ABSTRACT
The molecular mechanism of autophagy in Lactoferrin (LF) induced osteoblast differentiation is not fully demonstrated. In this study, alkaline phosphatase (ALP) activity, alizarin red S staining and ELISA were used to study N-terminal propeptide of type I procollagen (PINP) expression. mRFP-GFP-LC3 adenoviruses, mono-dansylcadaverine (MDC) staining, scanning electron microscopy, and western blot analysis was employed to probe the LF induced autophagy. The interaction between autophagy receptor Neighbor of Brca1 gene (Nbr1) and pp38 was studied. 3-methyladenine (3-MA) and chloroquine (CQ) could inhibit the activity of ALP, PINP and the autophagy in LF group. LF treatment could up-regulate and down-regulate the expressions of pp38 and Nbr1with a dose-dependent manner, respectively. LF could inhibit the recognition of pp38 and Nbr1. In addition, LF can prompt Nbr1-medicated autophagy and prevent pp38 degradation by autophagy. LF can induce Nbr1-mediated autophagy and inhibit pp38 entering into autophagy flux in the physiological process of osteoblast differentiation.
Abbreviations: CQ:chloroquine;LF: Lactoferrin; 3-MA: 3-methyladenine; ALP: Alkaline phosphatase; ANOVA: Analysis of variance; CCK-8: Cell Counting Kit-8; LC3: Microtubule-associated protein light chain3; MDC: Monodansylcadaverine; Nbr1: neighbor of Brca1 gene; PINP: N-terminal propeptide of type I procollagen; PVDF: Polychlorotrifluoroethylene; pp38: phosphorylation p38; RAPA: Rapamycin; SDS: sodium dodecyl sulfate.
Graphical Abstract
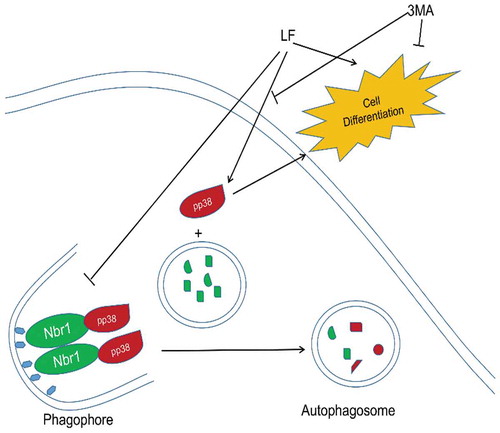
LF can induce Nbr1-mediated autophagy and inhibit pp38 entering into autophagy flux in the physiological process of osteoblast differentiation.
Postmenopausal osteoporosis is caused by progressive bone resorption capacity that exceeds progressive bone formation. This disease can lead to multiple malignant events, including microstructural destruction of bone tissue, decreased bone mass, increased bone fragility, and increased risk of fracture. Therefore, postmenopausal osteoporosis is a negative bone remodeling disease that is characterized by microstructural destruction of bone tissue [Citation1]. Although antiresorptive drugs have the effect of inhibiting negative bone remodeling, these drugs can only moderately increase bone density [Citation2]. The bone-promoting agents can achieve positive remodeling of bone balance by increasing bone remodeling ability. Therefore, these molecules can promote the acquisition of high bone mass [Citation3]. Osteoblast differentiation plays an important role in bone formation. Currently, bone-promoting drugs are attracting more and more attention to anti-osteoporosis research. However, the specific molecular mechanism of osteoblast differentiation has not been fully demonstrated.
Lactoferrin (LF) is an 80-kDa iron-binding glycoprotein derived from milk. This molecule has multiple beneficial biological properties for human health, including antimicrobial, antiviral, immunomodulatory and anti–inflammatory, anti-cancer and anti-osteoporosis [Citation4]. High expression of LF could be detected in human osteoblasts, osteoblasts, and fetal bone, suggesting its important role in promoting bone formation [Citation5]. A clinical study showed that postmenopausal osteoporosis treatment with LF had multiple significant benign effects, including reduced bone resorption, increased osteoblast formation, and restored bone turnover balance [Citation6]. LF injection in an adult mouse skull can effectively promote bone formation and increase bone mineral deposition [Citation7]. Moreover, LF exerts multiple benign effects by regulating the OPG/RANKL/RANK bone immune signaling pathway, such as increasing bone mass, trabecular number, thickness, and bone density in ovariectomized rats [Citation8]. In addition, LF has the potential to promote bone regeneration, thereby improving adverse clinical outcomes associated with bone nonunion in vivo and in vitro [Citation9,Citation10]. LF can promote osteoblast differentiation and maturation through the p38/MAPK signaling pathway. Meanwhile, p38/MAPK also plays an important role in the differentiation of bone marrow mesenchymal stem cells and osteoblasts [Citation11]. A previous study has shown that the physiological concentration of LF has an effect on the inhibition of osteoclastogenesis [Citation12]. Based on the above evidence, we speculate that LF should be a potential molecular for postmenopausal osteoporosis treatment.
Autophagy is a physiological process that transports substrates to autophagosomes through autophagy receptors. Autophagy can remove residuals and macromolecules from cells, which maintain the capability of cell renewal and homeostasis [Citation13]. Therefore, autophagy plays an important role in a variety of physiological processes such as development, tumor, neurodegeneration, and immunity [Citation14,Citation15]. Nbr1 is one of the co-receptors for selective autophagy. The truncation of Nbr1 in osteoblasts leads to excessive activation of p38 in the cell by avoiding Nbr1-mediated autophagy degradation, which is conducive to improving the osteoblast activity of postnatal bone in vivo [Citation16]. In addition, mutations in the autophagy co-receptor p62 lead to autophagy degradation of TRAF6, which can activate NF-κB signaling. Therefore, it can lead to increased osteoclast activity [Citation17]. Meanwhile, LC3 is currently the most thoroughly studied autophagy-related protein and can occur in advanced autophagolysosomes. When LC3 is produced in the cell, the C-terminus of the new LC3 is treated with ATG4 to generate LC3-I. When autophagy occurs, LC3-I binds to phosphatidylethanolamine and produces LC3-II under the action of ubiquitin-like enzymes. LC3-II is localized in pre-autophagosomes and autophagosomes, and is a marker molecule of autophagosomes. The ratio of LC3-II/LC3-I was positively correlated to the number of autophagosomes. Therefore, the ratio of the two can reflect the autophagy activity of the cell [Citation18]. Current research suggests that autophagy disorders in bone cells are closely related to the occurrence of bone diseases in vitro and in vivo [Citation19–Citation21]. Osteoblast maturation is accompanied by the formation of autophagic vesicles, which can transport the secrete apatite crystals [Citation22]. Meanwhile, a previous study has shown that estrogen promotes autophagy during osteoblast differentiation, which increases the survival rate and mineralization capacity of osteoblasts [Citation23]. Therefore, autophagy plays a key role in maintaining bone homeostasis.
Currently, the potential relationships between autophagy and LF-induced osteoblast differentiation have not been elucidated. The aim of this study was to examine the effects of LF on osteoblast differentiation and autophagy. In this work, our results suggest that lactoferrin plays multiple roles in LF-induced osteoblast differentiation, including promoting conversion rate of LC3-I to LC3-II, decreasing p62 expression, increasing autophagy in osteoblast differentiation. Moreover, LF-induced autophagy signaling pathway could inhibit p38 consumption caused by autophagy. The results obtained in this study would provide detailed information about the role of autophagy in LF-induced osteoblast differentiation.
Materials and methods
Reagents and antibodies
LF (Bovine source) with 95% purity was obtained from Wako Pure Chemical Industries, Ltd. (Japan). Rapamycin (V900930-1 MG) and MDC (RAB0064) were purchased from Sigma-Aldrich (USA). Hydroxy-chloroquine (CQ), 3-methyl adenine (3-MA) were purchased from Meilunbio (Dalian, China). The antibodies utilized in this study included LC3 (14600-1-AP) (1:800), P62 (18420-1-AP) (1:1500), GAPDH (60004-1-Ig) (1:100000) (Proteintech, USA), P38 (1:1000) (8690 S), pP38 (1:1000) (4511 S), and Nbr1 (1:1000) (9891 S) (Cell Signaling Technology, USA).
Cell culture and treatment
The mouse preosteoblastic cell line (MC3T3‐E1 subclone four) was purchased from the Cell Resource Center of the Chinese Academy of Sciences (Shanghai Academy of Biology, China). Cells were cultured with alpha-modified Eagle’s medium (α-MEM) (Solarbio, China), 10% fetal bovine serum (Thermo Scientific, USA), and 1% penicillin-streptomycin at 37°C with 5% CO2 in the incubator. Ascorbic acid (795437–100 G) (Sigma-Aldrich, USA) and beta-sodiumglycerophosphate (G5422-25 G) (Sigma-Aldrich, USA) were used to induce MC3T3-E1 differentiation according to the protocol provided by the manufacturer. After four days of induction, different LF concentrations were applied to treat cells on a 6-well culture plate. For the autophagy assay, cells were treated with or without LF (100 ugs/mL) after pretreatment with the inhibitor of autophagic interfering agents: 50 mM CQ treatment for 16 h, 7 mM 3 MA treatment for 2 h, rapamycin treatment for 2 h. Based on the different treatments, MC3T3-E1 cells were divided into the following groups: i) Control; ii) LF; iii) 3 MA; iv) 3 MA+LF; v) RAPA.
Cell proliferation assay
In this study, MC3T3-E1 cells were treated with LF concentrations, including 0, 1, 10, 100, 200, 300, 500 and 1000 ug/mL for 24, 48 and 72 h, respectively. Commercial CCK-8 kit (Beyotime, China) was applied according to the protocol provided by the manufacturer. The absorbance was measured at 450 nm using a microplate reader (Bio-Rad, USA).
ALP activity assay and Alizarin Red S staining
Cells were seeded into 24-well plates and cultured for 4 days in the inductive medium before LF treatment. Cells were washed three times with PBS after 24 h of treatment. 1% Triton X-100 was used to destroy cell membranes. The collected lysate was measured with an ALP activity assay kit (Beyotime, China). The detailed operation steps were strictly followed based on the instruction. Meanwhile, the total protein of cell lysate was examined with a bicinchoninic acid (BCA) protein assay kit (Meilunbio, China). Then, the ALP activity was normalized to the total protein. The differentia cell was fixed with 95% ethanol for 30 min at 37°C. Subsequently, cells were repeatedly washed two times with ddH2O. For calcium deposition study, cells were stained with 0.1% alizarin red S solution (ARS, pH 7.2) for 1 h. After the removal of ARS, the plates were observed with an optical microscope.
Monodansycadaverine (MDC) staining
After 24 h of 100 ug/mL LF treatment, cells were stained with 50 μM MDC at 37°C for 30 min. MDC was a selective fluorescent marker for autophagic vacuoles [Citation19]. Cellular fluorescence was observed and recorded with a fluorescence microscope (LAS Core, German).
Western blotting and Immunoprecipitation
The total cellular protein of MC3T3‐E1 cells in different treatments was isolated by the addition of 1% PMSF and RIPA lysis buffer (50 mM Tris-HCl (pH7.4), 150 mM NaCl, 1%NP-40, 0.1% SDS). After boiled with SDS-PAGE sample buffer for 5 min, the samples were performed for sodium dodecyl sulfate-polyacrylamide gel electrophoresis. Then the proteins were transferred onto a polyvinylidene difluoride membrane (Millipore, USA). After being blocked for 1 h at room temperature, the membrane was incubated with a 1:1000 dilution of rabbit polyclonal anti-mouse Nbr1 and pP38 overnight. Before detected with an ECL chemiluminescence detection kit (Advansta, USA), proteins were incubated with the corresponding secondary antibody for 1 h at room temperature. The bands were obtained by GeneGnome 5 (Synoptics Ltd., UK).
Transfection of adenoviruses mRFP-GFP-LC3
The cells were seeded in a 6-well plate and incubated with a 1:1 mixture of adenoviruses solution and culture medium for 6 h. The adenoviruses of double-tagging-LC3 with green/red fluorescent protein-LC3 was obtained from Hanbio Technology (Shanghai, China). Autophagic flux was analyzed with a Laser Scanning Confocal Microscope (LSCM, Nikon A1, Japan). The distribution and of mRFP-GFP- LC3B fluorescent signal was monitored.
Confocal immunofluorescence
Cells were fixed with cold 100% methanol for 15 min at room temperature and dried naturally for 10 min. Fixed cells were rinsed with PBS three times. After blocking with 5% BSA, primary antibodies of Nbr1 and pP38 were co-cultured with cells for 18 h. Polyclonal goat anti-rabbit/anti-mouse antibodies labeled with Alexa Fluor 488 and 594 (ABclonal, China) were applied as secondary antibodies. Laser scanning confocal microscopy (LSCM) was used to observe the fixed cells. Quantification analysis was performed with ImageJ software (National Institutes of Health, USA).
Transmission electron microscopy
MC3T3-E1 cells in different treated groups were collected. The obtained cells were fixed with 2.5% glutaraldehyde in 0.1 M cacodylate buffer (pH 7.3) at room temperature for 20 min. Subsequently, 1% osmium tetroxide buffer was applied to further treat cells at 20°C for 2 h. The samples were progressively dehydrated in gradient dilution of ethanol solution (50, 70, 80, 90, 95 and 100%) and embedded in the Epon 812 mixture overnight. The sections were observed using hibachi 7700 Transmission EM(Tokyo, Japan).
Statistical analysis
Data are were expressed as the mean ± SD. Statistical differences among groups were assessed with a two-way ANOVA analysis in Prism software (GraphPad, La Jolla, CA). P < 0.05 is considered as the significant difference.
Results
LF on MC3T3-E1 cells viability
In this study, MC3T3-E1 cells were treated with different LF concentrations (0, 1, 10, 100, 200, 300, 500, and 1000 ug/mL) (). The results showed that different concentrations of LF had no significant inhibitory effect on MC3T3-E1 cell activity after 24 h treatment. Meanwhile, the cell viability in the 500 ugs/mL and 1000 ug/mL LF treatment group was significantly lower than that in the control group (48 h after treatment) (P < 0.05). Moreover, the cell viability in the 200, 300, 500, and 1000 ug/mL LF treatment group were significantly lower than that in control group (72 h after treatment) (P < 0.05). Based on the evidence mentioned above, 100ug/mL LF was selected as the appropriate experimental concentration for further experiments.
LF on the differentiation of MC3T3-E1 and autophagy flux
In this study, we have employed different doses of LF, including 0, 50, 100, 200, and 500 ug/mL, to study ALP activity changes in MC3T3-E1 cells. ) suggested that low-dose LF (0, 50, 100, and 200ug/mL) treatments had better ALP activity than that in 500ug/mL LF treatment. It was notable that ALP activity in 100ug/mL LF treated group was significantly higher than that in the control group (P < 0.05) ()). Moreover, we have examined N-terminal propeptide of type I procollagen (PINP) expression and LF concentration in the culture medium. The results suggested that PINP expression in 500 ug/mL LF treated group was significantly higher than that in control, 100 ug/mL, and, 200 ug/mL LF treated group at 7 h after treatment (P < 0.05) ()). Meanwhile, PINP expression in 100 ug/mL and 200 ug/mL LF treated group was significantly higher than that in the control group at 24 h after treatment (P < 0.05) ()). It was notable that no difference of PINP expression could be detected between 500 ug/mL LF treated group and control group. Moreover, we have employed the western blot approach to study the Runx-2 expression which was the biomarker of osteocyte differentiation. The results suggested that Runx-2 expression with dosage manner in 50, 100, 200, and 500 ug/mL group was significantly higher than that in the control group (P < 0.001) ()). Meanwhile, we have examined the autophagy-related markers (P62 and LC3) with western blot. ) showed that LC3 expression with dosage manner in 100, 200, and 500 ug/mL group were significantly higher than that in the control group after 7 h LF treatment (P < 0.001) ()). However, no difference in LC3 expression could be calculated between 100, 200, 500 ug/mL group, and control at 24 h after LF treatment. P62 expression with a dosage manner in 200 and 500 ug/mL group was significantly higher than that in the control group at 7 h after LF treatment (P < 0.001) ()). However, no difference in LC3 expression could be calculated between 100, 200, 500 ug/mL group, and control group at 24 h after LF treatment. In addition, confocal microscopy was employed to study mRFP-GFP-LC3 labeled autophagosome and autolysosome in LF treated cells after 24 h treatment. ) showed that more GFP-LC3 (green) and mRFP-LC3 (red) labeled autophagosome and autolysosome in 200 and 500 ug/mL group could be observed than that in the control group. This result suggested that LF could stimulate autophagy flux during LF-induced preosteoblasts differentiation processes. In addition, SEM analysis of LF treated cells suggested that more autophagosomes could be retrieved in experimental groups than those in the control group ()). In summary, LF treatment could promote autophagy occurrence in LF induced MC3T3-E1 cells.
Figure 2. Effect of lactoferrin on preosteoblasts differentiation.
(a) Effect of lactoferrin on ALP activity in Control, 50, 100, 200, and 500ug/mL LF treated groups. MCT3-E1 cells were cultured with different LF concentrations for 24 h. (b) Protein expression of PINP (bone formation marker) and LF in the cell-culture medium were measured with ELISA kit at 7 h and 24 h after LF treatments. (c) Western blot analysis of osteoblastic differentiation-related Runx-2 protein expression in different LF treated groups (Control, 100, 200, and 500ug/mL LF treated group). GAPDH was used as an internal control.
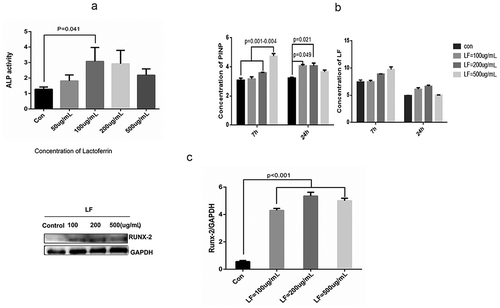
Figure 3. The role of autophagic flux in lactoferrin induced MCT3-E1 cells preosteoblasts.
(a) Western blot analysis of autophagy-related protein expressions(LC3 I, LC3 II, P62) at 24 h and 72 h after different LF dosages treatments. GAPDH was used as an internal control. (b) Immunofluorescence analysis of autolysosome related LC3 expression with Ad-GFP-mRFP-LC. GFP-LC3 and mRFP are green puncta and red puncta, respectively. Scale bar = 100 μm. (c) Scanning electron microscope (SEM) analysis of the autophagosome morphology in control and 100ug/mL lactoferrin treated group. Scale bar = 2 μm.
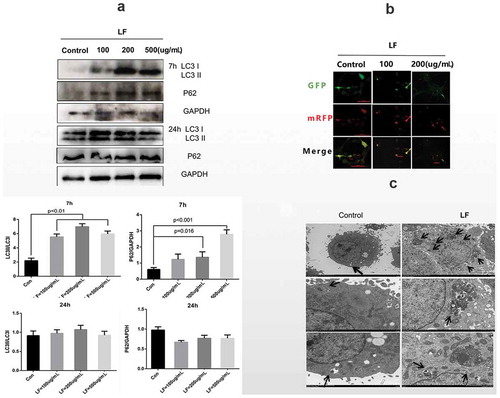
Autophagy and MC3T3-E1 cells differentiation
To investigate the role of autophagy in lactoferrin-mediated MC3T3-E1 differentiation, we have employed 3 MA and rapamycin to probe the further function of autophagy in drug-induced cell differentiation. Cells with different treatments were divided into five groups: Control, LF, 3 MA, 3 MA+ LF, and RAPA group. The results suggested that the number of MDC labeled vacuoles in the 3 MA treatment group was significantly less than that in the LF treatment group. 3 MA+LF treatment can promote the number of MDC-labeled vacuoles compared with that in 3 MA treatment. Meanwhile, as a positive control for autophagy, rapamycin can significantly induce the number of MDC-labeled autolyzates ()). Confocal fluorescent analysis of mRFP-GFP-LC3 revealed that the fluorescence intensity in 3 MA+ LF group was significantly weaker than that in 3 MA group ()). A similar situation could also be detected in SEM analysis ()). That is the number of autophagosomes and autolysosomes in 3 MA+ LF group was significantly less than that in 3 MA group ()). Moreover, we have further analysis of the ALP activity in five different groups. ) showed that ALP activity in the LF group was significantly higher than that in control, 3 MA, 3 MA+ LF, and RAPA group (P < 0.001). The lowest ALP activity could be retrieved in 3 MA group. Western blot analysis suggested that PINP expression in 3 MA+ LF group and CQ+LF group were significantly lower than those in LF group at 7 h after treatment (P < 0.001) (). Meanwhile, PINP expression in RAPA group was significantly higher than that in CQ+RAPA group at 7 h after treatment (P < 0.05) (). However, the differences in PINP expression could not be detected at 24 h. These results showed that LF had the strongest effect on PINP expression. Mineralization assessment indicated a positive effect of LF on MC3T3-E1 cell differentiation (). Therefore, LF-induced autophagy is beneficial to osteoblast differentiation.
Figure 4. Effect of lactoferrin on preosteoblasts differentiation-related autophagic flux.
(a) MDC staining analysis of autophagosomes and autolysosomes in different treated groups. LF: lactoferrin treatment; 3 MA: autophagy inhibitor 3-MA treatment; 3 MA+LF: lactoferrin and autophagy inhibitor 3-MA treatment; RAPA: autophagy activator rapamycin treatment. Scale bar = 50 μm. (b) Immunofluorescence analysis of preosteoblastic differentiation with Ad-GFP-mRFP-LC3. GFP-LC3 and mRFP are green puncta and red puncta, respectively. Scale bar = 100 μm. (c) Scanning electron microscope (SEM) analysis of the autophagosome morphology of control and 3 MA+LF treated group in MC3T3-E1 cells. Scale bar = 2 μm. (d) ALP activity analysis in five different groups. (e) Protein expression of PINP (bone formation marker) in the cell culture medium was measured with ELISA kit at 7 h and 24 h in five different groups. (f) Alizarin red staining analysis of osteogenesis differentiation in five different groups.
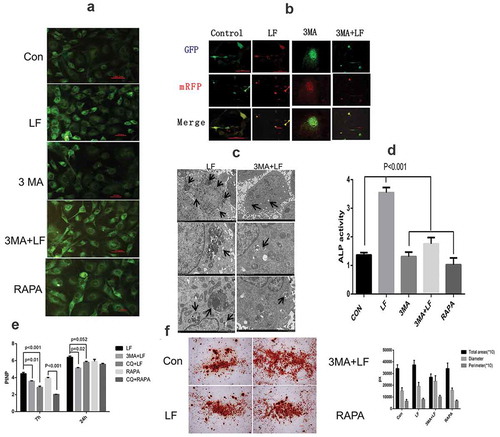
Autophagy was reducted via Nbr1 and pp38 in lactoferrin-induced MC3T3-E1 cell differentiation
In order to investigate the molecular mechanisms of autophagy in lactoferrin-induced MC3T3-E1 cell differentiation, we have analyzed the expressions of Nbr1 and P38 in 0, 100, 200 ugs/mL LF treated groups. The results suggested that P38 expression with a dosage manner was significantly higher than that in 100, 200 ugs/mL LF treated groups than that in the control group (P < 0.01). However, Nbr1expression with a dosage manner was significantly lower than that in 100, 200 ugs/mL LF treated groups than that in the control group (P < 0.01) ()). Moreover, in order to further explore the association between Nbr1, pp38, and autophagy, we used the autophagy inhibitor 3 MA and chloroquine (CQ) as pretreatment drugs before adding LF. Subsequently, we have examined the Nbr1 and P38 expressions in different groups (LF group, RAPA group, 3 MA group, and 3 MA+LF group). ) showed that pp38 expression in LF group was significantly higher than that in RAPA group, 3 MA group, and 3 MA+LF group without adding CQ (P < 0.01). This result revealed that 3 MA treatment could inhibit pp38 expression. However, Nbr1 expression in 3 MA group and 3 MA+LF group were significantly higher than that in LF group and RAPA group without adding CQ (P < 0.01). This result revealed that 3 MA treatment could promote Nbr1 expression ()). Moreover, CQ could reverse LF-induced expression of pp38 and Nbr1 in different groups. It is worth noticing that the autophagy activator rapamycin could not promote the expression of pp38 compared with that in LF treatment. In addition, a similar situation could be observed in immunofluorescence analysis ()). ) showed that LF could promote Nbr1 expression which was aggregated with high pp38 expression. However, RAPA could relieve autophagy. Therefore, LF is an effective drug to change Nbr1 and pp38 expressions.
Figure 5. Autophagy related pp38 and Nbr1 protein expression analysis in LF induced preosteoblastic differentiation. (a) Western blot analysis of p38, pp38, and Nbr1 expressions in LF (100ug/mL and 200ug/mL) induced preosteoblastic differentiation. (b) Western blot analysis of p38, pp38, and Nbr1 expressions in LF (100ug/mL and 200ug/mL) induced preosteoblastic differentiation with or without CQ treatment. GAPDH was used as an internal reference.
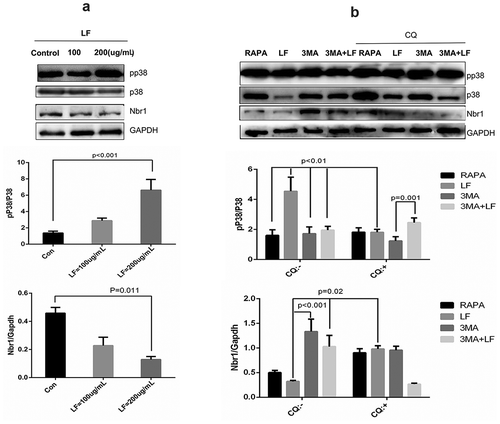
Figure 6. Subcellular distribution and expression of autophagy-related pp38 and Nbr1 in LF induced preosteoblasts differentiation. (a) Immunofluorescence analysis of Alexa Fluor-488 and Alexa Fluor-594 conjugated pp38 and Nbr1 in five different groups. (b) Co-immunoprecipitate analysis of pp38 and Nbr1 in four different groups.
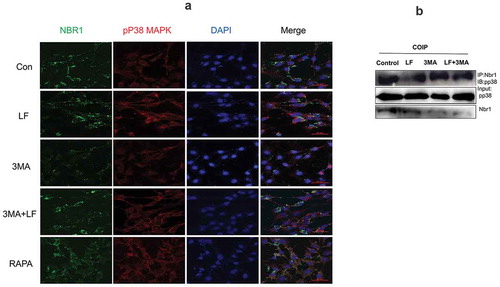
Discussion
The best way to enhance bone resistance to fractures is to promote osteoblast differentiation and improve bone quality. Although LF is considered to be an effective bone anabolic agent for mitosis and differentiation of osteoblasts, its mechanism of inducing differentiation and cell signal transduction pathways is still unclear [Citation24].
Our current results suggest that low concentrations of LF can promote osteoblast differentiation, which is closely related to Nbr1-regulated autophagy. Moreover, LF can inhibit the binding of Nbr1 to pp38 to prevent pp38 from entering the autophagosome-associated degradation pathway. In conclusion, LF promotes osteoblast differentiation by inhibiting substrate recognition in Nbrl mediated autophagy. Therefore, LF treatment can maintain a high expression of pp38 in cells. Autophagy inhibitors attenuate Nbr1-mediated autophagy and LF-induced osteoblast differentiation. Recently, there is increasing evidence that autophagy plays an important role in the proliferation, differentiation, and anti-apoptosis of osteoblasts [Citation25,Citation26]. Furthermore, previous studies have shown that estrogen as a recognized bone protector can increase osteoblast survival and mineralization by promoting autophagy [Citation23,Citation27]. Nbr1 is one of the co-receptors of large phage or selective autophagy [Citation28]. The truncation of Nbr1 in osteoblasts can inhibit Nbr1-mediated degradation of autophagy throughout the life cycle of mice. The above molecular processes can lead to excessive activation of p38 in the cells, which is beneficial to increase the osteoblast activity of the bone after birth [Citation29]. In addition, p62 is another autophagy co-receptor. P62 mutation can cause autophagy degradation of TRAF6 and activate NF-κB signaling, resulting in increased osteoclast activity [Citation30]. Based on the above evidence, autophagy receptors can recognize different degradants and alter the activity of bone cells. pp38 can activate or enhance some key transcription factors, including BMP, TGFβ, Wnt, estradiol, and PTH, Thus pp38 can promote the expression of osteogenic genes [Citation31]. The DNA damage is beneficial for phosphorylation and nuclear accumulation of P38. In this study, P38 and pp38 are usually located in the cytoplasm, which is consistent with our immunofluorescence results [Citation23]. Therefore, P38 plays an important role in osteoblast proliferation, migration, differentiation and osteogenic matrix remodeling [Citation25,Citation26].
Our results indicate that LF reduces pp38 consumption by up-regulating Nbr1-mediated autophagy. Therefore, LF can increase the pp38 content in the cytoplasm, which promotes the differentiation of osteoblasts. Similarly, a previous study has shown that LF-induced autophagy is also present in fibroblasts. Fibroblasts have the same source of autophagy as osteoblasts. LF-LRP1-AMPK is responsible for the production of autophagy in fibroblasts [Citation29]. In addition, bLF can significantly inhibit osteosarcoma-activated RANKL signaling pathways, thereby inducing osteoclastogenesis [Citation31]. A previous study has suggested that LF can act as an anabolic factor for osteoblast differentiation and bone matrix deposition in co-cultured pre-osteoblasts and monocyte/macrophage cell lines. LF can also act as an inhibitor of osteoclastogenesis and activity [Citation32]. Furthermore, the TβRII-dependent TGF-β signaling pathway plays an important role in osteoblast induction in MC3T3-E1 cells and C57BL/6 J mice [Citation33]. In addition, It has been reported that LF may promote osteogenesis through IGF1 signaling to prevent senile osteoporosis [Citation34].
In this study, low doses of LF could induce high autophagy fluxes in the early stage of osteoblast differentiation in a dosage-dependent manner. Although autophagy flux is partially blocked by high doses of LF, autophagy markers are still abundantly expressed. In vitro, 3 MA can partially inhibit LF-induced autophagy and differentiation of osteoblasts. In contrast, rapamycin, a nonselective autophagy agonist, activates pre-osteoblast differentiation. But F may produce more Nbr1 labeled autophagy. In vitro, 3 MA can partially inhibit LF-induced autophagy and differentiation of osteoblasts. In contrast, rapamycin, a nonselective autophagy agonist, activates pre-osteoblast differentiation. However, LF could produce more Nbr1 labeled autophagosomes [Citation35]. Therefore, LF plays multiple roles in osteoblast differentiation, including promoting Nbr1-mediated autophagy, negatively regulating cytoplasmic Nbr1, and blocking Nbr1 recognition of pp38. Therefore, LF can promote osteoblast differentiation, which is consistent with the negative regulation of Nbr1 on bone density in vivo. Although our work firstly shows Nbr1-mediated autophagy involved in LF-induced osteoblast differentiation, multiple issues still remain needed to be solved in future studies. For example, whether LF induced autophagy is macroautophagy or selective autophagy? Does this autophagy degrade p38 phosphorylase inhibitors?
Conclusions
In this study, our results revealed that LF can induce Nbr1-mediated autophagy and inhibit pp38 from entering into autophagy flux. LF could be a potential drug for osteoporotic treatment.
Author Contributions
Author contributions are as follows. Study design: Hou, Yang and Wu. Data collection and sampling: Yang, Zi-Nan, Xue, Li, and Zhao. Data analysis: Yang. Manuscript preparation: Yang and Hou.
Statement of Ethics
This study was approved by the Ethics Committee of Fujian Medical University.
Acknowledgments
The authors would give our sincere thanks to Dr. Shen Zhao from the Emergency Department, Fujian Provincial Hospital. Dr. Shen Zhao had provided helpful suggestions on experimental processes.
Disclosure Statement
The authors declare that they have no conflicts of interest.
Additional information
Funding
References
- Rizzoli R. Postmenopausal osteoporosis. Assessment and management. Best Pract Res Clin Endocrinol Metab. 2018;32(5):739–757.
- Boivin G, Farlay D, Bala Y, et al. Influence of remodeling on the mineralization of bone tissue. Osteoporos Int. 2009;20(6):1023–1026.
- Compston JE, McClung MR, Leslie WD. Osteoporosis. Lancet. 2019;393(10169):364–376.
- Lepanto MS, Rosa L, Paesano R, et al. Lactoferrin in aseptic and septic inflammation. Molecules. 2019;24(7):E1323.
- Amini AA, Nair LS. Lactoferrin: a biologically active molecule for bone regeneration. Curr Med Chem. 2011;18(8):1220–1229.
- Bharadwaj S, Naidu AG, Betageri GV, et al. Milk ribonuclease-enriched lactoferrin induces positive effects on bone turnover markers in postmenopausal women. Osteoporos Int. 2009;20(9):1603–1611.
- Amini AA, Kan HM, Cui Z, et al. Enzymatically cross-linked bovine lactoferrin as injectable hydrogel for cell delivery. Tissue Eng Part A. 2014;20(21–22):2830–2839.
- Hou JM, Xue Y, Lin QM. Bovine lactoferrin improves bone mass and microstructure in ovariectomized rats via OPG/RANKL/RANK pathway. Acta Pharmacol Sin. 2012;33(10):1277–1284.
- Gao R, Watson M, Callon KE, et al. Local application of lactoferrin promotes bone regeneration in a rat critical-sized calvarial defect model as demonstrated by micro-CT and histological analysis. J Tissue Eng Regen Med. 2018;12(1):e620–e626.
- Shi P, Wang Q, Yu C, et al. Hydroxyapatite nanorod and microsphere functionalized with bioactive lactoferrin as a new biomaterial for enhancement bone regeneration. Colloids Surf B Biointerfaces. 2017;155:477–486.
- Liu M, Fan F, Shi P, et al. Lactoferrin promotes MC3T3-E1 osteoblast cells proliferation via MAPK signaling pathways. Int J Biol Macromol. 2018;107(PtA):137–143.
- Cornish J, Palmano K, Callon KE, et al. Lactoferrin and bone; structure-activity relationships. Biochem Cell Biol. 2006;84(3):297–302.
- Chun Y, Kim J. Autophagy: an essential degradation program for cellular homeostasis and life. Cells. 2018;7(12):E278.
- Kuma A, Komatsu M, Mizushima N. Autophagy-monitoring and autophagy-deficient mice. Autophagy. 2017;13(10):1619–1628.
- Mizushima N, Komatsu M. Autophagy: renovation of cells and tissues. Cell. 2011;147(4):728–741.
- Mardakheh FK, Auciello G, Dafforn TR, et al. Nbr1 is a novel inhibitor of ligand-mediated receptor tyrosine kinase degradation. Mol Cell Biol. 2010;30(24):5672–5685.
- Islam M, Sooro MA, Zhang P. Autophagic regulation of p62 is critical for cancer therapy. Int J Mol Sci. 2018;19(5):1405.
- Chude CI, Amaravadi RK. Targeting autophagy in cancer: update on clinical trials and novel inhibitors. Int J Mol Sci. 2017;18(6):E1279.
- Jaber FA, Khan NM, Ansari MY, et al. Autophagy plays an essential role in bone homeostasis. J Cell Physiol. 2019;234(8):12105–12115.
- Ma Y, Qi M, An Y, et al. Autophagy controls mesenchymal stem cell properties and senescence during bone aging. Aging Cell. 2018;17:1.
- Pantovic A, Krstic A, Janjetovic K, et al. Coordinated time‐dependent modulation of AMPK/Akt/mTOR signaling and autophagy controls osteogenic differentiation of human mesenchymal stem cells. Bone. 2013;52(1):524–531.
- Nollet M, Santucci-Darmanin S, Breuil V, et al. Autophagy in osteoblasts is involved in mineralization and bone homeostasis. Autophagy. 2014;10(11):1965–1977.
- Gavali S, Gupta MK, Daswani B, et al. Estrogen enhances human osteoblast survival and function via promotion of autophagy. Biochim Biophys Acta Mol Cell Res. 2019;1866(9):1498–1507.
- Tang DZ, Hou W, Zhou Q, et al. Osthole stimulates osteoblast differentiation and bone formation by activation of beta-catenin-BMP signaling. J Bone Miner Res. 2010;25(6):1234–1245.
- Biederbick A, Kern HF, Elsässer HP. Monodansylcadaverine (MDC) is a specific in vivo marker for autophagic vacuoles. Eur J Cell Biol. 1995;66(1):3–14.
- Liu Y, Shi S, Gu Z, et al. Impaired autophagic function in rat islets with aging. Age (Dordr). 2013;35(5):1531–1544.
- Liu F, Fang F, Yuan H, et al. Suppression of autophagy by FIP200 deletion leads to osteopenia in mice through the inhibition of osteoblast terminal differentiation. J Bone Miner Res. 2013;28(11):2414–2430.
- Qi M, Zhang L, Ma Y, et al. autophagy maintains the function of bone marrow mesenchymal stem cells to prevent estrogen deficiency-induced osteoporosis. Theranostics. 2017;7(18):4498–4516.
- Kirkin V, Lamark T, Sou YS, et al. A role for NBR1 in autophagosomal degradation of ubiquitinated substrates. Mol Cell. 2009;33(4):505–516.
- Whitehouse CA, Waters S, Marchbank K, et al. Neighbor of Brca1 gene (Nbr1) functions as a negative regulator of postnatal osteoblastic bone formation and p38 MAPK activity. Proc Natl Acad Sci U S A. 2010;107(29):12913–12918.
- Waters S, Marchbank K, Solomon E, et al. Autophagic receptors Nbr1 and p62 coregulate skeletal remodeling. Autophagy. 2010;6(7):981–983.
- Rodríguez-Carballo E, Gámez B, Ventura F. 38 MAPK signaling in osteoblast differentiation. Front Cell Dev Biol. 2016;4:40.
- Li Y, Wang J, Ren F, et al. Lactoferrin promotes osteogenesis through TGF-β receptor II binding in osteoblasts and activation of canonical TGF-β signaling in MC3T3-E1 cells and C57BL/6J mice. J Nutr. 2018;148(8):1285–1292.
- Chen X, Li YH, Zhang MJ, et al. Lactoferrin ameliorates aging-suppressed osteogenesis via IGF1 signaling. J Mol Endocrinol. 2019;pii:JME-19–0003.R3.
- Oliver L, Hue E, Priault M, et al. Basal autophagy decreased during the differentiation of human adult mesenchymal stem cells. Stem Cells Dev. 2012;21(15):2779–2788.