ABSTRACT
Transmembrane 2 (TMEM2) gene inhibits chronic hepatitis-B virus (HBV) infection, while the underlying molecular mechanisms remain unknown. Transcriptome alterations in HepG2 cells following TMEM2 overexpression or silencing by shRNA were analyzed by next-generation sequencing. Both overexpression and knockdown of the TMEM2 gene caused wide-spread changes in gene expression in HepG2 cells. Differentially expressed genes caused by altered TMEM2 gene expression were associated with multiple biological processes linked with viral infection and various signaling pathways. KEGG analysis revealed that many of the differentially expressed genes were enriched in the PI3K/AKT signaling pathway. Moreover, we show that genes related to the PI3K/AKT signaling pathway, such as SYK, FLT4, AKT3, FLT1, and IL6, are biological targets regulated by TMEM2 in HepG2 cells. This is the first transcriptome-wide study in which TMEM2-regulated genes in HepG2 cells have been screened. Our findings elucidate the molecular events associated with TMEM2-mediated hepatocyte pathogenesis in chronic HBV infection.
GRAPHICAL ABSTRACT
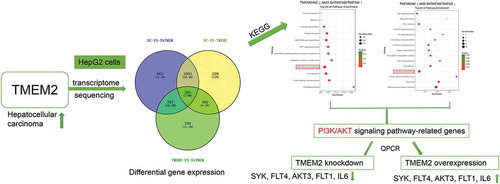
A large-scale analysis of gene expression profile alterations in HepG2 cells induced by TMEM2 knockdown and overexpression by transcriptome sequencing.
Chronic hepatitis-B virus (HBV) infection is a global public health problem and contributes to a large portion of liver-related morbidity and mortality worldwide [Citation1,Citation2]. It is usually acquired at birth or through person-to-person transmission. HBV infection has been established as a major risk factor for liver cirrhosis, which could further progress to liver failure and hepatocellular carcinoma (HCC) [Citation2]. Vaccination against HBV at birth, initiated nearly three decades ago, has greatly reduced the incidence of HBV infection and transmission, which has been a major breakthrough in the clinical management of HBV infection [Citation1]. Furthermore, the combined application of multiple assessment modalities such as virologic markers, liver biochemistry, and abdominal ultrasonography, as well as long-term oral nucleotide analogues and pegylated interferon treatment has enabled effective viral suppression and reduced incidence of liver-related complications [Citation1]. However, current therapies for chronic HBV infection are limited due to various adverse effects such as bone marrow suppression and poor tolerability [Citation2]. The global chronic HBV infection prevalence was estimated to be as high as 3.5% in 2016, indicating that over 250 million people are living with HBV infection [Citation3]. A better understanding of the molecular mechanisms involved in the hepatic cellular response to HBV infection is essential for developing novel antiviral treatments and reaching the World Health Organization’s goal of eliminating viral hepatitis by 2030 [Citation4].
Substantial progress has been made in elucidating the viral life cycle of HBV over the past decades [Citation5,Citation6]. The HBV virion enters hepatocytes via endocytosis by binding to sodium taurocholate cotransporting polypeptide receptors on the surface of liver cells. Relaxed viral circular DNA is released, which is then transferred into the hepatocyte nucleus and converted into closed covalent circular DNA (cccDNA) [Citation2,Citation5,Citation6]. Viral cccDNA in the hepatocyte nucleus is responsible for the persistence of HBV and it serves as a template for the expression of key viral mRNA and proteins essential for the ensuing viral replication and release [Citation1,Citation5,Citation6]. The natural history of chronic HBV infection is highly variable and is greatly influenced by dynamic interactions between HBV and the host immune system, involving multiple effector cells such as natural killer cells, dendritic cells, and Kupffer cells [Citation1,Citation7,Citation8]. Importantly, the susceptibility of host liver cells to HBV infection could also influence the disease course, which was shown to be associated with genetic factors such as host gene polymorphisms [Citation9–Citation11]. However, the underlying molecular networks regulating host cell susceptibility to HBV infection are not well understood.
Transmembrane 2 (TMEM2) is a type II member of the TMEM protein family encoded by the p.Ser1254Asn gene and is characteristic of a putative transmembrane domain with poorly understood biological functions [Citation12]. Previous genetic reports using zebrafish models showed that TMEM2 regulates myocardial and endocardial morphogenesis and the development of atrioventricular canal boundary and endocardial cushion [Citation13,Citation14]. Recently, TMEM2 was identified as a novel cell surface hyaluronidase responsible for cleavage of high- to intermediate-molecular-weight hyaluronan in extracellular space during hyaluronan catabolism [Citation15,Citation16]. We previously demonstrated that TMEM2 expression was greatly reduced in the liver tissue of patients and HepG2 cells with chronic HBV infection, compared with controls. Moreover, the reduced TMEM2 expression was correlated with host cell susceptibility to HBV [Citation17]. We also showed that TMEM2 suppresses HBV infection in cultured HepG2 and HepG2.2.15 cells via activation of the Janus kinase-signal transducer and activator of transcription (JAK-STAT) signaling pathway [Citation18]. However, the molecular mechanisms involved in TMEM2-regulated hepatocyte susceptibility to HBV infection remain unclear and need further investigation.
In the present study, we performed a large-scale analysis of gene expression alterations in HepG2 cells induced by TMEM2 knockdown and overexpression using transcriptome sequencing. Furthermore, we identified genes involved in the PI3K-AKT signaling pathway as targets of TMEM2 regulation in HepG2 cells. These results have provided new insights into the role of TMEM2 in liver pathogenesis during chronic HBV infection.
Material and methods
Cell culture and transfection
The HepG2 cell line was purchased from the American Type Culture Collection (ATCC, Manassas, USA) and cultured in Dulbecco’s modified Eagle’s medium (DMEM; Life Technologies, Carlsbad, USA) supplemented with 10% fetal bovine serum (FBS; Gibco, Life Technologies, Carlsbad, USA), 0.1% streptomycin, and 100 units/mL penicillin in a humidified incubator at 37°C supplied with 5% CO2.
TMEM2 overexpression and silencing
HepG2 cells overexpressing the TMEM2 gene (HepG2 TMEM2 cells) and HepG2 cells with silenced TMEM2 expression (HepG2 shTMEM2 cells) by small hairpin RNAs (shTMEM2) were established as previously described [Citation18]. Briefly, TMEM2 cDNA cloned into the LV-003 vector with a cytomegalovirus promoter (Forevergen Biosciences; Guangzhou, China) was used to transfect HepG2 cells to induce stable overexpression of TMEM2. Small hairpin RNAs (shTMEM2) were cloned into the LV-008 vector (Forevergen Biosciences, Guangzhou, China) with a U6 promoter. The sequence of the oligonucleotides used for generation of shTMEM2 was as follows: shTMEM2-1 sense, 5ʹ-CACCGCTTGCCCTTTGGGTCCTATACGAATATAGGACC
CAAAGGGCAAGC-3ʹ; antisense, 5ʹ-AAAAGCTTGCCCTTTGGGTCCTATATTCGTATAGG
ACCCAAAGGGCAAGC-3ʹ. shTMEM2-2 sense, 5ʹ-CACCGCGGAGGATACAGACATGCAAC
ACGAATGTTGCATGTCTGTATCCTCCGC-3ʹ; antisense, 5ʹ-AAAAGCGGAGGATACAGAC
ATGCAACATTCGTGTTGCATGTCTGTATCCTCCGC-3ʹ. Lentivirus was produced by cotransfecting HEK293T cells with recombinant LV-003-TMEM2 plasmid or LV-008-shTMEM2 plasmid with packaging vectors. HepG2 cells were then infected with collected Lentivirus in DMEM with 6 mg/mL polybrene for 48 h, and stable cell lines were generated by culturing for 10–15 days in DMEM with 2 µg/mL puromycin. Overexpression and silencing of the TMEM2 gene in HepG2 cells were confirmed by quantitative RT-PCR and Western blotting.
Transcriptome sequencing and gene expression quantitation
Sequencing of HepG2 cell transcriptomes was performed using total RNA samples extracted from HepG2 cells, HepG2 TMEM2 cells, and HepG2 shTMEM2 cells using the PureLink Viral RNA/DNA Mini Kit (Cat No. 12280050; Thermo Fisher Scientific, Carlsbad, USA) according to the manufacturer’s instructions. After DNA removal by digestion with DNase I (Thermo Fishier Scientific, Carlsbad, USA), mRNA was enriched using oligo (dT) magnetic beads, followed by fragmentation in a Thermomixer, single and double-strand cDNA synthesis using random hexamer primers, cohesive end repairing, and 3ʹ-adapter ligation. The cDNA library was amplified by PCR and subjected to quality checking using an Agilent 2100 Bioanalyzer and ABI StepOnePlus Real-Time PCR System. Deep sequencing was performed using an Illumina HiSeqTM 2000 system. Quality checks were performed on raw reads from sequencing by analyzing the base percentage composition along with reads and the distribution of base qualities. Reads with adaptors or a high N base ratio (>10%) and low-quality reads were removed.
Reads that passed pre-processing were mapped to the human reference sequence using the SOAPaligner/SOAP2 software as previously described, allowing five mismatched base pairs [Citation19]. Quality checks of alignment were performed by analysis of the distribution of matched reads on reference sequence and distribution of gene coverage. Relative gene expression levels based on uniquely matched reads were then calculated using the reads per kilobase transcriptome per million mapped reads (RPKM) method as previously described [Citation20]. Genes with significantly differential expression were defined by a false discovery rate (FDR) <0.01 and an expressional ratio of no less than 2.
Expression pattern cluster and functional categorization
Cluster analysis based on gene expression patterns was carried out using Cluster software as previously described [Citation21]. Clustering results of the gene expression patterns were visualized using the JavaTreeview software, with downregulated and upregulated genes shown in green and red, respectively. Gene Ontology (GO) functional categorization was performed based on molecular function, cellular component, and biological process (http://www.geneontology.org/) using the GO TermFinder software. Significant enrichment of differentially expressed genes in GO terms was defined by a P-value ≤0.05 after Bonferroni correction. Kyoto Encyclopedia of Genes and Genomes (KEGG) pathway analysis was performed as previously described [Citation22] and significant enrichment in KEGG pathways was defined using FDR < 0.05 as a threshold.
RNA extraction and quantitative real-time PCR (RT-qPCR) assay
TRIzol method (Takara) was applied to extract total RNA, and BestarTM qPCR RT kit (DBI Bioscience, cat. no. #2220) was utilized to synthetize cDNA using total RNA as a template following manufacturer’s instructions. RT-PCR was carried out using BestarTM qPCR MasterMix (DBI Bioscience) on the ABI 7300 real-time system. The expression levels of genes were analyzed using 2−ΔΔCt method by normalizing to housekeeping genes [Citation23]. The sequences of primers used in this study are shown in .
Table 1. Sequences of primers used for quantitative RT-PCR method.
Statistical analysis
All data were presented as mean ± standard deviation. Statistical analysis was performed using SPSS 19.0 software (IBM, Armonk, USA). Differential gene expression was analyzed using Student’s t-test. P < 0.05 was considered significant.
Results
Differential gene expression in HepG2 cells with altered TMEM2 expression analyzed by transcriptome sequencing
To explore the role of TMEM2 in modulating chronic HBV infection, we performed transcriptome sequencing using HepG2 cells overexpressing the TMEM2 gene (TMEM2 group) or with silenced TMEM2 gene expression using shRNA (the ShTMEM2 group), and normal control (NC) HepG2 cells. Our results showed that 2,545 and 341 genes in the ShTMEM2 group were significantly upregulated and downregulated, respectively, compared with the NC group ()). In the TMEM2 group, expression of 1,850 and 567 genes was significantly upregulated and downregulated, respectively, compared with the NC group ()). Compared with the TMEM2 group, 1,524 and 808 genes were significantly upregulated and downregulated, respectively, in the ShTMEM2 group ()). In addition, a number of differentially expressed genes overlapped between different group pairs ()). Heatmap analysis showed that 651 differentially expressed genes were regulated by TMEM2 overexpression and shRNA-mediated silencing in HepG2 cells with opposite changes ()). Among these genes, 269 were upregulated by TMEM2 overexpression and downregulated by TMEM2 silencing, whereas 382 genes were downregulated by TMEM2 overexpression and upregulated by TMEM2 silencing in Hep G2 cells ()).
Figure 1. Genes differentially expressed following TMEM2 overexpression and silencing in HepG2 cells. (a) Differential gene expression between NC and TMEM2 groups, NC and ShTMEM2 groups, and TMEM2 and ShTMEM2 groups are shown as volcano plots. (b) Overlapping of differentially expressed genes between the NC, TMEM2, and ShTMEM2 groups, presented as Venn diagrams. (c) Expression pattern cluster analysis of genes that were differentially induced by TMEM2 overexpression and silencing in HepG2 cells. Gene expression patterns are presented in a heatmap. NC, normal control; TMEM2, transmembrane 2; ShTMEM2, TMEM2 shRNA.
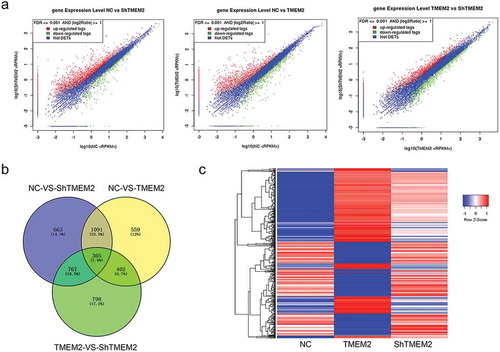
Functional categorization of differentially expressed genes following TMEM2 overexpression and silencing in HepG2 cells
TMEM2-regulated genes are defined as differentially expressed genes in HepG2 cells following overexpression or silencing of TMEM2 gene. Among them, those associated with virus infection were further analyzed for HepG2 gene expression alteration involved in chronic HBV infection. GO categorization showed that genes that were upregulated in HepG2 cells by TMEM2 overexpression but downregulated by TMEM2 gene silencing were significantly enriched in several GO terms associated with viral infection, including viral process, response to virus, viral life cycle, defense response to virus, regulation of viral process, regulation of viral life cycle, viral genome replication, and modulation by host of viral transcription ()). Furthermore, genes that were downregulated in HepG2 cells by TMEM2 overexpression and upregulated by TMEM2 gene silencing were greatly enriched in viral process, response to virus, defense response to virus, regulation of viral process, viral life cycle, viral entry to host cell, virus receptor activity, and virion part ()).
Figure 2. Biological processes and signaling pathways regulated by overexpression and silencing of TMEM2 in HepG2 cells. (a) GO biological processes related to viral infection with significant enrichment of genes upregulated by TMEM2 overexpression and downregulated by TMEM2 silencing in HepG2 cells. (b) GO biological processes with significant enrichment of genes downregulated by TMEM2 overexpression and upregulated by TMEM2 silencing in HepG2 cells. (c) KEGG pathways related to viral infection with enrichment of genes upregulated by TMEM2 overexpression and downregulated by TMEM2 silencing in HepG2 cells. (d) KEGG pathways with significant enrichment of genes downregulated by TMEM2 overexpression and upregulated by TMEM2 silencing in HepG2 cells. Circle diameter indicates the number of differentially expressed genes and circle color symbolizes the P-value. NC, normal control; TMEM2, transmembrane 2; ShTMEM2, TMEM2 shRNA.
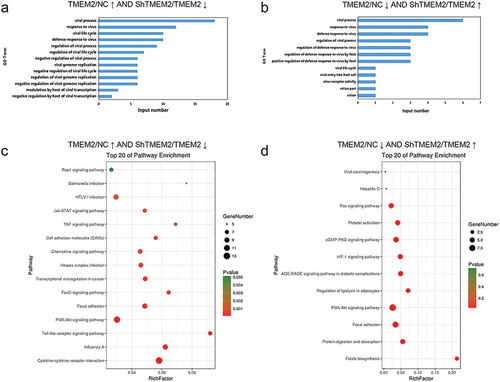
KEGG pathway analysis showed that genes upregulated in HepG2 cells by TMEM2 overexpression and downregulated by TMEM2 shRNA silencing were significantly associated with PI3K-AKT signaling pathway, Rap1 signaling pathway, Salmonella infection, HTLV-I infection, JAK-STAT signaling pathway, TNF signaling pathway, cell adhesion molecules, chemokine signaling, Herpes simplex infection, transcription misregulation in cancers, FoxO signaling pathway, focal adhesion, toll-like receptor signaling pathway, influenza A, and cytokine-cytokine receptor interaction ()). Furthermore, genes downregulated by TMEM2 overexpression but upregulated by TMEM2 silencing were involved in viral carcinogenesis, hepatitis C, Ras signaling pathway, platelet activation, cGMP-PKG signaling pathway, HIF-1 signaling pathway, AGE-RAGE signaling pathway in diabetic complications, regulation of lipolysis in adipocytes, PI3K-AKT signaling pathway, focal adhesion, protein digestion and absorption, and folate biosynthesis ()). These results indicate that the regulation of HBV infection by TMEM2 is mediated by various biological processes and signaling pathways.
Screening and validation of genes involved in PI3K-AKT signaling pathway that is regulated by TMEM2 in HepG2 cells
Based on transcriptome sequencing results, we found that most differentially expressed genes were enriched in the PI3K-AKT signaling pathway. Therefore, we selected a subset of these genes for validation. As shown in , hierarchical clustering has displayed the differentially expressed genes involved in PI3K-AKT signaling pathway in HepG2 cells with TMEM2 knockdown ()) and TMEM2 overexpression ()). The detailed information of these genes is described in . Moreover, RT-qPCR validation results showed that compared with the negative control shRNA (NC) group, shRNA interfering plasmids could inhibit TMEM2 expression, and the sh-TMEM2-2 interference was more effective. The expression of EGFR, spleen tyrosine kinase (SYK), LPAR5, FLT4, AKT3, FLT1, and interleukin (IL)-6 was notably downregulated, consistent with the transcriptome sequencing results. In contrast, CCND2 expression showed no change in TMEM2 knockdown group, whereas IL7R expression was upregulated ()). Furthermore, compared with the control (NC) group, EGFR, SYK, FLT4, CCND2, AKT3, IL7R, FLT1, and IL6 were markedly upregulated, whereas LPAR5 expression showed no change in the TMEM2 overexpression group ()). CCND2 and LPAR5 expression showed no change following TMEM2 knockdown or overexpression group, suggesting there is no direct regulatory relationship between TMEM2 and CCND2 or LPAR5 expression. More experiments are needed to confirm TMEM2 with the specific regulation of these genes. Taken together, we have experimentally validated the gene expression changes of SYK, FLT4, AKT3, FLT1, and IL6 shown by deep sequencing.
Table 2. The expressed PI3K-AKT signaling pathway-related genes in HepG2 cells with TMEM2 overexpression and TMEM2 knockdown.
Figure 3. The expression profile of genes involved in the PI3K-AKT signaling pathway in TMEM2-overexpressed or silenced HepG2 cells. Hierarchical clustering exhibited the up- and down-regulation of genes involved in PI3K-AKT signaling pathway in HepG2 cells following TMEM2 knockdown (a) or overexpression (b).
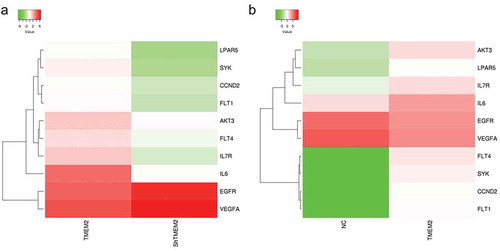
Figure 4. The validation of genes involved in the PI3K-AKT signaling pathway in TMEM2-overexpressed or silenced HepG2 cells. (a–b) The expression levels of genes involved in the PI3K-AKT signaling pathway (TMEM2, EGFR, SYK, LPAR5, FLT4, CCND2, AKT3, IL7R, FLT1, and IL6) were determined by RT-qPCR assay in HepG2 cells transfected with TMEM2 shRNA (sh-TMEM2-1 and sh-TMEM2-2) or TMEM2 overexpression plasmid. *P < 0.05, **P < 0.01.
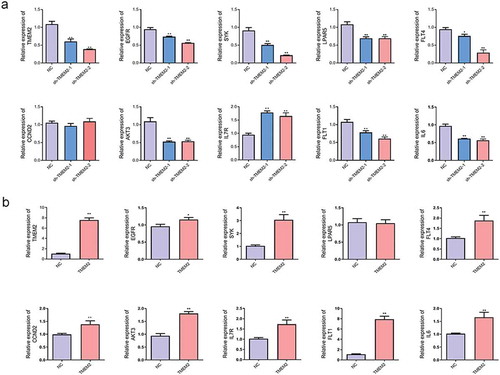
Discussion
The susceptibility of hepatocytes is a critical factor for chronic HBV infection. Recent identification of genetic factors regulating the cellular response of hepatocytes to HBV infection has furthered our understanding of the underlying molecular mechanisms [Citation10,Citation11]. For instance, early studies revealed that the class II cytokine receptor gene cluster is responsible for hepatitis-B persistence [Citation24]. Replication of HBV was previously shown to be facilitated by matrix metalloproteinase 9, which was activated in blood mononuclear cells of patients with HBV infection and in turn inhibited the JAK-STAT signaling pathway [Citation25]. However, the molecular mechanisms underlying the susceptibility of hepatic cells to chronic HBV infection remain poorly understood. We previously demonstrated that the transmembrane protein, TMEM2, inhibits chronic HBV infection via activation of the JAK-STAT signaling pathway in HepG2 cells [Citation17,Citation18]. However, little is known about the cellular processes and signaling pathways regulating TMEM2-mediated cellular responses to chronic HBV infection in hepatocytes. In the present study, we performed high-throughput transcriptome sequencing of HepG2 cells with altered TMEM2 gene expression using overexpression or shRNA-mediated gene silencing.
As expected, transcriptome sequencing analysis revealed a number of genes whose expression levels were significantly altered by TMEM2 gene overexpression or silencing in HepG2 cells. Among them, hundreds of genes that were significantly upregulated by TMEM2 overexpression were downregulated by the silencing of TMEM2 expression. For instance, X-linked cyclin-dependent kinase-like 5 (CDKL5), also known as serological thymidine kinase 9 (STK9), which was previously shown to be a critical regulator of severe neurodevelopmental retardation [Citation26], was greatly increased in HepG2 cells by TMEM2 overexpression, and was markedly suppressed by TMEM2 silencing in HepG2 cells. Conversely, another large group of genes that were downregulated by TMEM2 overexpression were upregulated by the silencing of TMEM2 gene expression. For instance, bone morphogenetic protein 7 (BMP7), which was previously identified as an essential gene associated with developmental defects in skeleton, kidney, and eye [Citation27], was significantly downregulated in HepG2 cells with TMEM2 overexpression, and was upregulated by TMEM2 silencing in HepG2 cells. Consistently, previous reports also demonstrated that BMP7 expression was correlated with hepatic inflammation and fibrosis caused by chronic HBV infection [Citation28,Citation29]. The large number of differentially expressed genes induced by altered TMEM2 gene expression further supports the inhibitory role of TMEM2 in chronic HBV infection in human hepatic cells.
Subsequent bioinformatics analysis revealed significant enrichment of these differentially expressed genes in multiple biological processes associated with viral infection, including multiple signaling pathways such as JAK-STAT and PI3K-AKT pathways. Identification of the JAK/STAT signaling pathway in the present study by transcriptome analysis further confirmed our previous finding that this signaling pathway inhibits chronic HBV [Citation18]. Furthermore, PI3K-AKT pathway plays a critical role in regulating cell proliferation, apoptosis, differentiation, survival, and migration of tumor cells [Citation30–Citation32]. The PI3K/AKT-mTOR signaling pathway regulates HBV replication under certain physiological and pathological conditions [Citation33]. Liu et al. [Citation34] found that HBV large surface antigen promoted tumorigenesis of hepatoma cells by activating the PI3K-AKT pathway. In chronic HBV patients, hepatitis-B core antigen increased programmed death-1 (PD-1) on CD4 + T cells through activation of PI3K-AKT pathway [Citation35]. Therefore, PI3K-AKT signaling pathway may be closely related to HBV infection. In our study, we have shown that overexpression and knockdown of TMEM2 lead to expression changes of genes involved in PI3K-Akt signaling pathway, including SYK, FLT4, AKT3, FLT1, and IL6. SYK is a cytoplasmic nonreceptor tyrosine kinase that is involved in HCC. Qu et al. [Citation36] found that HBV infection significantly increased SYK level in hepatocytes and upregulated SYK reduced HCC development. Hashimoto et al. [Citation37] revealed that AKT3 gene was upregulated in both early and advanced stages of HCC and may be involved in the development of hepatitis C virus (HCV)–HCC. In Chang’s study [Citation38], they showed that activation of IL6/IGFIR induced poor prognosis of HBV-HCC. The identification of the genes involved in PI3K-Akt signaling pathway has provided new information on TMEM2 function in viral infection and other processes.
Conclusions
In summary, transcriptome sequencing revealed that overexpression or silencing of TMEM2 gene led to gene expression changes of many genes in HepG2 cells. These alterations were associated with various biological processes and signaling pathways. Our study is the first in demonstrating the expression changes of genes involved in the PI3K-AKT signaling pathway (SYK, FLT4, AKT3, FLT1, IL6), that were previously implicated in the pathogenesis of HCC. Therefore, our study has provided new insight into the regulation of host cell susceptibility to chronic HBV infection by TMEM2 gene expression. In future studies, functions of many of these genes identified in our study need to be further explored in HCC.
Authors’ contributions
XZ and LP designed the study and wrote the draft. XHJ, ZSM, QYZ, TCB, WXX, and ZLG conducted the experiments, collected and analyzed the data. XZ and LP critically revised the manuscript. All authors read and approved the final manuscript.
Disclosure statement
No potential conflict of interest was reported by the authors.
Additional information
Funding
References
- Seto WK, Lo YR, Pawlotsky JM, et al. Chronic hepatitis B virus infection. Lancet. 2018;392(10161):2313–2324. PubMed PMID: 30496122.
- Tang LSY, Covert E, Wilson E, et al. Chronic hepatitis B infection: a review. JAMA. 2018;319(17):1802–1813. PubMed PMID: 29715359.
- Hutin Y, Nasrullah M, Easterbrook P, et al. Access to treatment for hepatitis B virus infection - worldwide, 2016. MMWR Morb Mortal Wkly Rep. 2018;67(28):773–777. PubMed PMID: 30025413.
- Lancet, The. Towards elimination of viral hepatitis by 2030. Lancet. 2016;388(10042):308. PubMed PMID: 27477148.
- Morikawa K, Suda G, Sakamoto N. Viral life cycle of hepatitis B virus: host factors and druggable targets. Hepatol Res. 2016;46(9):871–877. PubMed PMID: 26776362.
- Nishitsuji H, Ujino S, Shimizu Y, et al. Novel reporter system to monitor early stages of the hepatitis B virus life cycle. Cancer Sci. 2015;106(11):1616–1624. PubMed PMID: 26310603.
- Boeijen LL, Hoogeveen RC, Boonstra A, et al. Hepatitis B virus infection and the immune response: the big questions. Best Pract Res Clin Gastroenterol. 2017;31(3):265–272. PubMed PMID: 28774408.
- Tsai KN, Kuo CF, Ou JJ. Mechanisms of hepatitis B virus persistence. Trends Microbiol. 2018;26(1):33–42. PubMed PMID: 28823759.
- He Q, Huang Y, Zhang L, et al. Association between vitamin D receptor polymorphisms and hepatitis B virus infection susceptibility: a meta-analysis study. Gene. 2018;645:105–112. PubMed PMID: 29248582.
- Kacprzak-Bergman I, Nowakowska B. Influence of genetic factors on the susceptibility to HBV infection, its clinical pictures, and responsiveness to HBV vaccination. Arch Immunol Ther Exp (Warsz). 2005;53(2):139–142. PubMed PMID: 15928582.
- Moudi B, Heidari Z, Mahmoudzadeh-Sagheb H. Impact of host gene polymorphisms on susceptibility to chronic hepatitis B virus infection. Infect Genet Evol. 2016;44:94–105. PubMed PMID: 27346643.
- Wrzesiński T, Szelag M, Cieślikowski WA, et al. Expression of pre-selected TMEMs with predicted ER localization as potential classifiers of ccRCC tumors. BMC Cancer. 2015;15:518. 10.1186/s12885-015-1530-4. PubMed PMID: 26169495
- Totong R, Schell T, Lescroart F, et al. The novel transmembrane protein Tmem2 is essential for coordination of myocardial and endocardial morphogenesis. Development. 2011;138(19):4199–4205. PubMed PMID: 21896630.
- Smith KA, Lagendijk AK, Courtney AD, et al. Transmembrane protein 2 (Tmem2) is required to regionally restrict atrioventricular canal boundary and endocardial cushion development. Development. 2011;138(19):4193–4198. PubMed PMID: 21896629.
- Yamaguchi Y, Yamamoto H, Tobisawa Y, et al. TMEM2: a missing link in hyaluronan catabolism identified? Matrix Biol. 2019 May;78–79:139–146. PubMed PMID: 29601864; PubMed Central PMCID: PMCPMC6314907. eng.
- Yamamoto H, Tobisawa Y, Inubushi T, et al. A mammalian homolog of the zebrafish transmembrane protein 2 (TMEM2) is the long-sought-after cell-surface hyaluronidase. J Biol Chem. 2017;292(18):7304–7313. PubMed PMID: 28246172.
- Zhao Q, Peng L, Huang W, et al. Rare inborn errors associated with chronic hepatitis B virus infection. Hepatology. 2012;56(5):1661–1670. PubMed PMID: 22610944.
- Zhu X, Xie C, Li YM, et al. TMEM2 inhibits hepatitis B virus infection in HepG2 and HepG2.2.15 cells by activating the JAK-STAT signaling pathway. Cell Death Dis. 2016;7(6):e2239. PubMed PMID: 27253403.
- Li R, Yu C, Li Y, et al. SOAP2: an improved ultrafast tool for short read alignment. Bioinformatics. 2009;25(15):1966–1967. PubMed PMID: 19497933.
- Mortazavi A, Williams BA, McCue K, et al. Mapping and quantifying mammalian transcriptomes by RNA-Seq. Nat Methods. 2008;5(7):621–628. PubMed PMID: 18516045.
- Eisen MB, Spellman PT, Brown PO, et al. Cluster analysis and display of genome-wide expression patterns. Proc Natl Acad Sci U S A. 1998;95(25):14863–14868. PubMed PMID: 9843981.
- Kanehisa M, Araki M, Goto S, et al. KEGG for linking genomes to life and the environment. Nucleic Acids Res. 2008;36:D480–4. PubMed PMID: 18077471.
- Livak KJ, Schmittgen TD. Analysis of relative gene expression data using real-time quantitative PCR and the 2(-Delta Delta C(T)) method. Methods. 2001 Dec;25(4):402–408. PubMed PMID: 11846609; eng.
- Frodsham AJ, Zhang L, Dumpis U, et al. Class II cytokine receptor gene cluster is a major locus for hepatitis B persistence. Proc Natl Acad Sci U S A. 2006 Jun 13;103(24):9148–9153. PubMed PMID: 16757563; PubMed Central PMCID: PMCPMC1482581. eng.
- Chen J, Xu W, Chen Y, et al. Matrix metalloproteinase 9 facilitates hepatitis B virus replication through binding with type I interferon (IFN) receptor 1 to repress IFN/JAK/STAT signaling. J Virol. 2017 Apr 15;91(8). PubMed PMID: 28122987; PubMed Central PMCID: PMCPMC5375666. eng. DOI:10.1128/jvi.01824-16.
- Tao J, Van Esch H, Hagedorn-Greiwe M, et al. Mutations in the X-linked cyclin-dependent kinase-like 5 (CDKL5/STK9) gene are associated with severe neurodevelopmental retardation. Am J Hum Genet. 2004 Dec;75(6):1149–1154. PubMed PMID: 15499549; PubMed Central PMCID: PMCPMC1182152. eng.
- Jena N, Martín-Seisdedos C, McCue P, et al. BMP7 null mutation in mice: developmental defects in skeleton, kidney, and eye. Exp Cell Res. 1997 Jan 10;230(1):28–37. PubMed PMID: 9013703; eng.
- Tang CL, Zhou Z, Shi WQ. [Effects of Fuzheng Huayu Capsule on the ratio of TGF-beta1/BMP-7 of chronic viral hepatitis B fibrosis patients of Gan-Shen insufficiency blood-stasis obstruction syndrome]. Zhongguo Zhong xi yi jie he za zhi Zhongguo Zhongxiyi jiehe zazhi = Chinese journal of integrated traditional and Western medicine. 2012 Jan;32(1):20–24. PubMed PMID: 22500384; chi.
- Cao H, Shu X, Chen LB et al., [The relationship of expression of BMP-7 in the liver and hepatic inflammation and fibrosis in patients with chronic HBV infection]. Zhonghua shi yan he lin chuang bing du xue za zhi = Zhonghua shiyan he linchuang bingduxue zazhi = Chinese journal of experimental and clinical virology. 2010 Apr;24(2):101–103. PubMed PMID: 21110425; chi.
- Alzahrani AS PI3K/Akt/mTOR inhibitors in cancer: at the bench and bedside. Semin Cancer Biol. 2019 Dec;59:125–132. PubMed PMID: 31323288; eng.
- Aoki M, Fujishita T. Oncogenic roles of the PI3K/AKT/mTOR axis. Curr Top Microbiol Immunol. 2017;407:153–189. PubMed PMID: 28550454; eng.
- Ersahin T, Tuncbag N, Cetin-Atalay R. The PI3K/AKT/mTOR interactive pathway. Mol Biosyst. 2015 Jul;11(7):1946–1954. PubMed PMID: 25924008; eng.
- Guo H, Zhou T, Jiang D, et al. Regulation of hepatitis B virus replication by the phosphatidylinositol 3-kinase-akt signal transduction pathway. J Virol. 2007 Sep;81(18):10072–10080. PubMed PMID: 17609269; PubMed Central PMCID: PMCPMC2045390. eng.
- Liu H, Xu J, Zhou L, et al. Hepatitis B virus large surface antigen promotes liver carcinogenesis by activating the Src/PI3K/Akt pathway. Cancer Res. 2011;71(24):7547–7557.
- Li M, Sun X-H, Zhu X-J, et al. HBcAg induces PD-1 upregulation on CD4+ T cells through activation of JNK, ERK and PI3K/AKT pathways in chronic hepatitis-B-infected patients. Lab Invest. 2012;92(2):295.
- Qu C, Zheng D, Li S, et al. Tyrosine kinase SYK is a potential therapeutic target for liver fibrosis. Hepatology. 2018;68(3):1125–1139. PubMed PMID: 29537660.
- Hashimoto K, Mori N, Tamesa T, et al. Analysis of DNA copy number aberrations in hepatitis C virus-associated hepatocellular carcinomas by conventional CGH and array CGH. Mod Pathol. 2004;17(6):617–622. PubMed PMID: 15133472.
- Chang TS, Wu YC, Chi CC, et al. Activation of IL6/IGFIR confers poor prognosis of HBV-related hepatocellular carcinoma through induction of OCT4/NANOG expression. Clin Cancer Res off J Am Assoc Cancer Res. 2015;21(1):201–210. PubMed PMID: 25564572.