agarose overlay. Plates were incubated at 30°C for 48 h.
Liquid micro-dilution assay
Minimum growth inhibitory concentrations (MICs) of test drugs for AD∆/PmMDR1, AD∆/PmMDR2 and AD∆/PmMDR3 were determined using the liquid micro-dilution method in a 96-well microtiter plate format, as described in the Clinical Laboratory Standards Institute (CLSI) method, with some modifications. In brief, cells were grown in CSM (pH 7) for 16 h, diluted in fresh CSM to an OD600nm of 0.35, and grown until the OD600nm reached ~ 1. Cells from the resulting cultures were inoculated at 5 × 104 per well in a flat-bottom 96-well plate (Iwaki Asahi Techno Glass, Tokyo, Japan) in the presence of a series of two-fold-diluted test compounds in a final volume of 200 µL/well. Plates were incubated at 30°C without shaking for 48 h. Cell growth was assessed by monitoring the OD600nm in the wells using a Varioskan Lux microplate reader (Thermo Fisher Scientific, Lombard, IL, USA). MICs were defined as 90% inhibition of growth of a given strain.
For the polyamine susceptibility assays, cells were grown in two different minimal media, Mg2+-limited CSD (completely synthetic medium) supplemented with 0.05 mM MgSO4, and in basal medium (BM) containing (per one liter) 1.7 g of YNB without amino acids or NH4+ (Difco), 20 g of glucose and 2.65 g of (NH4)2SO4 (Nacalai Tesque) as described previously [Citation21,Citation22,Citation35,Citation44] with CSM-URA media as control positive.
Results
Classification of P. marneffei MFS transporters
The MFS transporter superfamily is classified into 88 subfamilies in living organisms. In this study, according to the genome sequence of P. marneffei, members of 17 of the MFS subfamilies are encoded by this organism; of those 17 groups, five and twelve -were considered major and minor MFS subfamilies, respectively, representing a total of 346 proteins with 256 putative (known function) and 90 hypothetical (unknown function) proteins predicted to belong to the MFS superfamily. Of the 256 putative proteins, 177 were predicted to contain the 12 or 14 TMSs seen in MFS transporters typically.
The five major MFS subfamilies included sugar porters (SP), drug:H+ antiporter 1 (DHA1) proteins, drug:H+ antiporter 2 (DHA2) proteins, monocarboxylate transporters (MCT) and anion cation symporters (ACS). Forty-five SP proteins were contained in P. marneffei and constituted the largest subfamily of MFS transporters in this fungus. The second largest subfamily in P. marneffei was the DHA1 group (39 proteins) and the ACS subfamily was the third-largest subfamily in P. marneffei, containing 34 proteins. Other major subfamilies in P. marneffei were the MCTs (containing 20 proteins) and the DHA2s (containing 16 proteins). P. marneffei paralogs of the DHA1 and DHA2 proteins contained 12 and 14 TMSs, respectively, and proteins of both subfamilies are conserved in all three kingdoms (; Table S2).
Table 2. Classification of typical P. marneffei MFS transporters with 12 and 14 transmembrane spans (TMSs).
Twelve minor subfamilies also were represented, including fucose symporters (FHS), phosphate:H+ symporters (PHS), oxalate:formate antiporters (OFA), siderophore-iron transporters (SIT), two types of vacuolar basic amino acid transporters (VBAAT and V-BAAT), peptide/acetyl-coenzyme A/drug transporters (PAT), feline leukimia virus C receptors (FLVCR), L-amino acid transporter-3 (LAT-3) proteins, N-acetylglucosamine transporters (NAG-T), and unidentified major-facilitator-12 (UMF12) and −23 (UMF23) proteins (; Table S2).
In addition, there were some atypical putative transporters in MFS superfamily predicted by TCDB (shown in Table S3).
Selection of MDR protein candidates of P. marneffei
According to the TCDB, 39 DHA1 transporters are predicted to be paralogs of proteins found in some eukaryotic organisms such as fungi (S. cerevisiae, Acremonium chrysogenum, Schizosaccharomyces pombe, Penicillium chrysogenum, Cercospora nicotianae, Metarhizium robertsii), plants (Arabidopsis thaliana), and animals (Homo sapiens) (Table S2).
In the MFS superfamily, many transporters of the DHA1 subfamily are known to confer drug resistance on microorganisms [Citation45]. In the present study, the amino acid sequences of P. marneffei DHA1 proteins were compared to those of the S. cerevisiae TPO proteins, budding yeast transporters known to have broad ranges of substrate specificities. The predicted products of the P. marneffei PMAA_034610, PMAA_080940, and PMAA_067100 genes showed the strongest similarities to ScTPO1 (41% amino acid identity), ScTPO2 (38%), and ScTPO3 (37%), respectively. Therefore, these P. marneffei proteins were selected as candidate proteins for further study and were designated PmMDR1, PmMDR2, and PmMDR3, respectively (). In support of this assignment, all characterized DHA1-subfamily proteins from S. cerevisiae, C. neoformans, C. albicans, and C. glabrata were evaluated for their sequence similarity with these P. marneffei proteins. The resulting phylogenetic tree analysis showed that three PmMDR proteins are classified in cluster 2; PmMDR1 was most-closely related to ScTPO1 and CaFLU1, while PmMDR2 and PmMDR3 were most-closely related to ScTPO2, ScTPO3, and CgTPO3 (). Examination of the sequences predicted that the PmMDR1 gene contains two introns and encodes a protein of 560 amino acids with a predicted molecular mass 62 kDa. The PmMDR2 gene was thought to contain three introns and encodes a protein of 400 amino acids with a predicted molecular mass 44.5 kDa. The PmMDR3 gene was predicted to contain no introns and encodes a protein of 617 amino acids with a predicted molecular mass 69 kDa.
Figure 2. The phylogenetic relationship of all characterized DHA1 transporters in S. cerevisiae, C. albicans, C. glabrata, C. neoformans, and P. marneffei.The proteins characterized in the present work (PmMDR1, PmMDR2, and PmMDR3) are indicated in boldfaced type.
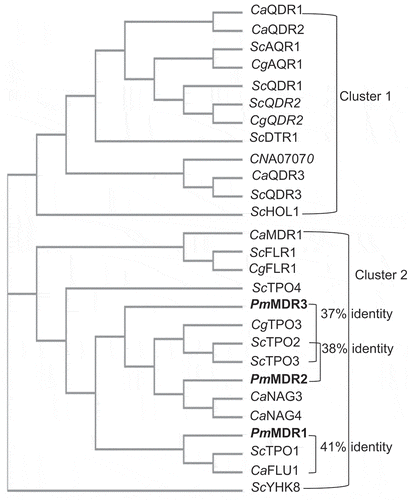
These proteins contain unique motifs found in the S. cerevisiae polyamine transporters ScTPO1, ScTPO2 and ScTPO3. Motif A (EkPLxxRxGkPxI), specific to MFS proteins, is located between TMS 2 and TMS 3, membrane spans that are important for transport activity [Citation20,Citation46]. Motif C, which is located in TMS 5 (GxxxGPxxGPxxG), is specific to DHA transporters and has been shown to be essential for drug transport, linking proton translocation to the antiport [Citation20,Citation46]. Three glutamate or aspartate residues also are located between TMS 2 and TMS 3, and in TMS 6 and TMS 12 (). In the paralogous proteins in other organisms, these residues have been shown to play an important role in interactions with polyamines [Citation22].
Figure 3. Predicted topology of fungal DHA1-subfamily transporters, including PmMDR1, PmMDR2, and PmMDR3, showing major topological features and motifs.Glutamate (e) and aspartate (d) in black boxes are important residues for interactions with polyamines [Citation22].
![Figure 3. Predicted topology of fungal DHA1-subfamily transporters, including PmMDR1, PmMDR2, and PmMDR3, showing major topological features and motifs.Glutamate (e) and aspartate (d) in black boxes are important residues for interactions with polyamines [Citation22].](/cms/asset/e1912e83-b727-4906-a3e8-28b3e83267eb/tbbb_a_1732185_f0003_b.gif)
Gene expression profiles of P. marneffei PmMDR1, PmMDR2, and PmMDR3
Gene expressions of drug efflux pumps in innate organisms are well known to be induced by addition of the drug. Then, in order to investigate expression level of three PmMDR genes in innate P. marneffei, accumulation of their transcripts in P. marneffei was measured under the absence and the presence of ITC conditions and then analyzed comparatively. In the presence of ITC, the PmMDR1 transcript accumulated to higher levels, with the mRNA levels exhibiting an apparently dose-dependent increase ()). Expression of PmMDR2 and PmMDR3 also increased in the presence of 0.001 μg/mL ITC, however, these inductions were reduced in the presence of 0.002 μg/mL ITC (–c)). These findings suggested that transcripts of all three PmMDR genes were induced in P. marneffei cells when cultured in the medium containing ITC (0.001 μg/mL).
Phenotypes of the ADΔ/PmMDR1, ADΔ/PmMDR2 and ADΔ/PmMDR3 strains
The susceptibilities of ADΔ/PmMDR1, ADΔ/PmMDR2 and ADΔ/PmMDR3 to various drugs or compounds were determined using an agarose diffusion assay and a liquid micro-dilution assay. All three MFS proteins contributed to multidrug resistance in P. marneffei, but to different degrees (PmMDR1 > PmMDR3 > PmMDR2).
Among the three strains, ADΔ/PmMDR1 showed the highest resistance to most of these drugs and compounds, exhibiting (compared to ADΔ/pABC3) 4-fold higher MIC of Nys; 8-fold higher MICs of MFG, TRB, Nour and POS; more than 16-fold higher MICs of FLC, KTC, VRC, LAT A, 5-FC, CHX, and G418; and approximately 1000-fold higher MIC of Quin (). Results of the agarose diffusion assay showed that the ADΔ/PmMDR1 strain had the highest MICs of Nour and TRB in all of these transformants, which was characteristic phenomenon of this strain ().
Table 3. Drug susceptibilities of S. cerevisiae AD∆ overexpressing P. marneffei PmMDR1, PmMDR2, and PmMDR3, and C. albicans CaMDR1.
Figure 5. Susceptibility of S. cerevisiae ADΔ/pABC3, ADΔ/CaMDR1, AD∆/PmMDR1, AD∆/PmMDR2, and AD∆/PmMDR3 to xenobiotics.Amounts (μg) loaded on discs: KTC, ketoconazole (0.04); FLC, fluconazole (3); VRC, voriconazole (0.025); TRB, terbinafine (100); Nour, nourseothricin (50).
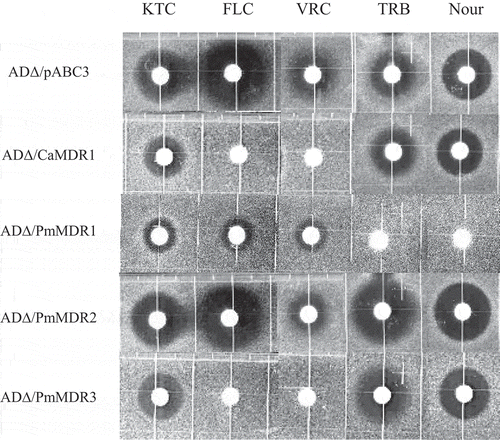
The overexpression of PmMDR2 conferred modest resistance to KTC, POS, R123, 5-FC, G418, Quin, and MPA, with MICs elevated 10-fold or less compared to the control strain (). There was no difference in susceptibility between ADΔ/PmMDR2 and ADΔ/pABC3 when assessed using the agarose diffusion assay ().
The ADΔ/PmMDR3 strain exhibited (compared to ADΔ/pABC3) 4-fold higher MICs of CER, R123 and Quin; 8-fold higher MICs of CHX, 5-FC, POS, MFG, G418, MPA, MCZ, and FLC; and 200-fold MIC of VRC (). Agarose diffusion assay also showed increased resistance to VRC and FLC compared to the control strain ().
Susceptibility of ADΔ/PmMDR1, ADΔ/PmMDR2 and ADΔ/PmMDR3 strains to polyamines
Given that ScTPO1 to ScTPO4 confer resistance to polyamines in S. cerevisiae, the ADΔ/PmMDR1, ADΔ/PmMDR2 and ADΔ/PmMDR3 strains were tested for susceptibility to SPD, SPM, and PUT. However, given that the ADΔ/PmMDR strains cannot grow in either CSD or BM medium, no MIC results could not be obtained by the standard assay (data not shown). Instead, CSM medium at pH 7 was used to check polyamine susceptibility. The results showed that the ADΔ/PmMDR strains remained susceptible to SPM, SPD and PUT ().
Discussion
MDR has become a challenging issue in pathogenic fungi due to the emergence of antifungal resistance and treatment failure in immunocompromised patients. MDR transporters, particularly those belonging to the ABC superfamily, have been identified and studied in various organisms.
In contrast, MFS transporters involved in MDR remain more-poorly characterized. Among pathogenic fungi that inhabit various environmental niches, C. neoformans has 33 ABC proteins [Citation47] and 150 MFS proteins [Citation48], and Aspergillus spp. has 50 ABC transporters [Citation47] and 275 MFS transporters [Citation49]. In contrast, C. albicans, which is associated with mammalian hosts, possesses a smaller number of ABC transporters (26 proteins) [Citation47] and MFS transporters (85 proteins) [Citation49], possibly due to its exposure to a narrower range of xenobiotics. We found that P. marneffei has 46 ABC transporters [Citation18] and 177 MFS transporters. The TCDB excluded PmMDR3 from the DHA1 subfamily [Citation19]; however, this gene product was found to be a functional drug efflux pump in our work, and so was included in the DHA1 subfamily in the present study.
The expression level of genes encoding DHA transporters usually are low in the native host, with transcriptional activation occurring under stressed conditions. Indeed, the PmMDR1, PmMDR2, and PmMDR3 transcripts accumulated to higher levels in the presence of ITC, suggesting that the PmMDR proteins may contribute to protecting cells from toxic substrates, such as azoles. Among the three PmMDR genes characterized in this present study, the PmMDR2 transcript accumulated to the highest level, despite showing the weakest drug-resistance phenotype of the three genes when assessed by heterologous expression in budding yeast. We postulate that the PmMDR2 protein might recognize fewer substrates than the other two PmMDR transporters, as was reported for ScTPO2 compared to other S. cerevisiae transporters [Citation22].
S. cerevisiae ADΔ is a suitable host for the expression and functional characterization of membrane proteins, including transporters [Citation15,Citation50,Citation51]. Overexpression of efflux pumps renders AD∆ highly resistant, since this strain harbors deletions in seven major ABC transporter-encoding genes. In this host, any gene inserted into the PDR5 locus will be overexpressed due to the presence of a gain-of-function mutation (PDR1-3) that upregulates gene expression through the PDR5 promoter [Citation52]. In the present study, three DHA1 transporters of P. marneffei were successfully expressed using this yeast heterologous expression system.
The strains expressing PmMDR1, PmMDR2, and PmMDR3 showed varying levels of drug resistance. PmMDR1 conferred the highest resistance to a broad range of substrates, including not only azoles but also structurally and functionally unrelated compounds. PmMDR2 did not show a significantly higher resistance to most of the compounds compared to the effects of the other two PmMDR transporters. We infer that most of the tested drugs and compounds are not substrates of PmMDR2. PmMDR3 also conferred high-level resistance to azole drugs, and appeared to be an efficient efflux pump for azoles, especially FLC. In addition, it was suggested that the substrate specificity of PmMDR3 was similar to that of CaMDR1, aside from minor differences in susceptibility to MFG and CER (). Interestingly, PmMDR1 and ScTPO1 transport a broad range of substrates, including Quin, CHX, and Nys [Citation21,Citation53], whereas PmMDR2 and PmMDR3, which showed higher amino acid identities to ScTPO2 and ScTPO3, respectively, transport a narrower range of substrates [Citation22].
Polyamines are widely distributed in all living organisms and are essential substrates for the maintenance of cell growth, survival and macromolecular biosynthesis. Polyamines are known substrates of ScTPO1 to ScTPO4 [Citation21,Citation22]; however, S. cerevisiae AD∆ (and derivatives thereof) are unable to grow in either CSD or BM medium, which are recommended for the assessment of polyamine susceptibility. Therefore, we were unable to definitively determine whether polyamines are substrates of PmMDR1, PmMDR2, or PmMDR3.
We used the AD∆ system to express MFS transporter proteins from P. marneffei for phenotypic analysis. This yeast expression system also could be used to express other P. marneffei MFS proteins, including both those whose paralogs have been implicated in drug resistance and those whose paralogs lack known functions. The strains constructed in the present study, in particular AD∆/PmMDR1, are expected to find use as tools for screening of pump inhibitors. Such inhibitors might by employed in combination therapy with existing antifungal agents such as azoles to prevent DHA1 transporter-mediated drug resistance.
Authors’ contributions
STU, CII, AB, TY and XC performed the experiments. STU, KN, MN and SK analyzed the data. STU, KN, MN and SK wrote the manuscript. STU, and SK reviewed and edited the manuscript. All authors read and approved the final manuscript.
Table.supplement.S1.pdf
Download PDF (266.1 KB)Acknowledgments
We thank Astella Pharma Inc. for providing micafungin.
Data availability statement
The data described in this article are openly available in the Open Science Framework at DOI:10.17605/OSF.IO/TPA6U.
Disclosure statement
No potential conflict of interest was reported by the authors.
Supplementary material
Supplemental data of this article can be accessed here.
References
- Houbraken J, de Vries RP, Samson RA Modern taxonomy of biotechnologically important aspergillus and penicillium species [Internet]. 1st ed. Vol. 86. In: Advances in Applied Microbiology. Copyright © 2014 Elsevier Inc. All rights reserved; 2014. 199–249 p. doi: 10.1016/B978-0-12-800262-9.00004-4
- Vanittanakom N, Cooper CR, Fisher MC, et al. Penicillium marneffei infection and recent advances in the epidemiology and molecular biology aspects. Clin Microbiol Rev. 2006;19(1):95–110.
- Chan JFW, Lau SKP, Yuen KY, et al. Talaromyces (Penicillium) marneffei infection in non-HIV-infected patients. Emerg Microbes Infect [ Internet]. 2016;5(3):1–9.
- Wong SYN, Wong KF. Penicillium marneffei infection in AIDS. Path Research Int. 2011;2011(Figure 3):12–20.
- Kawila R, Chaiwarith R, Supparatpinyo K. Clinical and laboratory characteristics of penicilliosis marneffei among patients with and without HIV infection in Northern Thailand: A retrospective study. BMC Infect Dis. 2013;13(1):1–5.
- Sar B, Boy S, Keo C, et al. In vitro antifungal-drug susceptibilities of mycelial and yeast forms of Penicillium marneffei isolates in Cambodia. J Clin Microbiol. 2006;44(11):4208–4210.
- Hu Y, Zhang J, Li X, et al. Penicillium marneffei infection: an emerging disease in Mainland China. Mycopathologia. 2013;175(1–2):57–67.
- Le T, Kinh NV, Cuc NTK, et al. A trial of itraconazole or amphotericin B for HIV-associated talaromycosis. N Engl J Med. 2017;376(24):2329–2340.
- Supparatpinyo K, Schlamm HT. Voriconazole as therapy for Systemic Penicillium marneffei infections in AIDS patients. Am J Trop Med Hyg. 2007;77(2):350–353.
- Gulshan K, Moye-Rowley WS. Multidrug resistance in fungi. Eukaryot Cell. 2007;6(11):1933–1942.
- Pao SS, Paulsen IT, Saier MH. Major facilitator superfamily . Microbiol Mol Biol Rev [Internet]. 1998;62(1):1–34. Available from: http://www.ncbi.nlm.nih.gov/pubmed/9529885%0Ahttp://www.pubmedcentral.nih.gov/articlerender.fcgi?artid=PMC98904.
- Tanabe K, Lamping E, Nagi M, et al. Chimeras of Candida albicans Cdr1p and Cdr2p reveal features of pleiotropic drug resistance transporter structure and function. Mol Microbiol. 2011;82(2):416–433.
- Holmes AR, Lin YH, Niimi K, et al. ABC transporter Cdr1p contributes more than Cdr2p does to fluconazole efflux in fluconazole-resistant Candida albicans clinical isolates. Antimicrob Agents Chemother. 2008;52(11):3851–3862.
- Tsao S, Rahkhoodaee F, Raymond M. Relative contributions of the Candida albicans ABC transporters Cdr1p and Cdr2p to clinical azole resistance. Antimicrob Agents Chemother. 2009;53(4):1344–1352.
- Nakamura K, Niimi M, Niimi K, et al. Functional expression of candida albicans drug efflux pump Cdr1p in a saccharomyces cerevisiae strain deficient in membrane transporters. Antimicrob Agents Chemother. 2001;45(12):3366–3374.
- Sanglard D, Ischer F, Calabrese D, et al. The ATP binding cassette transporter gene CgCDR1 from candida glabrata is involved in the resistance of clinical isolates to azole antifungal agents. Antimicrob Agents Chemother. 1999;43(11):2753–2765.
- Posteraro B, Sanguinetti M, Sanglard D, et al. Identification and characterization of a Cryptococcus neoformans ATP binding cassette (ABC) transporter- encoding gene, CnAFR1, involved in the resistance to fluconazole. Mol Microbiol. 2003;47:357–371.
- Panapruksachat S, Iwatani S, Oura T, et al. Identification and functional characterization of Penicillium marneffei pleiotropic drug resistance transporters ABC1 and ABC2. Med Mycol. 2016;54(5):478–491.
- Saier MH, Reddy VS, Tsu BV, et al. The Transporter Classification Database (TCDB): recent advances. Nucleic Acids Res. 2016;44(D1):D372–9.
- Paulsen IT, Brown MH, Skurray RA. Proton-dependent multidrug efflux systems . Microbiol Rev [Internet]. 1996;60(4):575–608. Available from: http://www.ncbi.nlm.nih.gov/pubmed/8987357%0Ahttp://www.pubmedcentral.nih.gov/articlerender.fcgi?artid=PMC239457.
- Tomitori H, Kashiwagi K, Sakata K, et al. Identification of a gene for a polyamine transport protein in yeast. J Bio Chem. 1999;274:3265–3268.
- Tomitori H, Kashiwagi K, Asakawa T, et al. Multiple polyamine transport systems on the vacuolar membrane in yeast. Biochem J. 2001;353:681–688.
- Vargas RC, Tenreiro S, Teixeira MC, et al. Saccharomyces cerevisiae multidrug transporter Qdr2p (Yil121wp): localization and function as a quinidine resistance determinant. Antimicrob Agents Chemother. 2004;48(7):2531–2537.
- Teixeira MC, Cabrito TR, Hanif ZM, et al. Yeast response and tolerance to polyamine toxicity involving the drug: H + antiporter Qdr3 and the transcription factors Yap1 and Gcn4. Microbiology. 2010;157(4):945–956.
- Nunes PA, Tenreiro S, Sá-Correia I. Resistance and adaptation to quinidine in saccharomyces cerevisiae: role of QDR1 (YIL120w), encoding a plasma membrane transporter of the major facilitator superfamily required for multidrug. Antimicrob Agents Chemother [ Internet]. 2001 May 1;45(5):1528LP– 1534. Available from: http://aac.asm.org/content/45/5/1528.abstract
- Velasco I, Tenreiro S, Calderon IL, et al. Saccharomyces cerevisiae Aqr1 Is an internal-membrane transporter involved in excretion of amino acids. Eukaryot Cell [Internet]. 2004 Dec 1;3(6):1492LP– 1503. Available from: http://ec.asm.org/content/3/6/1492.abstract
- Felder T, Bogengruber E, Tenreiro S, et al. Dtr1p, a multidrug resistance transporter of the major facilitator superfamily, plays an essential role in spore wall maturation in saccharomyces cerevisiae. Eukaryot Cell [Internet]. 2002 Oct 1;1(5):799 LP– 810. Available from: http://ec.asm.org/content/1/5/799.abstract
- Gaber RF, Kielland-Brandt MC, Fink GR. HOL1 mutations confer novel ion transport in Saccharomyces cerevisiae. Mol Cell Biol [Internet]. 1990 Feb 1;10(2):643 LP– 652. Available from: http://mcb.asm.org/content/10/2/643.abstract
- Nguye D, Alarco A, Raymond M. Multiple Yap1p-binding sites mediate induction of the yeast major facilitator FLR1 gene in response to drugs, oxidants, and alkylating agents. J Biol Chem. 2001;276(2):1138–1145.
- Barker KS, Pearson MM, Rogers PD. Original articles Identification of genes differentially expressed in association with reduced azole susceptibility in Saccharomyces cerevisiae. J Antimicrob Chemother. 2003 Apr; 51(5):1131–1140.
- Calabrese D, Sanglard D, Bille J. A novel multidrug efflux transporter gene of the major facilitator superfamily from Candida albicans (FLU1) conferring resistance to fluconazole. Microbiology. 2015;146(11):2743–2754.
- Yamada-Okabe T, Yamada-Okabe H. Characterization of the CaNAG3, CaNAG4, and CaNAG6 genes of the pathogenic fungus Candida albicans: possible involvement of these genes in the susceptibilities of cytotoxic agents. FEMS Microbiol Lett. 2002;212(1):15–21.
- Shah AH, Singh A, Dhamgaye S, et al. Novel role of a family of major facilitator transporters in biofilm development and virulence of Candida albicans. Biochem J [Internet]. 2014 May 13;460(2):223–235.
- Costa C, Henriques A, Pires C, et al. The dual role of Candida glabrata drug: H+ antiporter CgAqr1 (ORF CAGL0J09944g) in antifungal drug and acetic acid resistance. Front Microbiol. 2013 Jun;4: 1–13.
- Costa C, Nunes J, Henriques A, et al. Candida glabrata drug: H+antiporter CgTpo3 (ORF CAGL0I10384G): role in azole drug resistance and polyamine homeostasis. J Antimicrob Chemother. 2014;69(7):1767–1776.
- Costa C, Pires C, Cabrito TR, et al. Candida glabrata drug: H+ antiporter CgQdr2 confers imidazole drug resistance, being activated by transcription factor CgPdr1. Antimicrob Agents Chemother. 2013;57(7):3159–3167.
- Pais P, Pires C, Costa C, et al. Membrane proteomics analysis of the Candida glabrata response to 5-flucytosine : unveiling the role and regulation of the drug efflux transporters CgFlr1 and CgFlr2 strains and growth media. Front Microbiol. 2016 Dec;7:1–14.
- Costa C, Dias PJ, Sá-Correia I, et al. MFS multidrug transporters in pathogenic fungi: do they have real clinical impact? Front Physiol. 2014 May; 5:1–8.
- Lamping E, Monk BC, Niimi K, et al. Characterization of three classes of membrane proteins involved in fungal azole resistance by functional hyperexpression in saccharomyces cerevisiae. Eukaryotic Cell. 2007;6(7):1150–1165.
- Mcginnis S, Madden TL. at the core of a powerful and diverse set of sequence analysis tools. Blast . 2004;32:20–25.
- Sievers F, Higgins DG. Clustal Omega for making accurate alignments of many protein sequences. Protein Sci. 2018;27:135–145.
- Furusawa H, Miyazaki Y, Sonoda S, et al. Penicilliosis marneffei complicated with interstitial Pneumonia. Intern Med. 2014;53(4):321–323.
- Lamping E, Ranchod A, Nakamura K, et al. Abc1p is a multidrug efflux transporter that tips the balance in favor of innate azole resistance in Candida krusei. Antimicrob Agents Chemother. 2009;53(2):354–369.
- Maruyama T, Masuda N, Kakinuma Y, et al. Polyamine-sensitive magnesium transport in Saccharomyces cerevisiae. BBA - Biomembr. 1994;1194(2):289–295.
- Cannon RD, Lamping E, Holmes AR, et al. Efflux-mediated antifungal drug resistance. Clin Microbiol Rev. 2009;22:291–321.
- Griffith JK, Rouch A, Paulsen IT, et al. Membrane transport implications of sequence proteins: comparisons. Curr Opin Cell Biol. 1992;4:684–695.
- Kovalchuk A, Driessen AJM. Phylogenetic analysis of fungal ABC transporters. BMC Genomics. 2010;11:1.
- Janbon G, Ormerod KL, Paulet D, et al. Analysis of the genome and transcriptome of cryptococcus neoformans var. grubii reveals complex RNA expression and microevolution leading to virulence attenuation. PLoS Genet. 2014;10:4.
- Coleman JJ, Mylonakis E. Efflux in fungi: la pièce de résistance. PLoS Pathog. 2009;5:6.
- Niimi M, Wada S, Tanabe K, et al. Functional analysis of fungal drug efflux transporters by heterologous expression in saccharomyces cerevisiae. Jpn J Infect Dis. 2005;58(1):1–7.
- Holmes AR, Tsao S, Ong S, et al. Heterozygosity and functional allelic variation in the Candida albicans efflux pump genes CDR1 and CDR2. Mol Microbiol. 2006 Aug; 62(1): 170–186.
- Nichols JW, de Wet H, McIntosh DB, et al. ATPase and multidrug transport activities of the overexpressed yeast ABC protein Yor1p. J Biol Chem. 2002;273(20):12612–12622.
- Sá-Correia I, Dos Santos SC, Teixeira MC, et al. Drug:H+antiporters in chemical stress response in yeast. Trends Microbiol. 2009;17(1):22–31.