Abstract
Within the framework of the two-scale scattering model, Doppler shift and bandwidth of microwave scattering field from two-dimensional (2-D) sea surface have been simulated. And the comparisons of the simulated results with the Doppler anomaly measurements from Envisat ASAR demonstrate the rationality of the formulas proposed by us. Meanwhile, the influences of the tilt and the hydrodynamic modulations due to large-scale underlying waves on Doppler shift and bandwidth have also been discussed in detail. Numerical results show that the Doppler shifts for HH-and VV-polarizations are both observably affected by the tilt and the hydrodynamic modulations and the values of the predicted Doppler shifts are always larger than Bragg frequency. Because the radar scattering coefficient is more sensitive to sea surface slope in HH polarization than in VV, the predicted Doppler shifts in HH polarization are always somewhat larger than those in VV. Moreover, we also find that there is a remarkable difference between the Doppler shift predicted in upwind and downwind direction due to the hydrodynamic modulations. Relative to Doppler shift, bandwidth of Doppler spectrum is mainly affected by orbit velocity of large-scale waves and is insensitive to the modulations and radar polarizations.
1. Introduction
Doppler shift and bandwidth are important parameters of Doppler spectrum and correspond to the distribution of the power-weighted line-of-sight velocity of the scattering facets. Thus, the studies on Doppler spectrum of electromagnetic scattering fields from sea surface are of practical importance in a number of research areas such as sea surface wind retrieving [Citation1] and sea surface current measuring.[Citation2–7] Up to now, Doppler spectra of microwave signal backscattered from sea surface have been studied extensively.[Citation8–19] Experiment results show that the Doppler shifts are larger than Bragg frequency. Moreover, the Doppler shifts of horizontally polarized (HH-polarization) signals are generally larger than those for vertically polarized (VV-polarization) case. To explain those anomalous properties, microwave Doppler spectra from sea surfaces were investigated by numerical [Citation10–12] and asymptotic methods.[Citation15–19] In paper [Citation10], Doppler spectra were simulated by the method of moment (MoM) and the numerical simulations show an obvious separation between the vertical and horizontal polarization spectra. In papers [Citation11], Jonhson and Hayslip investigated the influences of the hydrodynamic sea surface models on Doppler spectra. Although the anomalous properties of Doppler spectrum have been reproduced in [Citation10,11], the reasons for the anomalous properties cannot be explained by numerical methods. For microwave scattering from sea surface, the two scale method (TSM) can be used to explain the polarization dependence of the microwave signals. Up to now, TSM is still the most practical model for theoretical description of microwave Doppler spectrum from sea surface. In [Citation20], the anomalous properties of Doppler spectrum have been explained by TSM. And the authors pointed out that not only the scattering filed from free-waves but also that from bound waves would impact Doppler property remarkably. And the so-called “bound waves” are always considered as “fast scatterers” which localize on the front faces of large scale waves in the vicinity of their crests and propagate with velocity close to the phase velocity of the large scale gravity waves. In Refs. [Citation10,11,15–19] significant differences between co-polarized predicated Doppler shifts have also been shown. However, it should be pointed out that in Refs. [Citation10,11,15–19] the influences of the scattering fields from “fast scatterers” are not considered and the results imply that not only the so-called “fast scatterers” but also the other mechanisms may be required to explain the anomalous properties of Doppler spectrum. Since Doppler spectra are weight by received power, some scattering mechanisms, such as the tilt and the hydrodynamic modulations of large-scale waves, may be responsible for the anomalous properties of Doppler spectrum. In [Citation18,19], the influences of these modulations were studied and a theoretical model of Doppler spectrum from one-dimensional sea surface was proposed. The numerical results in [Citation18,19] show that the modulations of large-scale waves have obvious influences on Doppler shift. Nevertheless, in the previous works [Citation17–19] only the scattering field from one-dimensional sea surface has been discussed. It is well known that 2-D sea surface model is more suitable for the practice.
This study is an extension of our previous work mentioned in [Citation19]. In the present investigation, at moderate-incident angles, the analytical formulas for Doppler shift and spectral width of microwave scattering from two-dimensional sea surface are derived on the basis of the two-scale scattering model. And this work focuses on analyzing the influences of the tilt modulation, the hydrodynamic modulation, the wind speed and the radar look direction on Doppler shift and spectral width. In order to demonstrate the validity of our formula, the predicted Doppler shifts have been compared with the results by the Geophysical model function C-band Doppler shift model (CDOP) and the measurements from Envisat ASAR. The rest of the paper is organized as follows. In Section 2, a linear hydrodynamic theory is employed to represent 2-D time-varying sea surface. In Sections 3 and 4, the analytical formulas for Doppler shift and bandwidth are derived when the effects of the tilt and the hydrodynamic modulations of large-scale waves are taken into account. In order to verify the theoretical Doppler shift model, an empirical model, called CDOP, is also given out in Section 5. The Section 6 is devoted to the results and the comments, while the concluding remarks and perspectives are provided in Section 7.
2. The two-scale model
In the two-scale scattering model (TSM), 2-D sea surface is partitioned into small- and large-scale waves, such that(1)
where Zl(x, y, t) represents the large-scale portion of the surface elevation. Zs(x, y, t), which has been modulated by large-scale waves, denotes small-scale roughness with 2ki cos θiZs(x, y, t) < < 1.0. ki is the wave number of microwave and θi is the incidence angle. If we assume that Zl(x, y, t) and Zs(x, y, t) are statistically independent, the total wave-height spectrum, i.e. , can be written as
(2)
where and
denote the wave-height spectra corresponding to small-and large-scale surface roughness. In this paper, the two-dimensional spectrum proposed by Apel [Citation21] is selected for sea waves.
On the bases of the Longuet-Higgins model, the large-scale waves can be simulated by(3)
(4)
(5)
where (x0, y0, z0) denotes the equilibrium position of each water particle on sea surface, F(Kxm, Kyn) is the Fourier coefficients of sea profile, the wavenumber and the angular frequency are and
with Kxm = 2π(m−M/2)/Lx, Kyn = 2π(n−N/2)/Ly Lx and Ly are the lengths of sea surface along
and
directions,
,
. In the first order approximation, the velocity components and the surface slopes can be expressed as
(6)
(7)
(8)
(9)
(10)
As is known to us all, the small-scale roughness of sea surface would be modulated by large-scale underlying waves and the spectral disturbance induced by the hydrodynamic modulation of large-scale waves can be written as(11)
Suppose that the short wave spectrum Wms is proportional to , the hydrodynamic modulation transfer function Rhydr takes the form as [Citation22]
(12)
where and
denote the wavenumbers of large- and small-scale waves, respectively. μ is the relaxation rate and has to be determined by experiment. For μ = 0, the maximum of the short wave energy occurs at the crests of the large-scale waves. However, the fact is that a nonvanishing phase shift between the maximum of the short wave spectral energy and the large-scale waves’ crest exists, which yields the maximum of the short wave energy on the front faces of long waves. Unfortunately, the value of the relaxation rate is still poorly known up to now. And the values estimated by various investigators differ by almost one order of magnitude [Citation23]. From the discussions in Section 6 of this paper, we find that a reasonable Doppler shift can be obtained if the nonvanishing phase shift is set to be about π/4 (i.e. μ = ωp). Here, ωp denotes the angle frequency of the spectral peak wave.
Thus, the roughness spectrum for the short ripples modulated by large-scale waves can be written as [Citation18,19].(13)
where Ws denotes the roughness spectrum which is not modulated by large-scale waves.
3. The joint probability density function
Let denote the joint probability density function (PDF) of vector
. Because
are all Gaussian random processes, then the six-dimensional PDF
can be written as [Citation24]
(14)
In (12), the vector is
(15)
And the corresponding covariance matrix(16)
In (14), denotes the determinant of the covariance matrix. Bij denotes the cofactor of the element mij of
.
4. Doppler shift and bandwidth
Doppler shift fDpp and bandwidth can be generally considered as the first- and the second-order moments of Doppler spectrum and weighted by scattering power [Citation25,26]. If the effect of Bragg frequency is removed, then the Doppler shift
and the bandwidth
are defined as
(17)
and(18)
where the subscript pp represents HH or VV polarization. The symbol denotes ensemble average, and
(19)
In Equations (Equation17(17) ) and (Equation18
(18) ), the Doppler frequency f is related to orbit velocity by
(20)
θi and φi denotes the incident angle and the azimuth angle.
Converting Equations (Equation17(17) ) and (Equation18
(18) ) into integral forms, we can obtain that
(21)
and(22)
here, represents the local incident angle.
Substituting (14) into (21) and (22) and performing integration over Vz, Vx, Vy, and Hy, we can obtain that(23)
(24)
with
In our simulations, we perform the integrations in (23) and (24) over Sx and Sy from −3γLs to 3γLs. Here, γLs denotes the mean RMS slope of large scale waves along and
direction.
Considering the effects of Bragg frequency and wind drift current, the total Doppler shift fDpp then can be written as(25)
with(26)
(27)
where the surface drift .
denotes the wind field at a height of 10 m above sea surface. KBragg and ϕB denote the wave number and azimuth angle of Bragg wave. And F(K, ϕ) is the angle spread function proposed in [Citation21].
5. Numerical results and discussions
In Figure , the predicted Doppler shifts at C-band (5.33 GHz) VV polarization as a function of azimuth angle are compared with the measurements from Envisat ASAR. Azimuth angle or
(
) indicates the upwind (downwind) configuration when the wind blows toward (away from) the antenna in the range direction. In order to predict Doppler shift by (23), the scattering coefficient, i.e.
, should be determined firstly. For the case of C-band microwave scattering in this paper, CMOD5, which is a geophysical model function for C-band VV polarization microwave backscattering from sea surface, is used to evaluate the scattering coefficient
[Citation27]. From the comparisons in Figure , we can find that the results evaluated by the theoretical Doppler shift model fit well with the measurements from Envisat ASAR.
Figure 1. Comparisons of the predicted Doppler shifts (solid line) by (23) with the measurements from Envisat ASAR (open circles) for different incidence angles. (a) ; (b)
.
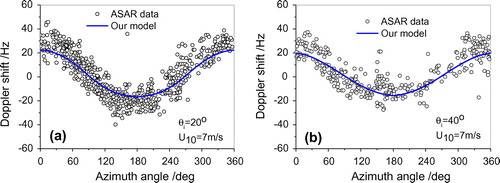
To further verify the theoretical Doppler shift model, the simulated Doppler shifts by Equation (Equation23(23) ) are also compared with the results of CDOP, which is proposed by Mouche in [Citation1] to provide the wind-dependent Doppler shift, for different incident angles and wind speeds in Figure . Just as in Figure , the scattering coefficient
is calculated by CMOD5. Nevertheless, the scattering coefficient for HH polarization i.e.
is evaluated by the relationship
, where app denotes the geometric factor in Bragg scattering theory and
is co-polarization power ratio. The comparisons in Figure show that the Doppler shifts predicted by (23) are in agreement with the results of CDOP for different incident angles and wind speeds. From Figure we can see that at moderate incidence angles the values of Doppler shifts are insensitive to incidence angle but increase with wind speed. Meanwhile, Figure also shows that the absolute values of Doppler shifts in HH polarization are always larger than the corresponding values in VV polarization because the tilt modulation for HH polarization is obviously larger than that for VV polarization (As shown in Figure of [Citation19]).
Figure 2. Comparisons between the Doppler shifts evaluated by CDOP (Squares and stars) and our model (Solid and dashed lines) for different incidence angles and wind speeds.
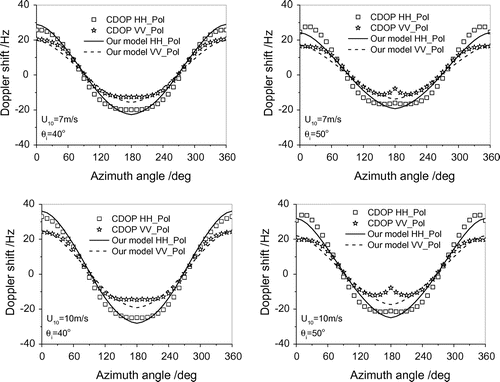
To further illustrate the influence of the slope of large scale waves, the predicted Doppler shifts are shown in Figure for different integral intervals in (23). Here, the incidence angle and the wind speed
. The curves in Figure show that the Doppler shifts due to Bragg frequency and surface drift current have nothing to do with radar polarization. However, if we increase the integral interval, the absolute value of the predicted Doppler shift will increase. And the Doppler shift for HH polarization is more sensitive to surface slope than VV polarization. However, when the integral limit is larger than 3γLs, the Doppler shift for both polarizations tends to be constant.
Figure 3. The influence of the integral interval on the predicted Doppler shift. (a) For VV polarization; (b) for HH polarization.
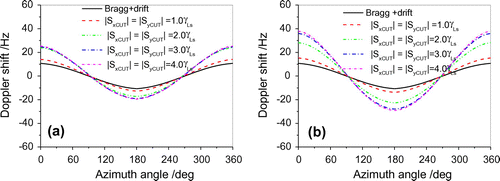
As shown in Figures , a remarkable difference between the absolute values of the Doppler shifts predicted in upwind direction (azimuth angle or
) and downwind direction (azimuth angle
). Generally, the absolute values in upwind direction are always larger than in downwind direction. The numerical results in [Citation18] showed that the horizontal-skewness of large-scale waves would induce this difference. However, at moderate incidence angle, the influence of the horizontal-skewness is insignificant, especially for VV polarization. The curves in Figure show the influences of the relaxation rate on the frequency difference for various wind speeds. Because Doppler shifts are weighted by the power of the scattering field, thus the hydrodynamic modulation would induce an effect on this difference. From Figure (a), we can find that this difference gradually increases as a function of the relaxation rate until a peak is reached, after which the difference decreases with the relaxation rate. The relaxation rate at which this peak occurs decreases with the increasing wind speed. The frequency difference as a function of the ratio between the relaxation rate and ωp is shown in Figure (b). From the curves, however, we can find that the values of the ratio at which the peaks occur are almost unrelated to windspeed and are about equal to 2.0. Because the hydrodynamic modulation of large-scale waves is more significant with wind speed, the frequency difference is also increased with wind speed.
Figure 4. The influence of the relaxation rate on the difference between the Doppler shifts predicted in upwind and downwind directions for different wind speeds.
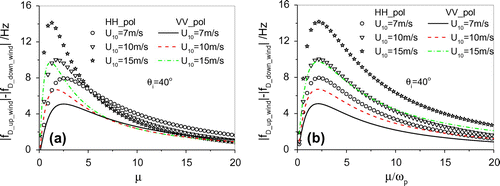
The predicted bandwidths in upwind direction for different wind speeds are shown in Figure . We can find that the influence of wind speed is very significant and the predicted bandwidth increases with the increasing wind speed. However, the predicted bandwidth is insensitive to radar polarization, relaxation rate, and incidence angle.
Figure 5. The predicted bandwidth as a function of the relaxation rate (a) and incidence angle (b) for difference wind speeds.
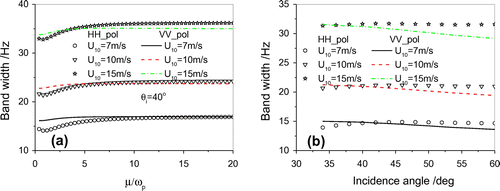
The predicted Doppler shifts and bandwidths for difference radar frequencies are shown in Figure . Here, the polarization of radar is VV-pol, the incidence angle , and the wind speed is U10 = 10 m/s. Here, in (23) and (24), the scattering coefficients for L- and Ku-band microwave scattering are evaluated by the geophysical model functions proposed in Refs. [Citation28] and [Citation29], respectively. As shown in Figure , not only Doppler shift but bandwidth is closely related to radar frequency. The higher the radar frequency is, the larger the values of the Doppler shift and bandwidth. From Figure (b), one can also find that the bandwidths in crosswind direction (azimuth angle
or
) are somewhat smaller than the bandwidths corresponding to upwind and downwind direction.
6. Conclusion
At moderate-incidence angles, the analytical formulas for Doppler shift and bandwidth of microwave echoes scattered from 2-D sea surface have been derived in this work. The predicted Doppler shifts by our formula are compared with the results by the Geophysical model function CDOP and the measurements from Envisat ASAR. And these comparisons demonstrate the validity and practicability of our formula. From the predicted Doppler shifts, we can also find that not only the tilt modulation but also the hydrodynamic modulation has significant effect on Doppler shift. The Doppler shift for HH-polarization is always larger than that in VV-polarization due to the tilt modulation. On the other hand, the difference between the Doppler shifts predicted in upwind and downwind directions is mainly affected by the hydrodynamic modulation, although the horizontal-skewness of large-scale waves would also induce this difference. Because the spectral width is mainly affected by orbit velocity of large-scale waves, the influence of wind speed on bandwidth is very significant. However, unlike the predicted Doppler shift, the spectral width is quasi-insensitive to the modulations, radar polarization, and incidence angle.
Disclosure statement
No potential conflict of interest was reported by the authors.
Funding
This work was supported by the National Natural Science Foundation of China under [grant number 41376179]; and the National Natural Science Foundation of China -Shandong Joint Fund for Marine Science Research Centers [grant number U1406404].
References
- Mouche AA, Collard F, Chapron B, et al. On the Use of Doppler Shift for Sea Surface Wind Retrieval from SAR. IEEE Trans. Geosci. Remote Sens. 2012;50:2901–2909.
- Chapron B, Collard F, Ardhum F. Direct measurements of ocean surface velocity from space: Interpretation and validation. J. Geophys. Res. 2005;110:1–17.
- Mouche A, Chapron B, Reul N, et al. Predicted Doppler shifts induced by ocean surface wave displacements using asymptotic electromagnetic wave scattering theories. Waves Random Complex Media. 2008;18:185–196.10.1080/17455030701564644
- Karaev V, Kanevsky M, Meshkov E. The effect of sea surface slicks on the Doppler spectrum width of a backscattered microwave signal. Sensors. 2008;8:3780–3801.
- Johannessen JA, Kudryavtsev V, Akimov D, et al. On radar imaging of current features; Part 2: mesoscale Eddy and current front detection. J. Geophys. Res. 2005;110:1–14.
- Kudryavtsev V, Akimov D, Johannessen JA, et al. On radar imaging of current features. Part1: model and comparison with observations. J. Geophys. Res. 2005;110:1–27. doi:10.1029/2004JC002505.
- Johnson JT, Burkholder RJ, Toporkov JV, et al. A numerical study of the retrieval of sea surface height profiles from low grazing angle radar data. IEEE Trans. Geosci. Remote Sens. 2009;47:1641–1650.10.1109/TGRS.2008.2006833
- Qi C, Zhao Z, Yang W, et al. Electromagnetic scattering and Doppler analysis of three-dimensional breaking wave crests at low-grazing angles. Prog. Electromagnet. Res. 2011;119:239–252.10.2528/PIER11062401
- Soriano G, Joelson M, Saillard M, et al. Doppler spectra from a two- dimensional ocean surface at L-band. IEEE Trans. Geosci. Remote Sens. 2006;44:2430–2437.10.1109/TGRS.2006.873580
- Toporkov JV, Brown GS. Numerical simulations of scattering from time-varying randomly rough surfaces. IEEE Trans. Geosci. Remote Sens. 2000;38:1616–1625.10.1109/36.851961
- Johnson JT, Toporkov JV, Brown GS. A numerical study of backscattering from time-evolving sea surfaces: comparison of hydrodynamic models. IEEE Trans. Geosci. Remote Sens. 2001;39:2411–2420.10.1109/36.964977
- Hayslip AR, Johnson JT, Baker GR. Further numerical studies of back- scattering from time-evolving nonlinear sea surfaces. IEEE Trans. Geosci. Remote Sens. 2003;41:2287–2293.10.1109/TGRS.2003.814662
- Saillard M, Forget P, Soriano G, et al. Sea surface probing with L-band Doppler radar: experiment and theory. C.R. Phys. 2005;6:675–682.10.1016/j.crhy.2005.06.008
- Hisaki Y. Doppler spectrum of radio wave scattering from ocean-like moving surfaces for a finite illuminated area. Int. J. Remote Sens. 2003;24:3075–3091.10.1080/01431160210153057
- Nouguier F, Guerin CA, Soriano G. Analytical techniques for the Doppler signature of sea surfaces in the microwave regime-I: linear surfaces. IEEE Trans. Geosci. Remote Sens. 2011;49:4856–4864.10.1109/TGRS.2011.2152848
- Nouguier F, Guerin CA, Soriano G. Analytical techniques for the Doppler signature of sea surfaces in the microwave regime-II: nonlinear surfaces. IEEE Trans. Geosci. Remote Sens. 2011;49:4920–4927.10.1109/TGRS.2011.2153207
- Wang YH, Zhang YM. Investigation on Doppler shift and bandwidth of backscattered echoes from a composite sea surface. IEEE Trans. Geosci. Remote Sens. 2011;49:2287–2293.
- Wang YH, Zhang YM, He MX, et al. Doppler spectra of microwave scattering fields from nonlinear oceanic surface at moderate- and low-grazing angles. IEEE Trans. Geosci. Remote Sens. 2012;50:1104–1116.
- Wang Y, Zhang Y, Guo L. Microwave Doppler spectra of sea echoes at high incidence angles: influences of large-scale waves. Prog. Electromagnet. Res. B. 2013;48:99–113.
- William J. Plant, A model for microwave Doppler sea return at high incidence angles: Bragg scattering from bound, tilted waves. J. Geophys. Res. 1997;102:21131–21146.
- Apel JR. An improved model of the ocean surface wave vector spectrum and its effects on radar backscatter. J. Geophys. Res. 1994;99:16269–16291.10.1029/94JC00846
- Alpers W, Ross DB, Rufenach CL. On the detectability of ocean surface waves by real and synthetic aperture radar. J. Geophys. Res. 1981;86:6481–6498.10.1029/JC086iC07p06481
- Caponi EA, Crawford DR, Yuen HC, et al. Modulation of radar backscatter from the ocean by a variable surface current. J. Geophys. Res. 1988;93:12249–12263.10.1029/JC093iC10p12249
- Fisz M, Probability theory and mathematical statistics. New York (NY): John Wiley and Sons; 1963.
- Romeiser R, Thompson DR. Numerical study on the along-track interferometric radar imaging mechanism of oceanic surface currents. IEEE Trans. Geosci. Remote Sens. 2000;38:446–458.10.1109/36.823940
- Keller WC, Plant WJ. Microwave backscatter from the sea: modulation of received power and Doppler bandwidth by long waves. J. Geophys. Res. 1994;99:9751–9766.10.1029/94JC00082
- Hersbach H, Stoffelen A, de Haan S. An improved C-band scatterometer ocean geophysical model function: CMOD5. J. Geophys. Res. 2007;112:1–18. doi:10.1029/2006JC003743.
- Isoguchi O, Shimada M. An L-band ocean geophysical model function derived from PALSAR. IEEE Trans. Geosci. Remote Sens. 2009;47:1925–1936.10.1109/TGRS.2008.2010864
- Wentz FJ, Smith DK. A model function for the ocean- normalized radar cross section at 14 GHz derived from NSCAT observations. J. Geophys. Res. 2007;104:11499–11514.