ABSTRACT
The cAMP-dependent protein kinase Pka1 is known as a regulator of glycogenesis, transition into meiosis, proper chromosome segregation, and stress responses in Schizosaccharomyces pombe. We demonstrated that both the cAMP/PKA pathway and glucose limitation play roles in appropriate spindle formation. Overexpression of Mal3 (1–308), an EB1 family protein, caused growth defects, increased 4C DNA content, and induced monopolar spindle formation. Overproduction of a high-affinity microtubule binding mutant (Q89R) and a recombinant protein possessing the CH and EB1 domains (1–241) both resulted in more severe phenotypes than Mal3 (1–308). Loss of functional Pka1 and glucose limitation rescued the phenotypes of Mal3-overexpressing cells, whereas deletion of Tor1 or Ssp2 did not. Growth defects and monopolar spindle formation in a kinesin-5 mutant, cut7-446, was partially rescued by pka1 deletion or glucose limitation. These findings suggest that Pka1 and glucose limitation regulate proper spindle formation in Mal3-overexpressing cells and the cut7-446 mutant.
Graphical abstract
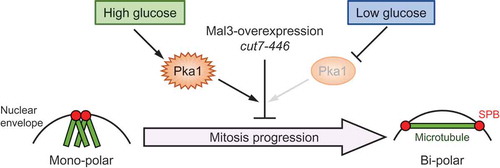
The loss of functional Pka1 or glucose limitation rescues monopolar spindle formation and the growth defects in Mal3-overexpressing cells and the cut7-446 mutant.
The EB1 family of proteins, commonly known as microtubule-associated proteins (MAPs), is conserved in eukaryotes ranging from yeast to human. EB1 proteins have several important roles in cellular functions, such as in promotion of microtubule polymerization, regulation of cell polarity, and stabilization of proper chromosome segregation [Citation1,Citation2]. In the fission yeast, Schizosac-charomyces pombe, EB1 protein Mal3 mainly regulates cell polarity by maintaining proper formation of microtubules and actin during interphase, and also by ensuring appropriate chromosome segregation by regulating microtubule-chromosome attachment in mitosis [Citation1,Citation3,Citation4]. Mal3 interacts with several proteins, including CLIP-170/Tip1 and kinesin/Tea2 in interphase, and blinkin/Spc7 and TOG/Dis1 in mitosis [Citation5–Citation7]. However, details regarding the mitotic function of Mal3 are mostly unclear. Mal3 has two major domains: the Calponin homology (CH) domain at the N-terminus and the end-binding (EB1) domain at the C-terminus [Citation8,Citation9]. The CH domain promotes microtubule polymerization, but its underlying mechanism is also unclear [Citation10]. The EB1 domain mediates interactions between binding partners, such as EB1/Mal3, CLIP-170/Tip1, and CLASP1/Peg1 [Citation2]. Two Mal3 mutants, Mal3 (K63D) and Mal3 (Q89E), that fail to bind microtubules in vitro have been isolated [Citation11], and another, Mal3 mutant (Q89R), was found to have excessive binding activity to microtubules, both in vivo and in vitro [Citation11,Citation12].
The kinesin-5 family of proteins is responsible for establishing bipolar spindles in many organisms [Citation13–Citation18], and inhibition of kinesin-5 activity results in monopolar spindle formation and chromosome mis-segregation [Citation18]. In S. pombe, kinesin-5 is encoded by the cut7 gene, which is essential gene for growth [Citation16]. A temperature sensitive cut7-446 mutant strain exhibits growth defects, abnormal chromosome segregation (the cut phenotype), and monopolar spindle formation at high temperatures [Citation16,Citation19,Citation20]. Notably, mal3∆ strains rescued the temperature sensitive phenotype of the cut7-22 mutant [Citation21].
The cAMP/PKA pathway is known as a major glucose response pathway in yeasts [Citation22–Citation25]. In S. pombe, the cAMP/PKA pathway consists of the G protein-coupled receptor Git3, as well as a heterotrimeric G protein alpha subunit (Gpa2), a beta subunit (Git5), a gamma subunit (Git11), an adenylate cyclase (Cyr1), a protein kinase A regulatory subunit (Cgs1), and a protein kinase A catalytic subunit (Pka1) [Citation26–Citation32]. This pathway has roles in glucose-sensing, regulation of transition to meiosis, chronological aging, stress response, and regulation of proper chromosome segregation [Citation29–Citation31,Citation33-Citation37]. We have recently reported that the pka1∆ strains exhibit a TBZ-sensitive phenotype and chromosome mis-segregation. These phenotypes were suppressed by overexpression of Mal3, and the ability of Mal3 to bind microtubules was an important part of these effects [Citation36]. We have also shown that deletion of pka1 rescued growth defects caused by Mal3-overexpression in the wild-type strain, but detailed mechanistic analysis has not been conducted [Citation36].
In this study, we showed that Mal3 overexpression induced monopolar spindle formation in addition to the growth inhibition, and these effects were suppressed by the deletion of pka1 and by glucose limitation. We also showed that deletion of pka1 and glucose limitation rescued the temperature sensitive phenotype and monopolar spindle formation in the cut7-446 mutant. As glucose limitation masks Pka1 activity by the formation of the Pka1-Cgs1 complex [Citation38], the glucose-Pka1 signaling pathway somehow controls microtubule organization. This likely occurs either through direct or indirect involvement of a microtubule-associated protein, such as Mal3.
Materials and methods
Yeast strains, media, and genetic methods
The S. pombe strains used in this study are listed in supplementary Table 1. Standard yeast culture media and genetic methods were used [Citation39,Citation40]. S. pombe cultures were grown in either YES medium (0.5% yeast extract, 3% glucose, 225 mg/L adenine, 225 mg/L uracil, 225 mg/L leucine, 225 mg/L histidine, and 225 mg/L lysine), YES glucose-limited medium (0.5% yeast extract, 0.1% glucose, 3% glycerol, 225 mg/L adenine, 225 mg/L uracil, 225 mg/L leucine, 225 mg/L histidine, and 225 mg/L lysine), or synthetic minimal medium (EMM) with appropriate auxotrophic supplements [Citation40].
Mutant and transgenic strains (TTP233: h− tor1∆, YMP324: h− cut7-446, YMP351: h− pka1∆ cut7-446, TTP157: h− cut7-446 GFP-atb2 sad1-mRFP, TTP171: h+ mal3∆ GFP-atb2 sad1-mRFP, and TTP277: h− pka1∆ cut7-446 GFP-atb2 sad1-mRFP) were constructed by genetic crossing and selection with markers using standard yeast genetics techniques [Citation41].
Plasmid construction and induction of expression under the nmt1 promoter
To construct pREP3X-Mal3 (135–241), the oligonucleotide primers MAL3-135BF (5ʹ-TATGGATCCTCATGACTGGCCCTTCTCGTCGCCGTC-3ʹ) and MAL3-241SSmR (5ʹ-ACAGTCGACCCGGGTTAAGTAGAATAAAGTATTGCTTG-3ʹ) were used to amplify a 340 bp fragment from pREP3X-Mal3 [Citation36]. The amplified mal3 (135–241) fragment was digested with BamHI and SmaI and ligated into corresponding sites in pREP3X [Citation42] to generate the plasmid pREP3X-Mal3 (135–241).
Construction of pREP3X-Mal3 (1–241 Q89E) was performed according to the method described previously [Citation36] using pREP3X-Mal3 (1–241) as a template. To analyze whether the level of Mal3 expression affected phenotypes, pREP41X-mal3, containing the nmt41 promoter rather than the nmt1 promoter, was constructed using the restriction enzymes BamHI and SmaI from pREP3X-Mal3 and pREP41X [Citation42]. Other plasmids used were described previously [Citation36].
Wild-type, mal3∆, pka1∆, tor1∆, and ssp2∆ cells were transformed with the pREP3X-derived plasmids (LEU2 marker) and transformants were selected onto EMMU (EMM containing uracil but lacking leucine) plates containing 15 µM thiamine to repress gene expression from the nmt1 promoter. To test the growth following mal3 overexpression, transformants were grown on EMMU containing 15 µM thiamine for 2 days at 30°C, and were then transferred onto EMMU without thiamine, to induce mal3 expression from the nmt1 promoter, and incubated for 1 day at 30°C. Cells were then spotted on EMMU plates in the presence or absence of 15 µM thiamine and incubated for 3 to 5 days at 30°C.
When mal3 expression was induced from the nmt1 promoter, cells were first grown in EMM containing 15 µM thiamine to mid-log phase (~5 x 106 cells/mL) at 30°C. The cells were then washed three times with water and resuspended in EMM lacking thiamine and incubated for an additional 24 h at 30°C.
Fluorescence microscopy of GFP or mRFP fusion protein
S. pombe cells were grown in EMMU liquid medium containing 15 µM thiamine, washed three times with water, transferred to EMMU liquid medium lacking thiamine, and incubated for 24 h at 30°C. GFP-tagged Atb2 and mRFP-tagged Sad1 proteins in living cells were visualized and imaged using a BX51 microscope (Olympus) equipped with a DP74 digital camera (Olympus) or on a BZ-X700 microscope (Keyence).
Fluorescence-activated cell sorting (FACS) of DNA contents
Cells were cultured to mid-log phase in EMMU containing 15 µM thiamine at 30°C, washed three times with water, resuspended in EMMU without thiamine, and cultured for 24 h at 30°C. Cells were then fixed for 1 day on ice using 70% ethanol and washed with 50 mM sodium citrate. Fixed cells were suspended into 0.1 mL of 50 mM sodium citrate containing 1 mg/mL RNase A and incubated for 3 h at 37°C. After RNA degradation, the suspended cells were stained with 2 µg/mL propidium iodide. Stained cells were analyzed using a FACSCalibur flow-cytometer (Becton-Dickinson).
Preparation of cell lysates and detection of Mal3 protein by immunoblotting
S. pombe cell lysates were prepared as previously described [Citation43]. Protein lysates were separated by SDS-PAGE, after which western blot analysis was performed using an ECL detection system (GE Healthcare) according to the supplier’s instructions. Rabbit polyclonal anti-Mal3 (diluted 1:1000) antibody was provided by Drs. M. Iimori (Kyushu University) and T. Matsumoto (Kyoto University). Rabbit polyclonal anti-PSTAIRE (Cdc2; diluted 1:1000) antibody was purchased from Santa Cruz Biotechnology. Horseradish peroxidase-conjugated anti-rabbit IgG antibody (Promega) was used as the secondary antibody.
Data and statistics
Experiments were performed in triplicate and the average values and standard deviation (SD) were calculated. Data from controls and cells overexpressing Mal3 were compared using a two-sample t-test. P-values <0.05 were considered statistically significant. All statistical analyses were performed using EZR version 3.61 (Saitama Medical Center, Jichi Medical University, Saitama, Japan) [Citation44], which is a graphical user interface for R (The R Foundation for Statistical Computing, Vienna, Austria).
Reproducibility
Experiments were conducted at least twice to confirm the reproducibility of the results.
Results
Overexpression of Mal3 induces monopolar spindle formation
Overexpression of Mal3 causes growth defects in wild-type cells [Citation3]. To confirm this phenotype, wild-type cells were transformed with pREP3x (empty vector) or pREP3x-mal3. Mal3-overexpressing cells exhibited growth defects ()) as described previously [Citation3]. FACS analysis of Mal3-overexpressing cells showed that the number of cells with mitotic defects, as indicated by the presence of 4C DNA content and chromosome mis-segregation, was significantly increased (). Since Mal3-overexpression caused mitotic defects, we next observed microtubules, by GFP-Atb2, and spindle pole bodies (SPB), by Sad1-mRFP. Around 25% of Mal3-overexpressing cells formed monopolar spindles, observed as a V-shape (). We confirmed that mal3-overexpression did not significantly affect the protein expression level of α-tubulin (supplementary Figure 1). We then used the nmt41 promoter, which is a weaker promoter than the nmt1 promoter, to govern the expression of Mal3 to test monopolar spindle formation. Mal3-overexpression under the nmt41 promoter showed normal spindle formation, although cells were elongated (supplementary Figure 2). Therefore, only a high level of Mal3 expression caused the monopolar spindle formation.
Figure 1. Overexpression of Mal3 causes a growth defect, accumulation of 4C DNA, and monopolar spindle formation. (a) Wild-type (PR109) cells harboring pREP3X (vector) or pREP3X-mal3 (1–308; full-length) were cultured for 1 day on EMMU containing 15 µM thiamine at 30°C to repress expression of the mal3 gene from the nmt1 promoter. Cells were spotted onto EMMU (2% glucose) in the presence or absence of 15 µM thiamine. All plates were incubated for 4 days at 30°C. (b) FACS profile of cells overexpressing Mal3. Cells were fixed and stained with propidium iodide. (c) Cells were stained with DAPI to visualize the nuclei and septum. Red arrowheads indicate chromosome mis-segregation. Scale bar: 10 µm. (d) A GFP-Atb2 (green color) and Sad1-mRFP (red color) expressing strain (TTP76) harboring pREP3X (vector) or pREP3X-mal3 (1–308; full-length); Atb2 localizes to microtubules and Sad1 localizes to spindle pole body which is similar to the centrosome in mammals. Sad1-mRFP was used to visualize the end of microtubules. Arrowheads indicate monopolar spindles. Scale bar: 10 µm. (e) Enumeration of monopolar spindle formation in the transformants. Approximately 200 cells were analyzed in each strain. Experiments were performed three times; averages with S.D. are shown.
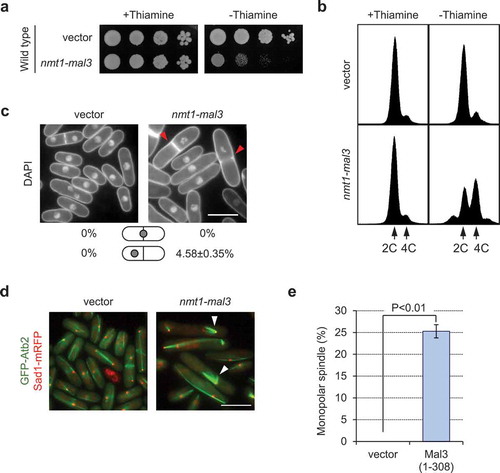
Overexpression of the Mal3 CH with the EB1 domain causes monopolar spindle formation
Since Mal3 has two major domains, namely, the Calponin homology (CH) domain for microtubule binding at the N-terminus (3–103) and the end-binding (EB1) domain for binding with other proteins at the C-terminus (197–241), we analyzed which domain caused growth defects and monopolar spindle formation in Mal3-overexpressing cells. To do so, we used five plasmids that included different portions of the fragmented Mal3 (supplementary Figure 3a and [Citation36]). The mal3∆ mutant was transformed with each plasmid, incubated in the absence of thiamine to induce the mal3 expression, and cells were spotted onto EMMU in the presence or absence of thiamine. Overexpression of Mal3 (1–308) or Mal3 (1–241) resulted in growth defects, whereas Mal3 (1–197), Mal3 (135–241), and Mal3 (135–308) showed normal growth ()). FACS analysis of Mal3 (1–308) and Mal3 (1–241) overexpressing cells showed 4C DNA content ()) and a substantial frequency of monopolar spindles was noted in microscopic analysis (). The protein expression levels of the Mal3 fragments were not different from the full length Mal3 (supplementary Figure 3b). These results suggest that the combination of the Mal3 CH and the EB1 domains is responsible for defective growth and monopolar spindle formation in the Mal3-overexpressing cells.
Figure 2. Overexpression of the CH and EB1 domain of Mal3 causes growth defects, accumulation of 4C DNA content, and monopolar spindle formation.
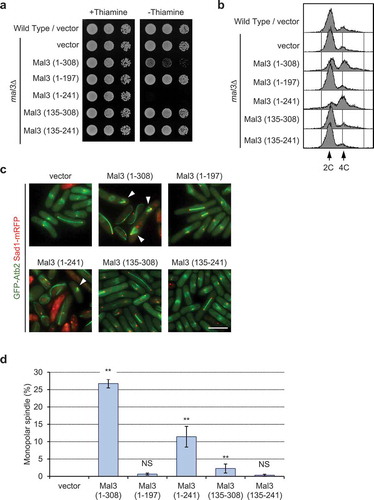
Microtubule binding by the Mal3 CH domain is required for monopolar spindle formation
We next investigated whether microtubule binding ability of Mal3 is responsible for the observed growth defects and monopolar spindle formation using the Mal3 (Q89R) and Mal3 (Q89E) mutant. The Mal3 (Q89R) mutant strongly binds to the microtubules but the Mal3 (Q89E) mutant does not [Citation11,Citation12]. Overexpression of the Mal3 (Q89R) mutant resulted in growth defects, whereas overexpression of the Mal3 (Q89E) mutant did not affect growth (). Interestingly, the Q89E mutation in Mal3 (1–241) abolished the defective growth phenotype ()), indicating that microtubule binding is important for causing the growth defects in Mal3-overexpressing cells. We also noted that the Mal3 (Q89R) mutant had an increased amount of 4 C DNA cells than Mal3 (1–308), and a substantial frequency of monopolar spindle formation, similar to Mal3 (1–308) (). Because a point mutation in Mal3 (Q89E) does not completely abolish microtubule binding by the CH domain, the Mal3 (Q89E) mutant showed a lower, but nonzero, frequency of monopolar spindle formation ()). The Q89E mutation in Mal3 (1–241) also resulted in reduced 4C DNA contents and monopolar spindle formation (). We confirmed protein levels of Mal3 and its derivatives were not different among Mal3 (1–308), Mal3 (1–241), Mal3 (Q89R), and Mal3 (Q89E) ()). These results suggest that microtubule binding by the CH domain contributes to the defective growth phenotype and to monopolar spindle formation in Mal3-overexpressing cells.
Figure 3. Microtubule binding of Mal3 causes growth defects, accumulation of 4C DNA, and monopolar spindle formation.
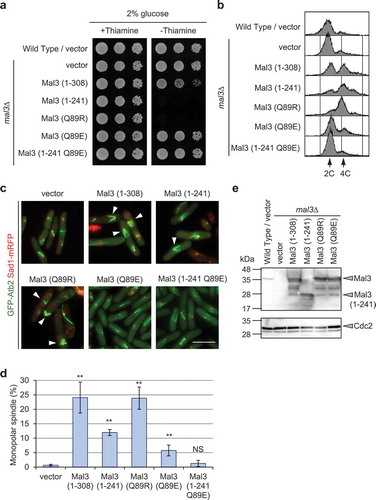
Mal3 (1-241) and Mal3 (Q89R) reduce cell viability and cause high ratio of monopolar spindle formation at early time point
Since the frequency of monopolar spindle formation was higher in Mal3 (1–308) and Mal3 (Q89R) expressed cells than Mal3 (1–241) expressed cells ()), we carefully examined the timing of cell viability loss and monopolar spindle formation in Mal3 (1–308), Mal3 (1–241) and Mal3 (Q89R) overexpressed cells. Overexpression of the Mal3 (1–241) and Mal3 (Q89R) mutants reduced cell viability at the earlier point than overexpression of Mal3 (1–308) ()). Monopolar spindle formation was substantially high (34-48%) at 18 h in Mal3 (1–241) and Mal3 (Q89R) overexpressed cells, then decreased after 24 h, whereas overexpression of Mal3 (1–308) remained at substantially high frequency (28%) at 24 h, then decreased at 30 h ()). Thus, expression of Mal3 (1–241) and Mal3 (Q89R) strongly induced monopolar spindle formation at the early stage.
Figure 4. Mal3 (1–241) and Mal3 (Q89R) immediately induce monopolar spindle formation.
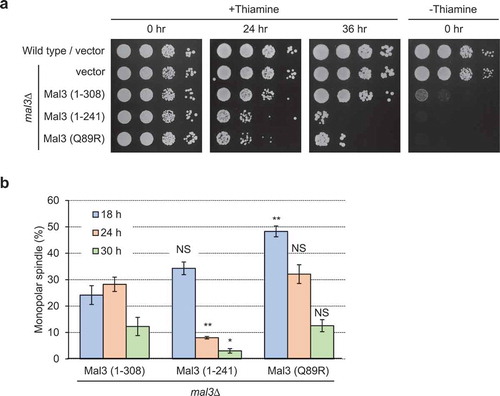
Loss of functional PKA rescues growth defects and monopolar spindle formation
We have previously reported that overexpression of Mal3 rescues the TBZ-sensitive phenotype of the pka1∆ mutant [Citation36]. We have also shown that the pka1∆ mutant grows normally when overexpressing Mal3 (1–308; full-length), but showed growth defects from Mal3 (1–241) or Mal3 (Q89R)-overexpression [Citation36]. To test the relevance of Pka1 in the phenotypes observed in this current study, the pka1∆ mutant harboring pREP3X or pREP3x-mal3 was spotted onto EMMU plates and the DNA contents of each transformant in the absence of thiamine was analyzed. The pka1∆ mutant did not exhibit the defective growth phenotype and had lower 4C DNA contents in Mal3-overexpressing cells than were observed in wild-type cells (). We also analyzed the phenotypes in cyr1∆ and cgs1∆ strains because deletion of cyr1 results in Pka1 inactivation and deletion of cgs1 results in constitutive activation of Pka1 [Citation29–Citation31,Citation33-Citation37]. We found that the cyr1∆ strain rescued the growth defects in Mal3-overexpressing cells but the cgs1∆ strain showed more severe growth defects (supplementary Figure 4), indicating that Pka1 activity is highly related to the suppressive effects of defective growth in Mal3-overexpressing cells. The frequency of monopolar spindle was significantly decreased in the pka1∆ mutant (), further suggesting that Pka1 is involved in the regulation of appropriate mitotic spindle formation.
Figure 5. The pka1∆ strain rescues growth defects, accumulation of 4C DNA, and monopolar spindle formation in Mal3-overexpressing cells.
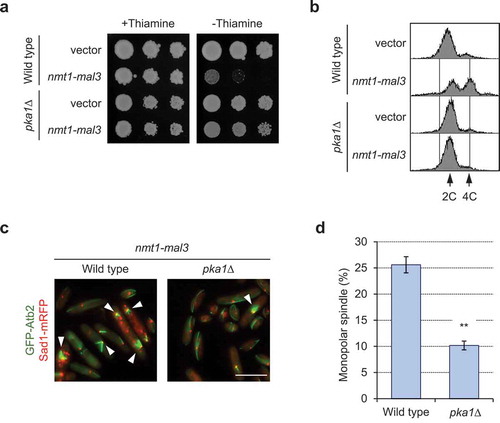
Glucose limitation rescues growth defects and abnormal spindle formation
Since the cAMP/PKA pathway is a known glucose response pathway, we next investigated whether glucose concentration affected growth defects and monopolar spindle formation in Mal3-overexpressing cells. Mal3 (1–308; full-length)-overexpressing cells grew normally on glucose-limited medium (0.1% glucose) ()), notably different from the defective growth observed on normal glucose medium (3% glucose) ()). However, cell growth on glucose-limited medium was inhibited in Mal3 (1-241)- or Mal3 (Q89R)-overexpressing cells ()), which had more severe phenotype compared to that of Mal3 (1–308). We next analyzed the DNA content of Mal3-overexpressed cells under glucose-limited conditions (0.1% glucose). Accumulation of 4 C DNA in Mal3 (1–308; full-length)-, Mal3 (1-241)-, and Mal3 (Q89R)-overexpressing cells ()) was reduced under glucose-limiting conditions (0.1% glucose). The monopolar spindle formation phenotype induced by Mal3 (1–308; full-length) was rescued under glucose-limiting conditions, whereas the same phenotype was not rescued by glucose limitation in Mal3 (1–241) or Mal3 (Q89R) expressing cells (). These results indicate that glucose limitations partially antagonize the growth defect and monopolar spindle formation in Mal3-overexpressed cells.
Figure 6. Glucose limitation rescues the Mal3-overexpression phenotype.
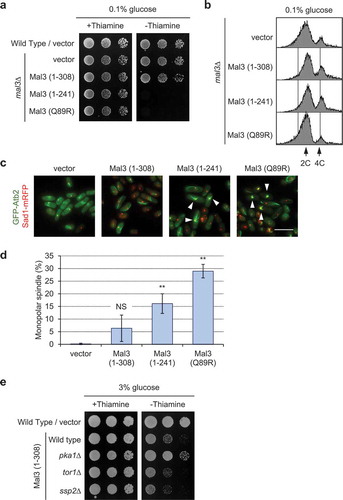
Because the TORC2 and AMPK pathways are also glucose response pathways in S. pombe [Citation45,Citation46], we next investigated whether a tor1∆ or ssp2∆ strain rescues defective growth in Mal3-overexpressing cells. The growth defects caused by Mal3-overexpression were not rescued in the tor1∆ and ssp2∆ strains under normal glucose conditions (3% glucose) ()), indicating that only the cAMP/PKA pathway is regulating the growth and monopolar spindle formation in Mal3-overexpressing cells.
The cut7-446 mutant is rescued by loss of functional PKA and glucose limitations
The cut7-446 mutant has previously been shown to exhibit monopolar spindle formation [Citation16] as was observed in Mal3-overexpressing cells, and the temperature sensitive phenotype in cut7-22 was rescued by mal3 deletion [Citation21]. Based on these observations, we investigated genetic interactions between the cut7-446 mutant and the pka1∆ strain and the effects of glucose limitation. The temperature sensitive phenotype of the cut7-446 mutant was partially rescued at 30°C by pka1 deletion ()) and by glucose-limitation ()). Monopolar spindle formation was also abolished by pka1 deletion and glucose limitation at semi-restriction temperature (30°C) in the cut7-446 mutant (). The cut7-446 mutant showed higher DNA contents than 4C by FACS analysis (supplementary Figure 5a). However, the cut7-446 mutant exhibited monopolar spindle formation at a restrictive temperature, 37°C, from deletion of pka1 or limited glucose (supplementary Figure 5b). Thus, loss of functional Pka1 and glucose limitation partially rescues growth defects and monopolar spindle formation in the cut7-446 mutant.
Figure 7. The phenotypes of the cut7-446 mutant are rescued by loss of functional Pka1 and under glucose-limiting conditions.
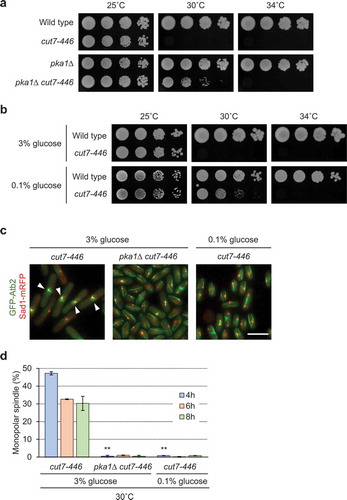
Discussion
In this study, we investigated the role of Mal3 in mitosis by observing the cellular effects of Mal3 overexpression. Overexpression of Mal3 resulted in a specific growth defect, increased 4C DNA contents, chromosome mis-segregation, and monopolar spindle formation () as has been described previously [Citation3]. Although microtubule dynamics are reportedly affected in Mal3-overexpressing cells [Citation3], the detailed molecular mechanism underlying this has not been described. Our findings in this study showed that overexpression of the CH domain (1–197) or the EB1 domain (135–308) alone did not result in defective growth or elevated monopolar spindle formation, and that the phenotypes caused by overexpression of Mal3 or Mal3 (1–241) were abolished by a mutation (Q89E) that renders the Mal3 protein unable to bind microtubules (). It has been shown that the CH domain contributes to microtubule binding and that the EB1 domain is involved in microtubule stability, proper chromosome segregation, and interactions with binding partners, such as Dis1/TOG, Tip1/CLIP-170, and Peg1/CLASP [Citation5–Citation7,Citation11,Citation12,Citation36,Citation47–Citation49]. Based on these observations, results from our current study suggest that the EB1 domain is responsible for monopolar spindle formation when Mal3 binds to microtubules through the CH domain. Bipolar spindle formation might be controlled by microtubule dynamics or Mal3 binding partners mediated through the EB1 domain. One such binding partner, Klp2, causes monopolar spindle formation upon Klp2 overexpression [Citation50]. Overexpression of Mal3 possibly recruits excess Klp2 around microtubules, which results in monopolar spindle formation similar to that observed from overexpression of Klp2. However, we observed that mal3 deletion did not rescue the growth defect caused by Klp2-overexpression and conversely klp2 deletion did not rescue the growth defect caused by Mal3-overexpression (data not shown). These observations indicate that the growth inhibitory effect of Mal3-overexpression does not depend on Klp2 and suggest that Mal3-overexpression might cause monopolar spindle formation by regulating microtubule dynamics or through the effect of yet unidentified binding partner(s). In our findings, overexpression of a truncated Mal3 (1–241) exhibited more severe phenotype compared to the full-length Mal3 (1–308), consistent with our previous report [Citation36]. These results suggest that the 241–308 region of Mal3 is required for proper chromosome segregation and might be responsible for viability or suppression of monopolar spindle formation. The EB1 family protein has an EEY/F motif at the tail, which is the binding site of the proteins harboring a CAP-glycine motif (GKNDG). S. pombe Mal3 has an ITF amino acid sequence at the tail which is thought to have a function similar to that of the EEY/F motif [Citation51]. S. pombe has at least 3 proteins including Tip1 (CLIP-170), Ssm4 (dynactin), and Alp11 (tubulin chaperone cofactor B) that harbor the CAP-glycine motif according to the S. pombe gene function database “PomBase” [Citation52]. Since tip1∆ and ssm4∆ did not restore growth defects caused by Mal3-overexpression (our unpublished data), Tip1 and Ssm4 are unlikely to be the target of Mal3. Because the alp11 gene is essential for growth, we were not able to test the loss of functional effect of alp11 upon Mal3-overexpression. Alp11 remains as a possible target of Mal3 mediated effects.
Previously, we had shown that the pka1∆ strain exhibits the TBZ sensitive phenotype, which can be rescued by overexpression of Mal3 [Citation36]. In the current study, we identified that growth defects and monopolar spindle formation were abolished by the loss of functional Pka1 in Mal3-overxpressing cells. Monopolar spindle formation of the cut7 mutant is rescued by deletion of pkl1 or klp2, both of which encode a kinesin-14 family minus-end directed microtubule motor [Citation50,Citation53]. Overexpression of Mal3 elevated monopolar spindle formation, similar to that found in the cut7 mutant, and the growth defect of the cut7 mutant is consistently rescued by mal3 deletion [Citation21]. In our findings, deletion of pka1 and glucose limitation rescued growth inhibition and monopolar spindle formation in Mal3-overexpressing cells and the cut7-446 mutant. These findings suggest that overexpression of Mal3 might cause inactivation of Cut7, and deletion of pka1 or glucose limitation might affect inactivation of kinesin-14, Pkl1 and/or Klp2. Since overexpression of Mal3 and the cut7 mutation result in increased 4C DNA contents and mitotic defects such as the cut phenotype or chromosome mis-segregation, some cells likely progress DNA replication without mitotic arrest. It has been shown that loss of functional Pka1 rescued the temperature sensitive phenotype of many cut mutants, such as the cut1-693, cut2-364, cut4-533, cut9-665, and cut20-100 mutants [Citation54–Citation57]. These results indicate that lowering Pka1 activity has an apparent benefit for mitotic progression during chromosomal segregation. Separase (Cut1) and securin (Cut2) involve chromosomal segregation and the APC/C complex, of which Cut4, Cut9, and Cut20 ensure chromosomal segregation through degradation of Cut2. Cut7 is a kinesine-5 microtubule motor protein involved in spindle formation, and a cut7-446 mutant, in fact, has a mitotic defect in which cells are separated by the septum before nuclear division that results in a mis-segregated nucleus [Citation16]. Despite this, it remains unclear why loss of functional Pka1 would rescue a defective mitotic phenotype in many cut mutants. One of our hypotheses is that Pka1 may be involved as a spindle assembly checkpoint to monitor aberrant spindle formation and loss of this function could enable mitotic progression. However, further analysis is required to attribute the actual roles of Pka1 to these phenomena.
The growth and cell cycle of S. pombe are affected by medium conditions, specifically the concentration of glucose as a carbon source is important [Citation40]. We found that glucose limitation restored monopolar spindle formation and growth defects caused by Mal3-overexpression and in the cut7-446 mutant. Glucose limitation has been shown to re-localize Pom1 to cell sides by severe microtubule destabilization and strongly delays mitosis [Citation58]. For microtubule destabilization, Pka1 activity is required to regulate the microtubule rescue factor CLASP/Cls1/Peg1 under glucose-limiting conditions [Citation58]. Since glucose limitation decreases cAMP levels and Pka1 remains inactivated due to its binding with Cgs1 [Citation27,Citation33], Pka1 inactivation rescued the phenotypes observed in Mal3-overexpressing cells and the cut7-446 mutant, whereas neither the tor1 nor the ssp2 deletion rescued Mal3-overexpressing and the cut7-446 mutant phenotypes, indicating that the glucose response pathways mediated by Tor1 and Ssp2 are not in charge of microtubule formation. Overexpression of Mal3 (1–241) or Mal3 (Q89R) resulted in severe growth defects and elevated monopolar spindle formation, both in the presence of 2% glucose and in the glucose-limiting conditions ( and ). As these phenotypes were not suppressed by the pka1 deletion (data not shown), the effect of glucose and the relevance of the Pka1 pathway is consistent.
In conclusion, the results of this study demonstrated that the EB1 domain of Mal3 plays a role in proper spindle formation that is coordinated with binding of the Mal3 CH domain binding to microtubules. Our findings show a novel function for Pka1, regulating proper spindle formation, in regard to the CH and EB1 domains of Mal3 and to the cut7-446 mutant.
We propose that Pka1 regulates bipolar spindle formation during mitosis in addition to the known roles of Pka1 in meiosis, gluconeogenesis, chorological aging, stress response, and proper chromosome segregation in S. pombe.
Author contributions
TT planned this study, designed the experiments, carried out the experiments, produced the yeast strains, and analyzed the data; MK analyzed the data and provided advice; YM planned this study, designed the experiments, made plasmids and strains, and analyzed the data. TT and YM wrote the original draft. TT, MK, and YM reviewed and edited the original draft.
Supplemental Material
Download MS Word (56.9 KB)Mal3_OE_supplementary_Figure_5.pdf
Download PDF (41 KB)Mal3_OE_supplementary_Figure_4.pdf
Download PDF (84.2 KB)Mal3_OE_supplementary_Figure_3.pdf
Download PDF (31.5 KB)Mal3_OE_supplementary_Figure_2.pdf
Download PDF (60.5 KB)Mal3_OE_supplementary_Figure_1.pdf
Download PDF (24.5 KB)Acknowledgments
FY10087 (MY2187) and FY13663 (JW952) were provided by the National Bio-Resource Project (NBRP), Japan. We thank Drs. M. Iimori (Kyushu University) and T. Matsumoto (Kyoto University) for the provision of anti-Mal3 antibody. The authors also thank all the members of the laboratory for helpful support and scientific advice. The authors thank the faculty of Life and Environmental Sciences in Shimane University for help in financial support for publication.
Disclosure statement
No potential conflict of interest was reported by the authors.
Supplementary Data
Supplementary material for this article can be accessed here.
Additional information
Funding
References
- Akhmanova A, Steinmetz MO. Microtubule +TIPs at a glance. J Cell Sci. 2010;123(20):3415–3419.
- Lansbergen G, Akhmanova A. Microtubule plus end: a hub of cellular activities. Traffic. 2006;7(5):499–507.
- Beinhauer JD, Hagan IM, Hegemann JH, et al. Mal3, the fission yeast homologue of the human APC-interacting protein EB-1 is required for microtubule integrity and the maintenance of cell form. J Cell Biol. 1997;139(3):717–728.
- Chang F, Martin SG. Shaping fission yeast with microtubules. Cold Spring Harb Perspect Biol. 2009;1(1):a001347.
- Busch KE, Hayles J, Nurse P, et al. Tea2p kinesin is involved in spatial microtubule organization by transporting tip1p on microtubules. Dev Cell. 2004;6(6):831–843.
- Kerres A, Vietmeier-Decker C, Ortiz J, et al. The fission yeast kinetochore component Spc7 associates with the EB1 family member Mal3 and is required for kinetochore-spindle association. Mol Biol Cell. 2004;15(12):5255–5267.
- Matsuo Y, Maurer SP, Yukawa M, et al. An unconventional interaction between Dis1/TOG and Mal3/EB1 in fission yeast promotes the fidelity of chromosome segregation. J Cell Sci. 2016;129(24):4592–4606.
- Gimona M, Djinovic-Carugo K, Kranewitter WJ, et al. Functional plasticity of CH domains. FEBS Lett. 2002;513(1):98–106.
- Hayashi I, Ikura M. Crystal structure of the amino-terminal microtubule-binding domain of end-binding protein 1 (EB1). J Biol Chem. 2003;278(38):36430–36434.
- Des Georges A, Katsuki M, DR D, et al. Mal3, the Schizosaccharomyces pombe homolog of EB1, changes the microtubule lattice. Nat Struct Mol Biol. 2008;15(10):1102–1108.
- Maurer SP, Fourniol FJ, Bohner G, et al. EBs recognize a nucleotide-dependent structural cap at growing microtubule ends. Cell. 2012;149(2):371–382.
- Iimori M, Ozaki K, Chikashige Y, et al. A mutation of the fission yeast EB1 overcomes negative regulation by phosphorylation and stabilizes microtubules. Exp Cell Res. 2012;318(3):262–275.
- Blangy A, Lane HA, d’Herin P, et al. Phosphorylation by p34cdc2 regulates spindle association of human Eg5, a kinesin-related motor essential for bipolar spindle formation in vivo. Cell. 1995;83(7):1159–1169.
- Enos AP, Morris NR. Mutation of a gene that encodes a kinesin-like protein blocks nuclear division in A. nidulans. Cell. 1990;60(6):1019–1027.
- Goshima G, Vale RD. The roles of microtubule-based motor proteins in mitosis: comprehensive RNAi analysis in the Drosophila S2 cell line. J Cell Biol. 2003;162(6):1003–1016.
- Hagan I, Yanagida M. Novel potential mitotic motor protein encoded by the fission yeast cut7+ gene. Nature. 1990;347(6293):563–566.
- Hoyt MA, He L, Loo KK, et al. Two Saccharomyces cerevisiae kinesin-related gene products required for mitotic spindle assembly. J Cell Biol. 1992;118(1):109–120.
- Mann BJ, Wadsworth P. Kinesin-5 regulation and function in mitosis. Trends Cell Biol. 2019;29(1):66–79.
- Akera T, Goto Y, Sato M, et al. Mad1 promotes chromosome congression by anchoring a kinesin motor to the kinetochore. Nat Cell Biol. 2015;17(9):1124–1133.
- Hirano T, Funahashi S, Uemura T, et al. Isolation and characterization of Schizosaccharomyces pombe cut mutants that block nuclear division but not cytokinesis. Embo J. 1986;5(11):2973–2979.
- Yukawa M, Yamada Y, Toda T. Suppressor analysis uncovers that maps and microtubule dynamics balance with the Cut7/Kinesin-5 motor for mitotic spindle assembly in Schizosaccharomyces pombe. G3. 2019;9(1):269–280.
- Hoffman CS. Glucose sensing via the protein kinase A pathway in Schizosaccharomyces pombe. Biochem Soc Trans. 2005;33(1):257–260.
- Kim JH, Roy A, Jouandot D, et al. The glucose signaling network in yeast. Biochim Biophys Acta. 2013;1830(11):5204–5210.
- Santangelo GM. Glucose signaling in Saccharomyces cerevisiae. Microbiol Mol Biol Rev. 2006;70(1):253–282.
- Van Ende M, Wijnants S, Van Dijck P. Sugar sensing and signaling in Candida albicans and Candida glabrata. Front Microbiol. 2019;10:99.
- DeVoti J, Seydoux G, Beach D, et al. Interaction between ran1+ protein kinase and cAMP dependent protein kinase as negative regulators of fission yeast meiosis. Embo J. 1991;10(12):3759–3768.
- Gupta DR, Paul SK, Oowatari Y, et al. Multistep regulation of protein kinase A in its localization, phosphorylation and binding with a regulatory subunit in fission yeast. Curr Genet. 2011;57(5):353–365.
- Kawamukai M, Ferguson K, Wigler M, et al. Genetic and biochemical analysis of the adenylyl cyclase of Schizosaccharomyces pombe. Cell Regul. 1991;2(2):155–164.
- Maeda T, Watanabe Y, Kunitomo H, et al. Cloning of the pka1 gene encoding the catalytic subunit of the cAMP-dependent protein kinase in Schizosaccharomyces pombe. J Biol Chem. 1994;269(13):9632–9637.
- Matsuo Y, Kawamukai M. cAMP-dependent protein kinase involves calcium tolerance through the regulation of Prz1 in Schizosaccharomyces pombe. Biosci Biotechnol Biochem. 2017;81(2):231–241.
- Matsuo Y, McInnis B, Marcus S. Regulation of the subcellular localization of cyclic AMP-dependent protein kinase in response to physiological stresses and sexual differentiation in the fission yeast Schizosaccharomyces pombe. Eukaryot Cell. 2008;7(9):1450–1459.
- Welton RM, Hoffman CS. Glucose monitoring in fission yeast via the Gpa2 galpha, the git5 Gbeta and the git3 putative glucose receptor. Genetics. 2000;156(2):513–521.
- Byrne SM, Hoffman CS. Six git genes encode a glucose-induced adenylate cyclase activation pathway in the fission yeast Schizosaccharomyces pombe. J Cell Sci. 1993;105(4):1095-1100.
- Roux AE, Quissac A, Chartrand P, et al. Regulation of chronological aging in Schizosaccharomyces pombe by the protein kinases Pka1 and Sck2. Aging Cell. 2006;5(4):345–357.
- Stiefel J, Wang L, Kelly DA, et al. Suppressors of an adenylate cyclase deletion in the fission yeast Schizosaccharomyces pombe. Eukaryot Cell. 2004;3(3):610–619.
- Tanabe T, Yamaga M, Kawamukai M, et al. Mal3 is a multi-copy suppressor of the sensitivity to microtubule-depolymerizing drugs and chromosome mis-segregation in a fission yeast pka1 mutant. PLoS One. 2019;14(4):e0214803.
- Wu SY, McLeod M. The sak1+ gene of Schizosaccharomyces pombe encodes an RFX family DNA-binding protein that positively regulates cyclic AMP-dependent protein kinase-mediated exit from the mitotic cell cycle. Mol Cell Biol. 1995;15(3):1479–1488.
- Gupta DR, Paul SK, Oowatari Y, et al. Complex formation, phosphorylation, and localization of protein kinase A of Schizosaccharomyces pombe upon glucose starvation. Biosci Biotechnol Biochem. 2011;75(8):1456–1465.
- Murray JM, Watson AT, Carr AM. Transformation of Schizosaccharomyces pombe: lithium acetate/dimethyl sulfoxide procedure. Cold Spring Harb Protoc. 2016. DOI:10.1101/pdb.prot090969
- Petersen J, Russell P. Growth and the environment of Schizosaccharomyces pombe. Cold Spring Harb Protoc. 2016. DOI:10.1101/pdb.top079764
- Ekwall K, Thon G. Spore analysis and tetrad dissection of Schizosaccharomyces pombe. Cold Spring Harb Protoc. 2017. DOI:10.1101/pdb.prot091710
- Forsburg SL. Comparison of Schizosaccharomyces pombe expression systems. Nucleic Acids Res. 1993;21(12):2955–2956.
- Matsuo Y, Tanaka K, Nakagawa T, et al. Genetic analysis of chs1+ and chs2+ encoding chitin synthases from Schizosaccharomyces pombe. Biosci Biotechnol Biochem. 2004;68(7):1489–1499.
- Kanda Y. Investigation of the freely available easy-to-use software ‘EZR’ for medical statistics. Bone Marrow Transplant. 2013;48(3):452–458.
- Matsuzawa T, Fujita Y, Tohda H, et al. Snf1-like protein kinase Ssp2 regulates glucose derepression in Schizosaccharomyces pombe. Eukaryot Cell. 2012;11(2):159–167.
- Saitoh S, Mori A, Uehara L, et al. Mechanisms of expression and translocation of major fission yeast glucose transporters regulated by CaMKK/phosphatases, nuclear shuttling, and TOR. Mol Biol Cell. 2015;26(2):373–386.
- Mana-Capelli S, McLean JR, Chen CT, et al. The kinesin-14 Klp2 is negatively regulated by the SIN for proper spindle elongation and telophase nuclear positioning. Mol Biol Cell. 2012;23(23):4592–4600.
- Nehlig A, Molina A, Rodrigues-Ferreira S, et al. Regulation of end-binding protein EB1 in the control of microtubule dynamics. Cell Mol Life Sci. 2017;74(13):2381–2393.
- Snaith HA, Thompson J, Yates JR 3rd, et al. Characterization of Mug33 reveals complementary roles for actin cable-dependent transport and exocyst regulators in fission yeast exocytosis. J Cell Sci. 2011;124(13):2187–2199.
- Yukawa M, Yamada Y, Yamauchi T, et al. Two spatially distinct kinesin-14 proteins, Pkl1 and Klp2, generate collaborative inward forces against kinesin-5 Cut7 in S. pombe. J Cell Sci. 2018;131(1):jcs210740.
- Akhmanova A, Steinmetz MO. Tracking the ends: a dynamic protein network controls the fate of microtubule tips. Nat Rev Mol Cell Biol. 2008;9(4):309–322.
- Wood V, Harris MA, McDowall MD, et al. PomBase: a comprehensive online resource for fission yeast. Nucleic Acids Res. 2011;40:D695–9.
- Yukawa M, Kawakami T, Okazaki M, et al. A microtubule polymerase cooperates with the kinesin-6 motor and a microtubule cross-linker to promote bipolar spindle assembly in the absence of kinesin-5 and kinesin-14 in fission yeast. Mol Biol Cell. 2017;28(25):3647–3659.
- Kawasaki Y, Nagao K, Nakamura T, et al. Fission yeast MAP kinase is required for the increased securin-separase interaction that rescues separase mutants under stresses. Cell Cycle. 2006;5(16):1831–1839.
- Yamashita YM, Nakaseko Y, Kumada K, et al. Fission yeast APC/cyclosome subunits, Cut20/Apc4 and Cut23/Apc8, in regulating metaphase-anaphase progression and cellular stress responses. Genes Cells. 1999;4(8):445–463.
- Yamashita YM, Nakaseko Y, Samejima I, et al. 20S cyclosome complex formation and proteolytic activity inhibited by the cAMP/PKA pathway. Nature. 1996;384(6606):276–279.
- Yanagida M, Yamashita YM, Tatebe H, et al. Control of metaphase-anaphase progression by proteolysis: cyclosome function regulated by the protein kinase A pathway, ubiquitination and localization. Philos Trans R Soc Lond B Biol Sci. 1999;354(1389):1559–1570.
- Kelkar M, Martin SG. PKA antagonizes CLASP-dependent microtubule stabilization to re-localize Pom1 and buffer cell size upon glucose limitation. Nat Commun. 2015;6(8445):8445.