ABSTRACT
In this study, we explore the issues and challenges involved in supporting students’ learning to discern relevant and critical aspects of determining oxidation states of atoms in complex molecules. We present a detailed case of an interaction between three students and a tutor during a problem-solving class, using the analytical tool of practical epistemology analysis (PEA). The results show that the ability to make relevant distinctions between the different parts of a molecule for solving the problem, even with the guidance of the tutor, seemed to be challenging for students. These shifts were connected to both purposes that were specific for solving the problem at hand, and additional purposes for general learning of the subject matter, in this case how to assign oxidation states in molecules. The students sometimes could not follow the additional purposes introduced by the tutor, which made the related distinctions more confusing. Our results indicate that in order to provide adequate support and guidance for students the tutor needs to consider how to sequence, move between, and productively connect the different purposes introduced in a tutor-student interaction. One way of doing that is by first pursuing the purposes for solving the problem and then successively introduce additional, more general purposes for developing students’ learning of the subject matter studied. Further recommendations drawn from this study are discussed as well.
Introduction
In this study, we analyze the interaction between three first-year university students and their tutor as they struggle to understand how to approach a complex molecular structure in order to determine the oxidation states of its atoms. Oxidation states, or oxidation numbers, are hypothetical charges assigned to atoms that help keeping track of the transfer of electrons in redox reactions. General chemistry textbooks (e.g. Atkins & Beran, Citation1992; Atkins & Jones, Citation2005; Burrows, Holman, Parsons, Pilling, & Price, Citation2013; Zumdahl & Zumdahl, Citation2007) give a simple set of rules for assigning oxidation states of atoms in compounds stemming from the IUPAC definition. According to this definition, the oxidation state of a free element is zero, that of a monatomic ion is the same as its charge, and that of fluorine −1, oxygen −2 (except in peroxide where it is −1), and hydrogen +1. The sum of oxidation states is equal to the overall charge of the molecule. These rules are commonly followed by examples of simple molecules such as H2O, HF and CO2 to illustrate how to assign the oxidation states of the different atoms in a compound. Applying these rules to simple molecules is quite straightforward but the process can be considerably more demanding when dealing with polyatomic and complex molecules such as metal complexes and organic compounds (Birk, Citation1992; Calzaferri, Citation1999; Eggert, Middlecamp, & Kean, Citation1990; Gupta, Ganegoda, Engelhard, Terry, & Linford, Citation2014; Halkides, Citation2000; Jensen, Citation2011; Jurowski, Krzeczkowska, & Jurowska, Citation2015; Steinborn, Citation2004).
The meaning and the assignment of oxidation states of atoms has been shown to be problematic to students in relation to redox reactions (e.g. Brandriet & Bretz, Citation2014; de Jong & Treagust, Citation2002; de Jong, Acampo, & Verdonk, Citation1995; Garnett & Treagust, Citation1992). In particular, students seem to have problems differentiating between oxidation state and charge (e.g. Brandriet & Bretz, Citation2014; Garnett & Treagust, Citation1992). For instance, in the study of Brandriet and Bretz (Citation2014), students believed that both sulfur and sulfate have oxidation states and charges. Garnett and Treagust (Citation1992) showed that students believe that oxidation states can be assigned by change in charges in polyatomic molecules instead of changes in oxidation states for individual atoms. Moreover, students have problems identifying oxidising and reducing agents (de Jong & Treagust, Citation2002). Students struggle even more with determining oxidation states of atoms when dealing with redox reactions involving complex molecules in organic and biochemistry, especially oxidation states of each carbon atom in the reaction (e.g. Halkides, Citation2000; Shibley, Amaral, & Aurentz, Citation2010). Also, teaching problems concerning oxidation states have been shown to arise in the classroom. For instance, de Jong et al. (Citation1995) showed that teachers introduce the concept of oxidation state without relating it to the meaning within the specific context in which it is used. Consequently, students considered oxidation states as fixed values of ion charges instead of numbers with formal value used for balancing redox equations. According to the students, the formal meaning was not plausible for nonionic compounds.
In order to make the process of determining oxidation states of atoms in molecules manageable for students, several researchers (e.g. Calzaferri, Citation1999; Gupta et al., Citation2014; Holleran & Jespersen, Citation1980; Jørgensen, Citation1969; Jurowski et al., Citation2015; Kauffman, Citation1986; Loock, Citation2011; Packer & Woodgate, Citation1991; Steinborn, Citation2004; Woolf, Citation1988) have suggested different approaches. These approaches vary from simple rules as those presented above to more complex sets of rules taking into account many exceptions such as different oxidation states of atoms in different molecules (e.g. transition metals, carbon and nitrogen) and similar electronegativity values. Some of the studies have focused particularly on how to determine oxidation states of atoms in complex molecules such as organic molecules and metal complexes (Bentley, Franzen & Chasteen, Citation2002; Calzaferri, Citation1999; Gupta et al., Citation2014; Halkides, Citation2000; Jurowski et al., Citation2015; Steinborn, Citation2004). According to Calzaferri (Citation1999), applying a set of rules for determining oxidation states of atoms in organic molecules is not enough which is due to the richness reflected by the homopolar nature of C–C and C–H bonds in these molecules, a problem discussed by Jørgensen (Citation1969). Carbon, then, can have different oxidation states that range from −4 (e.g. CH4) to +4 (e.g. CO2). Calzaferri (Citation1999) suggested that oxidation states can be ascribed by analogy (substitution of similar atoms or groups) to compounds where the oxidation states are determined from the specific rules. Halkides (Citation2000) illustrated how to determine oxidation states of carbon atoms in a given organic molecule focusing on carbon atoms in different functional groups in a molecule (e.g. carbonyl carbon and methyl carbon). In addition, Steinborn (Citation2004) discussed oxidation states of atoms in another kind of complex molecules, namely metal complexes. According to him, electrons involved in metal–ligand bonds are assigned to the ligands and then, the oxidation state of the metal is the overall charge of the whole molecule minus the sum of the charges of all ligands. Indeed, a set of rules can be useful for determining oxidation states in molecules, especially with increasing complexity. However, these sets of rules have important limitations related to the complexity of molecules, exceptions to the rules, and how parts such as functional groups or ligands are recognised.
There are several studied that showed that tasks related to molecular structures are challenging for students and in particular deriving relevant information from a given structure (e.g. Ayalew & Ochonogor, Citation2015; Cooper, Underwood, Hilley, & Klymkowsky, Citation2012; Kozma, Citation2003; Parchmann, Broman, Busker, & Rudnik, Citation2015; Shane & Bodner, Citation2006; Stains & Talanquer, Citation2007; Stieff, Citation2007). Students tend to approach molecular structures by emphasising disconnected pieces (Kozma & Russell, Citation1997; Parchmann et al., Citation2015). Parchmann et al. (Citation2015) examined how chemistry students approach a chemical structure in order to solve a problem compared to expert chemists. The students concentrated only on small parts of the complex structure in question, namely the different functional groups. The expert chemists, on the other hand, approached the problem by first considering the molecule as a whole. Then, they went on to scrutinise the relevant functional groups, discussing their connections and arrangements while still maintaining a holistic view in relation to these parts.
Considering this complexity, an interesting question is how students early at the undergraduate level are supposed to make necessary distinctions such as identifying metal-ligands and functional groups, or dealing with slightly small differences in electronegativity between atoms, when encountering new and complex molecules. In encountering a molecular structure appearing to students as consisting of many puzzling and disconnected parts, it may be an insuperable task to see which parts to distinguish, at which stage in the process, and how these parts may be related to each other. It is therefore important to have detailed understanding of how we may support students in learning to make relevant distinctions concerning the parts and wholes of chemical structures. In this study, we address this issue with a particular focus on how a tutor may help students deal with complex molecules for determining oxidation states.
Relationship between distinctions and purposes of a practice
Goodwin (Citation1994, Citation1997) showed that discerning relevant features of a problem involves particular ways of approaching and analyzing the problem that are consistent with the discourse of a particular practice. Moreover, he emphasised the role of experts for the socialisation of students into the discourse in order to become competent participants in the practice. For example, when graduate chemistry students were learning to make relevant distinctions concerning the specific tone of ‘jet black’ in a chemical reaction, this involved deploying organised work practices in the laboratory through an interaction with a competent practitioner, i.e. the tutor (Goodwin, Citation1997). Moreover, these distinctions were particular to the specific activity as a diagnostic sign for reaching a certain desired chemical reaction. This indicates that the particular activity and its purposes provide the framework for deciding which distinctions to be considered relevant and correct for the particular task and the specific practice (cf. also Wickman, Citation2004). Thus, there seems to be a close connection between which distinctions are relevant and which purposes are pursued in an activity.
Indeed, students in interaction with experts, learn to act in ways which are in line with the practice of chemistry. However, students’ learning at the undergraduate level is commonly realised, not only in interaction with an expert, but also in specifically designed learning and teaching activities with specific learning goals. Thus, in these settings tasks, problems, and activities are invoked, organised and operated primarily for educational purposes, thus constituting activities which experts normally do not engage in (other than as educators). In particular, teaching and learning activities are characterised by involving two kinds, or levels, of purposes, those that are directly connected to what students are supposed to do in the moment and those connected to what the students are supposed to eventually learn from the teaching activity (Johansson & Wickman, Citation2011, Citation2018; Wickman & Ligozat, Citation2011; see also Millar, Tiberghien, & Le Marechal, Citation2002; Sensevy, Schubauer-Leoni, Mercier, Ligozat, & Perrot, Citation2005). Moreover, there is a close connection between learning to make distinctions and doing it in relation to specific purposes (e.g. Hamza & Wickman, Citation2013). Therefore, an important challenge for a tutor is to provide support for the students concerning how the purposes pursued in a particular moment when dealing with a chemical problem, in this case the assignment of oxidation states to molecules, are connected to the distinctions made in these situations (Hamza & Wickman, Citation2013; Wickman & Ligozat, Citation2011). The aim of this study is to examine how a tutor may provide support and guidance to the students to make relevant distinctions in relation to different purposes. We address the following research questions:
What distinctions and purposes are invoked by the tutor for guiding the students to determine oxidation states of atoms in molecules?
How are the distinctions made by the tutor and the students related to the different purposes pursued during the activity?
In what ways do the distinctions and the related purposes invoked by the tutor enable or hinder students to determine oxidation states of atoms in molecules?
Methodology
Study context
The study was conducted in the introductory chemistry course at a university in a large city in Sweden. This first chemistry course provides students with a broad introduction to chemistry, encompassing physical, organic, inorganic, and biochemistry. It is divided into four main modules: equilibrium, structural chemistry, reactivity, and biochemistry. Each module has a lecture series linked to laboratory activities and problem-solving classes. The laboratory activities are mandatory whereas lectures and problem-solving classes are optional. In problem-solving classes, the students have the opportunity to engage in an interactive environment with other students and a tutor when solving chemical problems. The problems are usually provided to students in a handout and concern tasks such as calculating oxidation states, explaining chemical concepts introduced in lectures, drawing resonance forms of chemical compounds, and discussing reactivity of molecules.
In the present study, data was drawn from the structural chemistry module. During the data collection, the students were discussing problems related to the topic of chemical bonding and structure and particularly, Lewis structures, resonance structures and hybridisation as well as key concepts such as oxidation state, formal charge, octet rule, and electronegativity.
Data collection
The original data used in this study was derived from eight sessions of problem-solving classes in the structural chemistry module and reactivity module. Each session was between 2 and 2.5 h. Ten students in total, who agreed to participate, were audio and video recorded in an authentic classroom environment during two problem-solving classes, one from each module. During these classes, the students were engaged in solving problems and questions provided in a handout. Moreover, a tutor was available during these classes. Tutors get basic preparation by taking a course in general university pedagogy. The tutor involved in the module of structural chemistry has long experience of being a tutor in this course and holds a doctoral degree in materials chemistry. In the reactivity module two tutors who were doctoral students in organic chemistry, were available. The role of the tutor in the problem-solving classes was mainly facilitative, involving providing support to the students, answering questions related to the problems in the handout, and helping the students by clarifying content introduced in previous lectures if needed. Materials available to the students during these classes were a book in general chemistry (Burrows et al., Citation2013) and the Nuffield advanced Science: Book of data. The Book of data includes physics and chemistry data suitable for all A Level Physics and Chemistry students.
The video recordings were transcribed verbatim. As the interest in this study was to examine how the tutor helped students determine the oxidation states of atoms in a complex molecular structure, and how distinctions and purposes were noticed and dealt with during the interaction, we identified sequences in the transcripts with discussions between students and the tutor. The analysis of these sequences was done in the original language (Swedish) in order to avoid loss of meaning of what is said by the students and the tutor and thereby to enhance the validity of the analysis. The sequence eventually chosen as the case to be presented in the results (see below) was finally translated into English by the first author and, then, checked by the three co-authors to ensure the accuracy of the translation.
Data analysis
In this study, we sought to provide detailed analyses of tutor-student interactions during the activity of determining oxidation states of atoms in complex molecular structures with a particular focus on what distinctions the students and the tutor made when dealing with the task and how these distinctions were related to the different purposes dealt with during the task. The basic unit analyzed, then, constitutes the tutor’s and students’ actions embedded in the specific settings of the activity. To this end, Practical epistemology analysis, PEA (Wickman & Östman, Citation2002) was used. PEA enables a detailed analysis of how actions and purposes, including talk, interact in an activity and what consequences this has for the participants’ possibilities to proceed in the activity towards its purpose which is determining oxidation states of atoms in molecules. This analytical tool has been extensively used to study how distinctions and purposes constitute central aspects of learning in action in the science classroom (Almqvist & Östman, Citation2006; Anderhag, Hamza, & Wickman, Citation2015; Hamza, Citation2013; Hamza & Wickman, Citation2013; Johansson & Wickman, Citation2011; Kelly, McDonald, & Wickman, Citation2012).
In this study, analyzing the purposes pursued and the related distinctions was done by using the four concepts of PEA, namely, gap, relation, stand fast, and encounter. The analysis was conducted in the following way:
The first step of the analysis was to identify the purposes through the gaps that the students or the tutor noticed during the activity. A gap is an operationalisation of a need for the participants to make something intelligible in order to deal with the task and go on with the activity. Thus, gaps reflect the immediate purpose pursued by the participants, that is, what they are engaged in at a particular moment.
Gaps are continually filled with relations to what stands fast to the participants, that is, previous knowledge and experiences employed to deal with the gap. The need to establish relations to fill gaps occurs in response to encounters, which can be with instructions, peers, or various material conditions. The second step is to identify distinctions through the relations in order to deal with the different purposes. These distinctions concern different aspects of the activity, for instance distinctions between the different parts of the molecular structure or between the general and specific parts of the molecule.
The third step of the analysis examines whether these distinctions directed the activity towards or away from the main purpose and accordingly whether these purposes and the related distinctions enabled or constrained students in dealing with the task. Taking the simple example of the molecule NH4+, if the purpose is to determine the oxidation state of nitrogen in the molecule (step 1) it can be fulfilled by discerning hydrogen from the molecule, provided that its oxidation state stands fast to the students (step 2). Based on this distinction, the oxidation state of nitrogen can be determined (step 3).
The three analytical steps were initially conducted by the first author on different sequences. The process involved identifying distinctions and related purposes as well as if these distinctions and purposes helped dealing with the task of determining the oxidation states of atoms in molecules, as outlined above (step 1–3). One long sequence (the selected case presented in the findings) was then carefully scrutinised together with the co-authors through several discussion sessions, in which distinctions and related purposes were went through and checked for agreement. This particular case was originally chosen by the first author because it included typical issues that were partly seen also in other sequences concerning other molecules. In other words, the sequence has the convenient feature of including all the aspects of interest to this study which we observed also in the other sequences analyzed. Therefore, it was particularly rich enabling us to illustrate the multiple interrelations of the specific phenomenon of dealing with the problem of determining oxidation states of atoms in molecules with the guidance of a tutor. The representativeness of the case was continually checked against the other sequences in the joint analysis sessions between the four authors. In the presented case, the students were given pseudonyms to protect their identity.
The discussion sessions between the coauthors during the analytical phase enabled enhancing the trustworthiness of the study. These sessions gave the chance to examine and reexamine the transcripts and engage in discussions about our interpretations for reaching an agreement. This, in turn, reduced researcher bias and enhanced the consistency throughout the analytical process. Moreover, the strength of the analytical approach used in this study which involves the thick and rich description and examination, produced a detailed understanding of the features under investigation (Bryman, Citation2012).
The case: an ongoing process of shifting between parts and wholes
In the presented case we show how students with the guidance of the tutor noticed and discerned aspects that were important for determining the oxidation states of the different atoms in the molecule of . First, the students approached the task with distinctions that were regarded as given. Second, additional distinctions were made between parts and wholes of the molecule in the course of dealing with the task. This movement between the parts and wholes we termed ‘zooming in or out’ of the molecule. Third, this ongoing shift between parts and wholes was connected to concomitant shifts in purposes pursued by the students and the tutor.
Background to the case
The problem to be solved was determining the oxidation states of all atoms in the given molecules. Most of these molecules included either oxygen, hydrogen or both (,
,
,
, HF,
, H2O2). The students had learned a set of guidelines for assigning the oxidation states, among others that the oxidation state of hydrogen is +1 and that of oxygen usually −2. By initially discerning oxygen and hydrogen atoms, and then assigning their given oxidation states, the students could subsequently determine the oxidation states also of the other atoms in a given molecule. In such cases, the task was manageable for the students. To illustrate this we present a short sequence where two students determined the oxidation states of the different atoms in the molecule of
.
The gap that the students noticed and were trying to fill here concerns what oxidation states they should assign to the different atoms in the molecule, and thus concerned the main purpose of the activity. The students started by discerning oxygen and hydrogen from the rest of the molecule. Thus, it here stood fast that this distinction is the first to be made when assigning oxidation states. Since also the oxidation states of these atoms stood fast, oxygen was immediately assigned an oxidation state of −2 and hydrogen +1 [Line 1]. Based on these distinctions the students could thereafter first determine the overall charge of the molecule (the relation ‘It’s negative there’, Line 2) and then rather easily calculate the unknown oxidation state of sulfur. The sequence thus shows that when certain distinctions stood fast, they aided the students in determining the unknown oxidation state of the remaining, unknown atoms in a molecule.
Results
When hydrogen and oxygen were absent from the molecule the students had significantly more trouble in accomplishing the task. In the case presented here, the three students Elvin, Robin and Stella faced challenges in accomplishing the task of determining the oxidation states of the different atoms in the molecule , as they could not discern the atoms of hydrogen and/or oxygen in the molecule. Therefore, the students reached out to the tutor (TA) for help. As we will show, the ensuing interaction was characterised by distinctions which moved the focus of the discussion in and out of the molecule, as well as by concomitant shifts concerning which purposes that were pursued by the students and the tutor.
Overall (summarised in ), our analysis showed that the tutor played an important role in guiding, and sometimes misguiding, the activity by invoking diverse distinctions that entailed ongoing shifts in focus of zooming in and out on the different parts of the molecule. These distinctions served different purposes pursued by the tutor and/or the students during the activity. Some purposes were directly related to the problem while others served more general learning of the subject matter studied. As some of the purposes, for general learning in particular, were not clearly communicated to the students or as the tutor did not connect them to the task in a productive way, they had negative consequences on dealing with the task and developing students’ learning.
Figure 1. A summary of the shifts in purposes followed by shifts between wholes and parts across the seven sequences. Purposes related to the main task are labelled ‘M’ and purposes for general learning are labelled ‘G’. Purposes and distinctions invoked by the tutor appears in bold text. The arrows in the figure represent the zooming in and out on the different parts of the molecule.
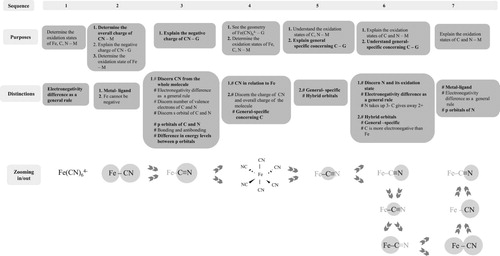
To make these features of the analysis easier to follow, we divided the presentation of the interaction into seven sequences illustrating this process of guiding the students in making distinctions and shifting purposes for accomplishing the task. Each sequence illustrates a certain shift in focus, concerning zooming in or out of the molecule and in relation to certain purposes pursued. Thus, the division into seven sequences primarily serves a pedagogical function and should not be considered as definite demarcations of what was, of course, a continuous flow of tutor-student interaction.
Sequence 1: electronegativity difference as a general rule
The students initially approached the tutor with a query connected to the main purpose of the activity, that is, to determine the oxidation states of atoms in a molecular structure. In particular, here the students worked with how to determine the oxidation states of the different atoms in the complex molecule .
As hydrogen and oxygen were not part of the molecule, a gap of how to determine the oxidation states of the different atoms emerged [Line 1]. The tutor’s first action to help the students fill this gap was by initially discerning each atom in the molecule and then determine the electronegativity values of the different atoms involved (). This distinction is general in the sense that the difference between the electronegativity values of the atoms helps determine which atoms attract electrons in the molecule and, accordingly, tell about the oxidation states.
Sequence 2: zoom in on central atom-ligand
The tutor’s next action was to introduce another distinction which also entailed a shift in focus by zooming in on the parts of the molecule. This involved discerning the central atom from the ligands (where Fe is the central atom and CN is the ligand) and, consequently, zooming in on the ligand (CN) of the molecule for the purpose of determining its overall charge ().
Table
The distinction invoked by the tutor in Lines 14 and 16 was a step towards determining the oxidation states of the atoms, that is, it was still part of filling the gap concerning the main purpose of the activity. Accordingly, Robin responded directly by noting that CN has a negative charge. However, the tutor did not settle with discerning the overall charge of CN being −1, and pursuing the main purpose. Instead, he introduced an additional purpose by noticing the gap ‘ … why is that?’ [Line 18]. This gap did not deal directly with the main purpose of determining the oxidation states of the atoms in the molecule, but was more general in character, asking for an understanding of the negative charge of CN. This new, general purpose invoked by the tutor, in turn, evoked yet another shift in focus concerning the parts of the molecule, that is from CN to Fe, ‘Since iron can hardly be minus’ [Line 20]. This time, the shift in focus came from the students as they had trouble filling the gap of why CN is negative. Although reinforced by the tutor [Line 22 and 24], this shift in focus did not end in the students filling the gap. Instead, the discussion returned to the distinctions of metal–ligand complex. As they understood that CN as a ligand has an overall charge of −1, the discussion also shifted from the general purpose invoked by the tutor in Line 18 back to the main purpose of the activity, that is, determining the oxidation state of the atoms [Line 25–29].
This sequence shows how the tutor zoomed in on CN for the purpose to determine its charge as a whole. This purpose was a step towards the main purpose of the activity. However, the additional, general purpose of understanding why CN has a negative charge did not directly connect to the task, and the students accordingly had trouble filling the gap with working relations. Consequently, the students eventually shifted the discussion back to the main purpose of the task of determining the oxidation states of the individual atoms. This shift in purpose, moreover, entailed zooming in on Fe instead of on CN.
Sequence 3: zooming in further on CN and discerning its parts
However, the tutor persisted in pursuing the purpose of explaining the negative charge of CN as a whole. This shift in purpose had consequences on the activity as further distinctions were needed.
As the tutor returned to the general purpose concerning the negative charge of CN (seen through the gap ‘Why is CN minus?’, Line 31), yet another distinction, and concomitant shift in focus, was made, namely zooming in further, from CN as a whole to its individual parts (). As a start, Robin noted the difference between the electronegativity values of nitrogen and carbon [Line 32]. This was in regard to the general rule of electronegativity difference introduced by the tutor in sequence 1. This was followed by the difference between the number of valence electrons of carbon and nitrogen [Line 35]. In line with these two distinctions and the relation in line 36 the tutor encouraged the students to draw molecular orbital diagram (MO diagram) for the purpose to explain the negative charge of CN as a whole.
It is important to note that the distinctions made for dealing with the general purpose of ‘why is CN minus?’ were actually relevant distinctions for accomplishing the main purpose of the activity. However, this was not communicated to the students. Instead, the tutor proceeded by encouraging the students to draw MO diagram, which he needed to guide the students through. Accordingly, further distinctions were made along with the distinction in Lines 35, which was regarded as given.
As a start, TA made a new distinction between the p orbitals of carbon and the p orbitals of nitrogen in the MO diagram [Line 41]. This distinction evoked another distinction between bonding and antibonding orbitals concerning their energy levels [Line 42]. However, Stella overlooked the energy difference between px, py and pz which, then, was noticed by the tutor [Line 43 and 45]. Consequently the gap ‘How do we know that?’ [Line 47] emerged. This gap did not directly deal with the purpose of drawing MO diagram but rather the students asked for an understanding of the energy difference between the three p orbitals. As the gap was filled with working relations [Lines 49–52] Stella proceeded by distinguishing between the antibonding p orbitals that corresponded to the earlier distinction invoked in lines 43 and 45. Lastly, she filled the molecular orbitals [Line 59] with the aid of an additional distinction made earlier, namely the difference between the number of electrons of carbon and nitrogen that was regarded as given [Lines 55 and 57]. As the purpose of drawing the MO diagram of CN was completed, a shift back to the purpose of why CN has a charge of −1 was made. The tutor discerned the empty place from the other occupied orbitals, which, in turn, explained the negative charge of CN as a whole.
Figure 2. Simplified version of MO diagrams of CN drawn by the students, including the valence p orbitals (s orbitals are not shown). On the left, the general MO diagram with the molecular orbitals in the same energy level. On the right the completed MO diagram.

This sequence shows that the distinctions made for guiding the students to draw the MO diagram helped dealing with the purpose of explaining the negative charge of the ligand. Moreover, this purpose was clearly communicated by the tutor which may explain the success in accomplishing it.
Sequence 4: zooming out to deal with the molecule as a whole
Once the gap of why CN has a charge of −1 was filled, the discussion shifted back to the main purpose of the activity (). However, this was accomplished through a new more general purpose first being initiated by the students, namely how CN is attached to Fe.
Through the gap that emerged in Line 67, Robin shifted the purpose towards a need for understanding the relationship between CN and Fe in the molecule. This general purpose, moreover, was connected to a shift in focus by zooming out to view the relationship between the parts of the molecule (). In order to fill the gap, the tutor explained the relationship between the different parts of the molecule, viz. the six CN ligands and particularly their position in space in relation to iron [Line 68]. Then, Stella recalled the main purpose of the task which was determining the oxidation states of the individual atoms [Line 71]. Accordingly, Robin highlighted and specified earlier distinctions that were regarded as given for determining the oxidation states of the atoms [Line 72]. This led to the emergence of the gap ‘why is it −1?’ [Line 73]. Likely the negative charge of CN as a whole became unintelligible through the shift from the part of CN (sequence 3) to viewing the part-whole relationship. Moreover, this gap shows that the tutor’s purpose of helping students understand the negative charge of CN by drawing molecular orbital diagram, although successful in the context of sequence 3, was not helping the students when there had been a shift in focus of the molecule.
The sequence ends with the tutor invoking a new distinction between the general and the specific concerning the molecule of . Generally this molecule is an example of a coordination complex with metal in the centre surrounded by ligands and specifically here, an iron atom in the centre surrounded by cyanide ions as ligands [Line 80]. This distinction was important in the sense that it clarified the view of whole-part relationship in an anticipated way.
This sequence shows that the tutor’s guidance in shifting the focus by zooming out for the purpose to see the molecule as a whole was an important step towards completing the main purpose of the task. This shift entailed important distinctions such as between the general and the specific for understanding the part-whole relationship. However, it also had negative consequences since Stella could not maintain the view of the parts. This, in turn, raises the issue of whether drawing the molecular orbital diagram in the prior sequence was successful or necessary for that matter, for filling the gap of why CN has a negative charge.
Sequence 5: zooming in on carbon individually
As the gap concerning the relationship between the different atoms in the molecule was filled, the students shifted back to the main purpose of the task. This evoked yet more distinctions invoked by the tutor.
In response to the gap concerning the oxidation state of carbon and nitrogen in the molecule [Line 82] introduced by the students, another shift in focus was made by the tutor. This shift entailed zooming in further on carbon individually (). To be precise, the students shifted purpose from the main purpose to a more general one, in particular, from determining the oxidation state of carbon and nitrogen to acquiring an understanding of these oxidation states. In order to fill the gap, the tutor invoked a new distinction between the general and the specific for carbon, thereby also introducing yet another general purpose. Carbon commonly has an oxidation state of +4 which means it forms four bonds and in this specific molecule ‘three go to nitrogen and one to iron’ [Line 83]. Consequently the gap reemerged [Line 84]. The tutor, then, invoked the hybrid orbitals for carbon as a way of reinforcing earlier distinctions [Line 87]. Yet, the original gap introduced by the students reemerged once again [Line 88]. It seems as if the distinctions made by the tutor were not useful to the students, as they did not address the general purpose concerning the oxidation state of carbon pursued by the students, but rather concerned another general purpose of understanding the general in relation to the specific concerning carbon. Put differently, the students’ purpose did not agree with the tutor’s purpose which resulted in a lingering gap.
The sequence shows that the distinctions invoked by the tutor, concerning the general and the specific were not helpful in accomplishing the main purpose of the task but rather created confusion. Since this purpose was not obvious to the students, the tutor did not succeed in supporting the students to accomplish it. Moreover, the tutor’s choice to relate to the hybrid orbitals may not even be necessary, or indeed relevant, for fulfilling the purpose concerning the general and the specific for carbon.
Sequence 6: zooming out on CN
However, as the students still pursued the purpose of explaining the oxidation state of carbon and nitrogen, additional new distinctions along with earlier distinctions were made.
As the students were still pursuing the same purpose (seen through the gap in line 88) further distinctions were made followed by another shift in focus, namely zooming out on CN and its parts (). The tutor initiated this shift by discerning nitrogen and its oxidation state, which was regarded as given [Line 89]. This, in turn, aided Stella in the relation that carbon gives away two electrons [Line 90]. Then, as a reinforcement, the tutor recalled the difference between the electronegativity values of carbon and nitrogen [Lines 93 and 95] as a general rule for determining which atom has a negative oxidation state. Consequently, Elvin established that nitrogen takes up three so it’s 3- then carbon leaves two so it’s 2+ [Line 98–100]. Here, the tutor could have guided the students towards the main purpose of the task. Instead, the tutor pursued a more general purpose of understanding how carbon may behave differently than expected under certain circumstances, as he added that carbon must give away four electrons by recalling two earlier distinctions first, concerning the hybridised orbitals [Line 101] and second what is general and what is specific for carbon [Line 108]. As a result an uncertainty and confusion concerning the oxidation state of carbon in the molecule emerged [Line 103 and 105] which evoked a new distinction between the electronegativity values of carbon and iron: ‘But carbon is more electronegative than iron' [Line 109]. This distinction is incorrect in regard to the part-whole relationship for determining the oxidation states in this molecule that the tutor tried to communicate to the students in the previous sequences. Likely, it was a consequence of the unclear purpose of zooming in on carbon pursued by the tutor.
This sequence shows that whereas the students were pursuing the purpose concerning the oxidation state of carbon and nitrogen in this specific molecule, the tutor persisted with the additional, more general purpose pursued in the previous sequence, that is, understanding what is general and what is specific for the molecule. Since the tutor was not clear concerning this purpose, the invoked distinctions and the zooming in were not useful but rather created confusion for the students.
Sequence 7: zooming both ways
In the last sequence the tutor recapitulated the main distinctions invoked in the previous sequences which entailed several shifts in focus for explaining the oxidation states of carbon and nitrogen.
As a consequence of the gap concerning the oxidation state of carbon (seen through the gap in line 109), several earlier distinctions were highlighted and a shift back in focus was made, namely zooming back out to view the molecule as central atom-ligand with focus on CN as a ligand (). First, the tutor invoked the earlier distinction between parts and wholes, specifically concerning iron-cyanide [Line 110]. Second, he invoked the earlier distinction between the specific and the general concerning the molecule as a whole [Line 112], ending up with the general distinction between the electronegativity values of the atoms involved. [Line 113–116].
Discussion
This study explored how an experienced tutor provided support and guidance to a group of students in determining the oxidation states of atoms in a complex molecule by means of making relevant distinctions and how these distinctions were connected to the different purposes pursued during the activity. Similar to other studies (e.g. Kozma, Citation2003; Parchmann et al., Citation2015), the results showed that the students struggled with making distinctions of the relevant parts of the molecular structure for solving the problem at hand. Our students had trouble in identifying the part-whole relationships (e.g. metal-ligand) in the given molecule for solving the problem, in contrast to the tutor’s ability (as an expert) to connect to a wide range of structures that he had encountered and was familiar with due to long experience in the chemistry discipline (Kozma & Russell, Citation1997). However, our results demonstrate that learning to identify equivalencies to other familiar molecules (e.g. coordination complexes), how to discern the relevant parts of the molecule and what is general and specific, is closely tied to the understanding of the different purposes pursued during the activity.
In order to determine oxidation states of atoms in complex molecules such as metals in metal complexes, Steinborn (Citation2004) suggested subtracting the charge of the complex from the sum of the charges of all ligands. Indeed, this knowledge is important for supporting the process of determining oxidation states of metals in metal complexes, assuming that students can make the initial distinction of metal-ligand. However, our results show that students had trouble making such a distinction. To draw on this, determining oxidation states of different carbon atoms in organic molecules, such as carbonyl carbon and methyl carbon (Halkides, Citation2000), can also be problematic. Particularly, early at the undergraduate level, students may struggle discerning the functional groups and also with increasing complexity of molecules.
Our results also show how the tutor guided the students to make relevant distinctions by shifting focus on the relevant parts in relation to the different kinds of purposes (e.g. Johansson & Wickman, Citation2011). Part of these purposes were undoubtedly necessary, as they constituted steps towards the completion of the task which the tutor occasionally did well in guiding the students. However, in addition to supporting the students in pursuing this main purpose, the tutor also invoked other purposes aiming towards more general learning of the subject matter. This was sometimes problematic. The tutor was not always clear with these purposes and consequently the several distinctions invoked by him were unproductive and even misled the students during the activity. Moreover, sometimes, these general purposes were invoked during the process in such a way that the tutor had not only trouble helping the students to understand but also hindered further dealing with the main purpose. As a result, the students could not always follow the guidance of the tutor in the process of zooming in and out.
It could be argued that some of the general purposes invoked by the tutor were not only unproductive for dealing with the task but sometimes irrelevant or even incorrect in relation to the task (e.g. the invocation of MO diagrams to explain why CN− is negative). However, tutors and teachers need to make many in-the-moment decisions in the classroom, including which purposes are important to include in an activity and which to hold on to until later. These in-the-moment decisions are made from a complex set of knowledge and experiences, such as the tutors’ knowledge of the chemistry content, knowledge of pedagogy and their practical and situated knowledge of individual learners. Consequently, some purposes invoked might be considered helpful in the moment, although they may be realised to be incorrect or simply not make sense afterwards, on reflection. Our case seems to have captured one or two examples of this. However, we believe that analyses such as those presented here help drawing attention to such occasions, thereby offering practicing tutors and teachers opportunities to reflect on and critically evaluate their decisions which, accordingly, might lead to more thoughtful and deliberate decisions in future teaching activities.
Implications
The tutor obviously has an important role in guiding students through the process of solving problems related to complex molecules, and in particular making relevant distinctions of molecular structures in relation to different purposes. How, then, can the support and guidance provided by the tutor become more productive? First, we need to consider that solving problems related to complex molecular structures, that is, determining oxidation states of atoms in this study, is a matter of appropriating a discursive competence of discerning relevant and important parts of a problem (e.g. Goodwin, Citation1997). Moreover, it involves incessantly zooming in and out on the different parts of a molecular structure without losing the details during the process of shifting and more importantly in relation to the purposes pursued. In order to make this process more manageable for the students, tutors may need to consider what general purposes are important to include (or exclude for that matter) and how to sequence, move between, and productively connect these different purposes (e.g. Johansson & Wickman, Citation2011). One suggestion could be that the tutor may start with pursuing the main purpose directly connected to the specific task in order to minimise confusion among students. Then, additional purposes could be introduced for potentially developing students’ learning of more general content. By doing so, tutors may be able to provide students with productive purposes for both solving the specific task and further developing students’ learning of the subject matter studied. This increased alignment between purposes and invoked distinctions may allow students to struggle less with following the ongoing shifts in focus of zooming in and out of complex molecular structures. Moreover, new purposes that are introduced and pursued by the tutor should be explicitly communicated during the process to make sure that students follow the concomitant shifts in focus of zooming in an out on the different parts of a molecular structure. In particular, purposes for general learning should be clear since they provide students with opportunities to connect the task to the subject matter studied.
Teaching and learning to make relevant distinctions in relation to purposes is important and useful also to other tasks such as drawing resonance forms and determining aromaticity and reactivity of organic molecules. In these tasks, distinctions need to be made for keeping track on electrons in the molecule in relation to different purposes such as checking for not exceeding the octet rule, achieving minimised formal charge and form a valid Lewis structure. We believe that analyzing tutor-student interaction can be beneficial and constitute a useful preparation and practice for tutors as it encourages them to reflect on how they guide and support students to learn to make distinctions of molecular structures and to what purposes in order for this interaction to be more productive.
Finally, the results of the analysis of tutor-student interaction may complement other studies (e.g. Calzaferri, Citation1999; Halkides, Citation2000; Jurowski et al., Citation2015) for determining oxidation states of atoms in complex molecules. The results also suggest more thoughtful and deliberate ways for dealing with the different purposes that exists also within the chemistry classroom in general. Such analysis can be used in research in chemistry education to examine students’ patterns in dealing with different tasks in chemistry in relation to different purposes and more importantly, future studies may examine the impact of purposes invoked by tutors and teachers. Moreover, based on the evaluation of purposes invoked, further research may suggest improvements of learning and teaching practices by reconstructing learning activities for invoking useful and productive purposes, both purposes directly related to the task and purposes for general learning.
Disclosure statement
No potential conflict of interest was reported by the authors.
ORCID
Ilana A. Manneh http://orcid.org/0000-0003-0712-0681
Karim M. Hamza http://orcid.org/0000-0003-4051-3698
References
- Almqvist, J., & Östman, L. (2006). Privileging and artifacts: On the use of information technology in science education. Interchange, 37(3), 225–250.
- Anderhag, P., Hamza, K. M., & Wickman, P.-O. (2015). What can a teacher do to support students’ interest in science? A study of the constitution of taste in a science classroom. Research in Science Education, 45(5), 749–784. doi: 10.1007/s11165-014-9448-4
- Atkins, P. W., & Beran, J. A. (1992). General chemistry (2nd ed.). New York, NY: Freeman.
- Atkins, P. W., & Jones, L. L. (2005). Chemical principles: The quest of insight (3rd ed.). New York, NY: Freeman.
- Ayalew, T., & Ochonogor, C. E. (2015). Assessment of undergraduate chemistry students’ difficulties in organic chemistry. ISTE international conference proceedings, Unisa Press, pp. 285–296.
- Bentley, R., Franzen, J., & Chasteen, T. G. (2002). Oxidation numbers in the study of metabolism. Journal of Biochemistry and Molecular Biology Education, 30(5), 288–292. doi: 10.1002/bmb.2002.494030050114
- Birk, J. P. (1992). The computer as student: An application of artificial intelligence. Journal of Chemical Education, 69(4), 294–295. doi: 10.1021/ed069p294
- Brandriet, A. R., & Bretz, S. L. (2014). Measuring meta-ignorance through the lens of confidence: Examining students’ redox misconceptions about oxidation numbers, charge, and electron transfer. Journal of Chemistry Education Research and Practice, 15(4), 729–746. doi: 10.1039/C4RP00129J
- Bryman, A. (2012). Social research methods (4th ed.). New York, NY: Oxford University Press.
- Burrows, A., Holman, J., Parsons, A., Pilling, G., & Price, G. (2013). Chemistry3: Introducing inorganic, organic and physical chemistry (2nd ed.). Oxford: Oxford University Press.
- Calzaferri, G. (1999). Oxidation numbers. Journal of Chemical Education, 76(3), 362–363. doi: 10.1021/ed076p362
- Cooper, M. M., Underwood, S. M., Hilley, C. Z., & Klymkowsky, M. W. (2012). Development and assessment of a molecular structure and properties learning progression. Journal of Chemical Education, 89(11), 1351–1357. doi: 10.1021/ed300083a
- de Jong, O., Acampo, J., & Verdonk, A. (1995). Problems in teaching the topic of redox reactions: Actions and conceptions of chemistry teachers. Journal of Research in Science Teaching, 32(10), 1097–1110. doi: 10.1002/tea.3660321008
- de Jong, O., & Treagust, D. (2002). The teaching and learning of electrochemistry. In J. K. Gilbert, O. de Jong, R. Justi, D. F. Treagust, & J. H. van Driel (Eds.), Chemical education: Towards research-based practice (pp. 317–337). Dordrecht: Kluwer Academic Publishers.
- Eggert, A. A., Middlecamp, C. H., & Kean, E. (1990). An oxidation number assignment expert for CHEMPROF. Journal of Chemical Information and Computer Sciences, 30(2), 181–187.
- Garnett, P. J., & Treagust, D. F. (1992). Conceptual difficulties experienced by senior high school students of electrochemistry: Electric circuits and oxidation-reduction equations. Journal of Research in Science Teaching, 29(2), 121–142. doi: 10.1002/tea.3660290204
- Goodwin, C. (1994). Professional vision. American Anthropologist, 96(3), 606–633. doi: 10.1525/aa.1994.96.3.02a00100
- Goodwin, C. (1997). The blackness of black: Color categories as situated practice. In L. B. Resnick, R. Säljö, C. Pontecorvo, & B. Burge (Eds.), Discourse, tools and reasoning: Essays on situated cognition (pp. 111–140). Berlin: Springer-Verlag.
- Gupta, V., Ganegoda, H., Engelhard, M. H., Terry, J., & Linford, M. R. (2014). Assigning oxidation states to organic compounds via predictions from X-ray photoelectron spectroscopy: A discussion of approaches and recommended improvements. Journal of Chemical Education, 91(2), 232–238. doi: 10.1021/ed400401c
- Halkides, C. (2000). Assigning and using oxidation numbers in biochemistry lecture courses. Journal of Chemical Education, 77(11), 1428–1432. doi: 10.1021/ed077p1428
- Hamza, K. M. (2013). Distractions in the school science laboratory. Research in Science Education, 43(4), 1477–1499. doi: 10.1007/s11165-012-9316-z
- Hamza, K. M., & Wickman, P.-O. (2013). Supporting students’ progression in science: Continuity between the particular, the contingent, and the general. Science Education, 97(1), 113–138. doi: 10.1002/sce.21042
- Holleran, E. M., & Jespersen, N. D. (1980). Elementary oxidation-number rules. Journal of Chemical Education, 57(9), 670. doi: 10.1021/ed057p670.1
- Jensen, W. B. (2011). Oxidation states versus oxidation numbers. Journal of Chemical Education, 88(12), 1599–1600. doi: 10.1021/ed2001347
- Johansson A.-M., & Wickman P.-O. (2011). A pragmatist understanding of learning progressions. In B. Hudson & M. A. Meyer (red.), Beyond fragmentation: Didactics, learning and teaching in Europe ( s. 47–59). Leverkusen: Barbara Budrich Publishers.
- Johansson, A.-M., & Wickman, P.-O. (2018). The use of organizing purposes in science instruction as a scaffolding mechanism to support progressions: A study of talk in two primary science classrooms. Research in Science & Technological Education, 36(1), 1–16. doi: 10.1080/02635143.2017.1318272
- Jørgensen, C. K. (1969). Oxidation Numbers and Oxidation States. Heidelberg: Springer.
- Jurowski, K., Krzeczkowska, M. K., & Jurowska, A. (2015). Approaches to determining the oxidation state of nitrogen and carbon atoms in organic compounds for high school students. Journal of Chemical Education, 92(10), 1645–1652. doi: 10.1021/ed500645v
- Kauffman, J. M. (1986). Simple method for determination of oxidation numbers of atoms in compounds. Journal of Chemical Education, 63(6), 474–475. doi: 10.1021/ed063p474
- Kelly, G. J., McDonald, S., & Wickman, P.-O. (2012). Science learning and epistemology. In B. J. Fraser, K. Tobin, & C. J. McRobbie (Eds.), Second international handbook of science education (pp. 281–291). Heidelberg: Springer.
- Kozma, R. (2003). The material features of multiple representations and their cognitive and social affordances for science understanding. Learning and Instruction, 13(2), 205–226. doi: 10.1016/S0959-4752(02)00021-X
- Kozma, R., & Russell, J. (1997). Multimedia and understanding: Expert and novice responses to different representations of chemical phenomena. Journal of Research in Science Teaching, 34(9), 949–968. doi: 10.1002/(SICI)1098-2736(199711)34:9<949::AID-TEA7>3.0.CO;2-U
- Loock, H.-P. (2011). Expanded definition of the oxidation state. Journal of Chemical Education, 88(3), 282–283. doi: 10.1021/ed1005213
- Millar, R., Tiberghien, A., & Le Marechal, J.-F. (2002). Varieties of labwork: A way of profiling labwork tasks. In D. Psillos, & H. Niedderer (Eds.), Teaching and learning in the science laboratory (pp. 9–20). Dordrecht: Springer.
- Packer, J. E., & Woodgate, S. D. (1991). Lewis structures, formal charge, and oxidation numbers: A more user-friendly approach. Journal of Chemical Education, 68(6), 456. doi: 10.1021/ed068p456
- Parchmann, I., Broman, K., Busker, M., & Rudnik, J. (2015). Context-based learning on school and university level. In J. Garcia-Martinez & E. Serrano-Torregrosa (Eds.), Chemistry education: Best practices, opportunities and trends (pp. 259–278). Berlin: Wiley-VCH Verlag GmbH.
- Sensevy, G., Schubauer-Leoni, M.-L., Mercier, A., Ligozat, F., & Perrot, G. (2005). An attempt to model the teacher’s action in the mathematics class. Educational Studies in Mathematics, 59(1–3), 153–181. doi: 10.1007/s10649-005-5887-1
- Shane, J. W., & Bodner, G. M. (2006). General chemistry students’ understanding of structure-function relationships. The Chemical Educator, 11(2), 130–137.
- Shibley, I. A., Amaral, K. E., & Aurentz, D. J. (2010). Oxidation and reduction reactions in organic chemistry. Journal of Chemical Education, 87(12), 1351–1354. doi: 10.1021/ed100457z
- Stains, M., & Talanquer, V. (2007). Classification of chemical substances using particulate representations of matter: An analysis of student thinking. International Journal of Science Education, 29(5), 643–661. doi: 10.1080/09500690600931129
- Steinborn, D. (2004). The concept of oxidation states in metal complexes. Journal of Chemical Education, 81(8), 1148–1154. doi: 10.1021/ed081p1148
- Stieff, M. (2007). Mental rotation and diagrammatic reasoning in science. Learning and Instruction, 17(2), 219–234. doi: 10.1016/j.learninstruc.2007.01.012
- Wickman, P.-O. (2004). The practical epistemologies of the classroom: A study of laboratory work. Science Education, 88(3), 325–344. doi: 10.1002/sce.10129
- Wickman, P.-O., & Ligozat, F. (2011). Scientific literacy as action: Consequences for content progression. In C. Linder, L. Östman, D. A. Roberts, P.-O. Wickman, G. Erickson, & A. MacKinnon (Eds.), Exploring the landscape of scientific literacy (pp. 145–159). New York, NY: Routledge.
- Wickman, P. O., & Östman, L. (2002). Learning as discourse change: A sociocultural mechanism. Science Education, 86(5), 601–623. doi: 10.1002/sce.10036
- Woolf, A. A. (1988). Oxidation numbers and their limitations. Journal of Chemical Education, 65(1), 45–46. doi: 10.1021/ed065p45
- Zumdahl, S. S., & Zumdahl, S. A. (2007). Chemistry (7th ed.). Belmont, CA: Brooks Cole.