Abstract
Objective
To evaluate the effects of various initiation time points and durations of hormone therapy (HT) on cardiovascular and metabolic parameters of premenarche, primary ovarian insufficiency (POI) mouse model, induced by 4-vinylcyclohexene diepoxide.
Methods
A total of 50 mice at 4 weeks of age were developed into POI mouse model, further randomly categorized into 5 groups: control group without any intervention; no HT group with only high-fat diet (NT); group 1 with delayed estradiol treatment (T1); group 2 with on-time, continuous estradiol treatment (T2); and group 3 with on-time estradiol treatment but early stop (T3). Cardiovascular risk and metabolic parameters were measured.
Results
Presenting with similar body weights, blood glucose levels of T1, T2, and T3 were all significantly lower than NT (p < .001). Serum total cholesterol and insulin were also significantly lower in all HT groups than in NT, especially in T2 (p < .001). For serum low-density lipoprotein-cholesterol, only T2 resulted in the statically lower level than those of NT, T1, and T3 (p < .001). Aortic thickness was significantly increased with aggravated fibrotic change of the intima in NT, and such consequence was significantly ameliorated in HT groups, mostly lowered in T2 (p < .05). Last, serum pro-inflammatory cytokines were significantly low in the HT groups than in NT, especially in T2 with the lowest level (p < .05).
Conclusions
On-time, continuous E2 treatment immediately after a biologic estrogen deprivation event significantly reduced metabolic and cardiovascular risks in young, pre-menarche female mouse models of POI, confirming decreased serum levels of pro-inflammatory cytokines.
Introduction
Primary ovarian insufficiency (POI) has gained scholarly attention for decades as one of the serious health and social issues conflicting modern women, with its latest global prevalence of approximately 1%; the prevalence of associated genetic disorders seems static, but as more female patients take advantages of life-at-stake therapies such as chemotherapy to survive from cancers, the overall prevalence of POI appears to be increasing [Citation1,Citation2]. POI is commonly diagnosed when a women experiences cessation of menstruation before 40 years of age and her serum follicle-stimulating hormone (FSH) level of menopause; since these women are exposed to estrogen deprivation longer than their age-matched counterparts of natural menopause, they are more prone to menopause-related complications, including metabolic, cardiovascular, musculoskeletal, and neurodegenerative risks [Citation3]. Morbidity and mortality of such health risks are well known to be higher in POI, and estrogen replacement is the keystone of disease management [Citation1,Citation4]. Various international societies have suggested guidelines discussing possible systemic hormone therapy (HT) regimens, according to its long-term benefits and risks; despite in-depth evaluations and analyses, most of their evidence studies are retrospective-nature, with a limited number of intervention or animal studies on underlying pathophysiologic mechanisms [Citation4–6].
In terms of HT effects on cardiovascular and metabolic risks in natural menopause, ground-breaking studies confirming ‘window of opportunity’ or ‘timing hypothesis’ have been rigorously published, affecting international guidelines on HT use in women’s menopause [Citation7–9]. These guidelines agree that HT initiation in women aged younger than 60 years or within 10 years of menopause onset significantly reduces all-cause mortalities, and such protective effect of HT becomes uncertain when such ‘window’ has passed − 10 years from the menopause onset [Citation7–9]. Several theories regarding its underlying mechanism have been suggested, including the estrogen deprivation duration and pathophysiologic changes adapting to exogenous estrogen, especially in cardiovascular risk perspectives [Citation10–13]. In POI, the patients experience an extended duration of estrogen deprivation when the diagnosis is delayed, and sufficient estrogen exposure cannot be achieved when the diagnosis is made before puberty. The concept of ‘timing hypothesis’ is thus needed to be thoroughly investigated in such estrogen-naïve POI patients, and an evidence-based HT regimen with established mechanisms from not only clinical but also animal studies should be developed. Therefore, the current study evaluates the effects of various initiation time points and durations on cardiovascular and metabolic parameters of young POI mouse model before puberty.
Materials and methods
Animals
Three-week-old female C57BL/6 female mice were purchased from the Korea Experimental Animal Center (Daegu, Korea) and were acclimated in a controlled laboratory with room temperature of 22 ± 2 °C, relative humidity of 55 ± 5% and 12-h light and dark cycle for 1 week, located at the animal facility of Korea Institute of Toxicology (Jinju, Gyeongsangnam-do, Republic of Korea). At the time of initiation of the study, the mice were 4-week old. Throughout the study period, the mice were able to access food and water available ad libitum. Body weight was measured at weekly basis, and fasting blood glucose (FBG) at bi-weekly basis. All protocols were approved by the Institutional Animal Care and Use Committee at Korea Institute of Toxicology.
Study design and group classification
The summarized study design and group classification are demonstrated in and . First, in order to induce ovarian failure, mice were weighed and administered via intra-peritoneal (IP) injections of 4-vinylcyclohexene diepoxide (VCD) (V3630; Sigma Aldrich, St Louis, MO, USA), in sesame oil as solvent, at a dose of 160 mg/kg/day using a dosing standard of 2.5 ml/kg/day for the first 15 consecutive days, as previously established [Citation14]. After the establishment of POI mouse model with the 15-day injection period of VCD, serum anti-Müllerian hormone (AMH), and FSH levels were measured, and the results confirmed POI status of the VCD-treated mice, as shown in . Afterward, 10 mice were randomly selected as controls with continuing normal diet and no intervention such as E2 treatment, and the remaining 40 mice were switched from the normal diet to high-fat diet (HFD) as the intervention group in order to exacerbate metabolic and cardiovascular risks along with the estrogen deprivation. Mice in the intervention group were further randomized into four groups: no estradiol treatment group (NT); treatment group 1 with delayed estradiol treatment for 3 weeks (T1); treatment group 2 with on-time estradiol treatment for 6 weeks (T2); treatment group 3 with on-time estradiol treatment lasted for 3 weeks and paused (T3). As the repletion of estradiol, 17β-estradiol (E2; Sigma Aldrich, St. Louis, MO, USA) was dissolved in sesame oil and was administered orally at a dose of 0.2 mg/kg/day according to the study protocol described in and . For NT, 0.1 ml of sesame oil was treated as vehicle control.
Figure 1. Study design and VCD treatment results.
A. Flow-chart visualization of the study group classification.
IP, intra-peritoneal; VCD, 4-vinylcyclohexene diepoxide with 160mg/kg; ND, normal diet; E2, estradiol with 0.2mg/kg; NT, no estradiol treatment; T1, treatment group 1 with delayed estradiol treatment for 3 weeks; T2, treatment group 2 with on-time, continuous estradiol treatment for 6 weeks; T3, treatment group 3 with on-time, short estradiol treatment for 3 weeks; PO, per oral; NS, normal saline 0.9%; HFD, high fat diet.
B. AMH and FSH levels after the completion of VCD treatment.
AMH, anti-Müllerian hormone; FSH, follicle-stimulating hormone; VCD, 4-vinylcyclohexene diepoxide. *P-value <.05 (vs. vehicle).
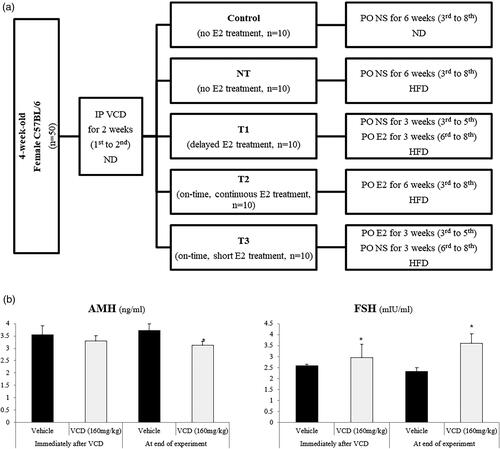
Table 1. Study design and group classification.
Measurement of blood glucose and body weight gain
Mice were fasted for 4 h, and their blood glucose levels were measured by a noninvasive tail-cuff machine (Hatteras Instrument, Inc., Cary, NC, USA) for 0, 2, 5 weeks until the endpoint of the experiment. Body weight gain was measured once a week using an electronic balance (CAS, Yangju, Gyeonggi, Korea) at the fixed time of the day during the experimental period.
Collection of blood and tissue samples
At the end of the experiment, mice were sacrificed using carbon dioxide (CO2). Immediately, blood was collected directly from the heart and placed into plain tubes without anticoagulant to separate the serum. The tubes were centrifuged at 3000 rpm for 10 min, and serum was stored at −20 °C. Subsequently, the excised tissues and organs of the liver, heart, spleen, kidneys, visceral fat, uterus, and ovaries were weighted. Thoracic aorta tissues were removed and perfusion-fixed with 4% paraformaldehyde solution for histology and processed for paraffin embedding.
Histology analyses of the aorta
For histological evaluation, paraffin blocks of the thoracic aorta were cut into 5 μm sections along the axial and transversal artery, stained with hematoxylin and eosin (H&E). The sections were digitally scanned and the thickness of the aortic wall was measured in five different areas per aortic section using an ImageScope (Aperio Technologies Inc., Vista, CA, USA).
Measurement of biochemical parameters and lipid profiles
Serum levels of tumor necrosis factor-alpha (TNF-α; ABclonal, Wuhan, China), interleukin-1 beta (IL-1β; ABclonal), total cholesterol (TC; Mybiosource, San Diego, CA, USA), triglyceride (TG; Mybiosource) and low-density lipoprotein-cholesterol (LDL-C; Mybiosource) were measured using commercial kits with an automatic chemistry analyzer. The absorbance of sample was read at 450 nm.
Statistical analysis
Statistical analysis was performed using SPSS program (version 27), and all data were presented as a mean ± standard deviation (SD). Multiple comparisons of body weights, organ weights, FBG levels, biochemical parameters, lipid profile measurements and aortic thickness evaluations were analyzed by one-way or two-way analysis of variance (ANOVA) with post hoc Bonferroni-Dunn when necessary. A p-value of <0.05 or 0.005 for Bonferroni correction was considered statistically significant.
Results
shows the effect of E2 on body weight and FBG level of the study groups during the course of HT. Starting at the similar body weights, the final body weights of all groups at the end of experiment were not statistically different (body weights in mean ± SD grams of control, NT, T1, T2, and T3 as 20.3 ± 1.1, 22.4 ± 2.3, 20.8 ± 1.1, 22.0 ± 1.9, and 21.8 ± 1.3, respectively). FBG levels of T1, T2, and T3 were all significantly lower than NT at the end of the experiment, close to the control. Before starting HT (5th week), T1 showed a similar FBG level to that of NT (means ± SD of 144 ± 19 to 141 ± 16 mg/dL, respectively), but 3 weeks after HT, FBG level of T1 became as low as those of other groups than NT, which remained high at the end of the experiment.
Figure 2. Effect of estradiol (E2) treatment on physiologic status of POI mice during the course of HT.
A. Body weight change pattern. B. Fasting blood glucose (FBG) level change pattern.
FBG, fasting blood glucose; Cont, control; NT, no estradiol treatment; T1, treatment group 1 with delayed estradiol treatment for 3 weeks; T2, treatment group 2 with on-time estradiol treatment for 6 weeks; T3, treatment group 3 with on-time estradiol treatment for 3 weeks. #P-value <0.001 (vs. NT); ##P-value <0.01(vs. NT).
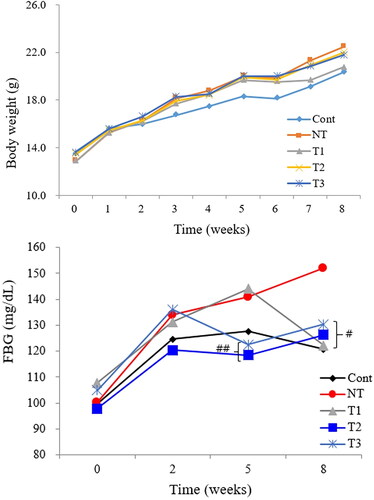
At the end of 8th week, the animals were sacrificed, and the final results are stated in and . describes the effect of E2 on various organ weights of the study groups. Weights of the liver, heart, spleen kidneys, and uterus were not statistically different among the groups. On the other hand, visceral fats were significantly accumulated in HFD groups compared to the control, and T1 and T2 showed significantly lower weights of visceral fats than that of NT, despite the similar body weights at the end of the experiment (p-value <.001). Ovary weights successfully increased in HT groups compared to the control and NT groups (p-value <.001).
Figure 3. Effect of estradiol (E2) streatment on metabolic parameters in POI mice with different initiation time points and durations of HT.
A. Serum total cholesterol levels. B. Serum triglyceride levels. C. Serum insulin levels. D. Serum low-density lipoprotein-cholesterol levels.
Cont, control; NT, no estradiol treatment; T1, treatment group 1 with delayed estradiol treatment for 3 weeks; T2, treatment group 2 with on-time estradiol treatment for 6 weeks; T3, treatment group 3 with on-time estradiol treatment for 3 weeks; LDL-C, low-density lipoprotein-cholesterol. *P-value <0.001 (vs. Cont); **P-value <0.01 (vs. Cont); #P-value <0.001 (vs. NT); ##P-value <0.005 (vs. NT); †P-value <0.005 (vs T2); ‡P-value <0.001(vs T2).
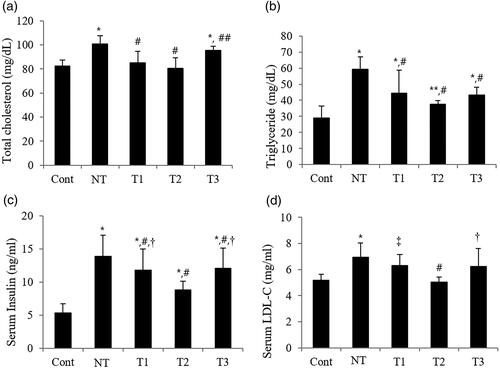
Figure 4. Effect of estradiol (E2) treatment on aortic thickness (μm) with different initiation time points and durations of HT.
A. Transverse section of descending thoracic aorta of the study subjects. B. Comparison of aorta thickness among the study groups.
Cont, control; NT, no estradiol treatment; T1, treatment group 1 with delayed estradiol treatment for 3 weeks; T2, treatment group 2 with on-time estradiol treatment for 6 weeks; T3, treatment group 3 with on-time estradiol treatment for 3 weeks. *P-value <0.001 (vs. Cont); #P-value <0.005 (vs. NT).
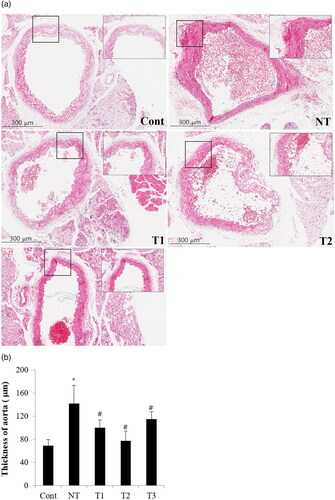
Figure 5. Effect of estradiol (E2) treatment on serum pro-inflammatory cytokines in POI mice with different initiation time points and durations of HT.
A. Serum TNF-α. B. Serum IL-1β.
TNF-α, tumor necrosis factor-α; IL-1β, Interleukin-1β;Cont, control; NT, no estradiol treatment; T1, treatment group 1 with delayed estradiol treatment for 3 weeks; T2, treatment group 2 with on-time estradiol treatment for 6 weeks; T3, treatment group 3 with on-time estradiol treatment for 3 weeks. *P-value <0.001 (vs. Cont); #P-value <0.001 (vs. NT); ##P-value <0.005 (vs. NT).
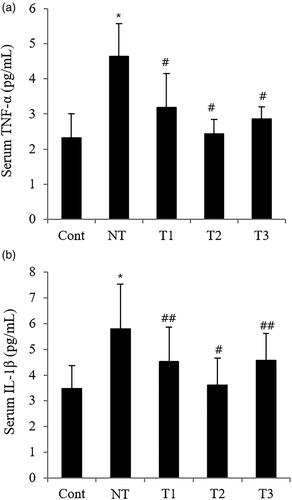
Table 2. Effect of estradiol (E2) treatment on organ weights in grams (g) of POI mice with different initiation time points and durations of HT.
In , the effect of E2 on metabolic parameters in the study groups with different initiation timing and duration of HT is evaluated. Serum TC was significantly lower in all HT groups than in NT, especially in T2 with the closest value to the control (p-value <.005); T1 and T2 showed similar levels to that of the control, but T3 had a significantly higher level of serum TC than the control (p-value <.001). Serum TG was also significantly lower in the HT groups than in NT, with the lowest value in T2 (p-value <.001). Similarly, serum insulin significantly decreased in the HT groups than in NT, with the statistically significant lowest value in T2 (p-value <.001). In the case of serum LDL, only T2 resulted a the statically lower level than those of NT, T1, and T3 (p-value <0.001).
displays the effect of E2 on possible cardiovascular risk markers in the study groups with different initiation time points and durations of HT. The thickness of the thoracic aorta was significantly increased with aggravated fibrotic change of the intima in mice with no E2 treatment, and such consequence was significantly ameliorated in HT groups, mostly lowered in T2 (p-value <0.005). Despite the lack of statistical significance, lipid deposits in the aortic lumen were the most vivid in NT, and among HT groups, T3 showed the densest lipid deposits in the aortic lumen along with the most fibrotic, thickened changes in aortic intima.
further analyzes the possibly underlying inflammatory pathway associated with the effect of E1 on the physiological changes in POI mice. Serum pro-inflammatory cytokines, both TNF-α and IL-1β, were significantly low in the HT groups than in NT, especially in T2 with the lowest level (p-value <.005).
Discussion
In POI patients, who require a longer duration of HT than the age-matched normal population undergoing natural menopause, the possible benefits of HT have been suggested and therefore cautiously recommended as disease management [Citation15]. Yet, specific HT regimen for POI has only been based on the limited number of longitudinal studies, and clinical data on possible health risks associated with the medication initiation timing and duration have not been published [Citation16]. Several retrospective cohort studies on the ‘older’ POI patients – patients with secondary amenorrhea and disease diagnosis after puberty – have been conducted to assess the relationship between HT and health risks, generating crude but rigid evidence for HT benefits on those patients, but for the ‘younger’ POI patients, including adolescents and children diagnosed before puberty, the evidence is more sparse [Citation17,Citation18]. These young POI patients are limited to be subjected to scientific research primarily due to rarity and ethical issues; secondarily, in a clinical setting, establishing the precise disease model of a ‘true’ estrogen-naïve state is almost impossible. Instead, animal studies with the POI mouse model could be a reasonable alternative and essential pilot candidate to further develop the understanding of the disease, especially when exploring physiologic changes and underlying mechanisms of specific medication with the lowest possible risks to the study subjects [Citation3,Citation19].
Several mouse models of ovarian insufficiency have been introduced, including surgical removal of ovaries (id est [i.e.], overiectomy) and medical intoxication of ovaries (i.e. systemic chemoagents) [Citation19]. Overiectomy has been popularly adopted in the previous studies on various types of menopause, but, due to its abrupt fall in circulating gonadal hormone levels after the surgical procedure, it is not so commonly used in the recent studies on transitional menopause with gradual decline in ovarian function [Citation19]. Mimicking gradual ovarian failure in humans, systemic cytotoxic agents such as chemoagents have been used, but it also has several limitations regarding unnecessary systemic damage on other organs than ovaries as well as the general physiology of the animal [Citation20]. To overcome such drawbacks, a novel mouse model of ovarian insufficiency using VCD was established in 2002; VCD explicitly targets primordial and primary follicles, with no evidence of an effect on large follicles after secondary follicle development, and therefore causes follicle depletion without affecting other tissues, and the resulted endocrine state well resembles that of ovary-intact menopausal transition [Citation21]. Since then, it has been widely spread around the globe for numerous studies exploring the effects of estrogen deprivation, its underlying mechanism and possible treatments on various diseases of neurodegenerative, cardiovascular, metabolic, endocrine and even psychosocial realms [Citation14,Citation22–26]. In the current study evaluating the ‘timing hypothesis’ of HT on physiologic parameters of POI mouse model, VCD-induced mouse model of ovarian failure was consequently chosen to best represent young POI women.
By definition, POI includes those patients with disease onset before puberty, thus considering young Turner syndrome patients, adolescents, and even younger patients with childhood cancer survivors, with a known prevalence of 1 in 10,000 under the age of 20 years. Female mice at 4 weeks of age were chosen in this study to exemplify such a younger population of POI women. The mice were treated with VCD as previously described, and a successful POI model was prepared, resulting the lower serum AMH and higher FSH, along with significantly decreased ovarian weights, in comparison to their age-matched control, suggesting significantly decreased ovarian function (data not shown) [Citation21]. These mice were exposed to E2 for 3 to 6 weeks at different initiation timing, in order to minimize the possible influence of aging and to maximize expected physiologic changes resulting on the subject by E2, as previously employed [Citation24,Citation27]. As expected, varying initiation timing and duration of E2 resulted in significant differences in FBG levels within similar body weights, accompanied by differences in visceral fat accumulation and metabolic parameters. Previous literature has confirmed the relationship between ovarian failure state and excessive fat accumulation along with deteriorated metabolic markers, and E2 treatment has been known for its corresponding compensating effect [Citation16,Citation28]. The treatment duration affected such compensating effect of E2 and consequent disease course; in this study of hormone-naïve model of POI mice, the group with the presence of estrogen deprivation benefited from the exposure to E2 per se, despite the delay, with better physiologic state than that of the never-treated group. The degree of restoration did fail to reach to that of the on-time, continuous treatment group, but the ameliorated difference was statically significant. As previously described, to maintain the protective effect of estrogen, its treatment was required to be steadily continuous without skipping intervals [Citation29].
Agreeing to the previous literature, estrogen deprivation caused by ovarian failure status in the current study led to the worsening of major metabolic and cardiovascular parameters. Such deteriorations were best ameliorated by immediate, continuous E2 treatment in pre-menarche female mice; when the treatment was delayed or stopped, its positive effect was less than the immediate intervention but still sufficient to result in more favorable outcomes than in the never-treated group. The immediate, continuous E2 treatment, even in the pre-menarche stage, could have possibly blocked the vicious cascade of systemic changes due to estrogen deprivation, in advance. The protective effect of E2 was maximized when E2 was initiated immediately after the ovarian failure event and used continuously; when the treatment stopped, the physiologic parameters of the animals were exacerbated to the level of those in the never-treated group with E2. In spite of a limited number of studies using only the reproductive-aged mouse model of ovarian insufficiency, several studies have already successfully evaluated the degree of E2 treatment with various initiation timing on not only cardiovascular parameters but also other metabolic markers and even neurodegenerative [Citation24,Citation29]. ‘Timing hypothesis’ of E2 treatment on atherosclerotic lesions, including actively growing and established-advanced ones, was studied by Ebenebe et al. [Citation24]; in their study, varying E2 initiation time points resulted in different degrees of protective effects, and possible explanations included the cellular mechanism of atherosclerosis formation and different types of estrogen receptors associated [Citation30]. Pollow et al. [Citation25] also reported the importance of immediate E2 initiation in the prevention of severe hypertension in their VCD-induced ovarian failure mouse model. Adding to the amplified benefit of E2 on cardiovascular risks, immediate E2 initiation further ensured improved brain function in mice [Citation26,Citation27]. The concept of ‘timing hypothesis’ in cardiovascular disease management, especially concerning atherosclerosis, may somewhat shed a light on explaining the interestingly similar pattern of benefits exerted by varying estrogen treatment initiations on other chronic diseases, building up the crucial knowledge to complete the puzzle for therapeutic guideline of such unresolved health issues.
In order to further examine the complex mechanism underlying varying E2 initiation time points and associated protective effects, the degree of oxidative stress and key pro-inflammatory cytokines were analyzed. López-Grueso et al. [Citation27] observed increased activities of antioxidant enzyme activities and decreased oxidative stress with E2 treatment on their VCD-induced mouse model of ovarian failure, and such benefits of E2 treatment varied among different initiation time points. In the current study, both serum TNF-α and IL-1β decreased in all E2-treated groups, and, repeatedly, the on-time, continuous E2 treatment group showed the lower level of those pro-inflammatory cytokines, suggesting that the probable state of oxidative stress caused by systemic estrogen deprivation was most amended by immediate E2 supplementation. Further investigations regarding the relationship between estrogen receptors and expression levels of pro-inflammatory protein markers are warranted, as such association is still on controversial [Citation31,Citation32].
The current study still has controversial limitations. As previously stated, VCD-induced mouse model of ovarian failure has been known for deteriorated metabolic parameters, such as elevated FBG level, insulin resistance, and especially, fatty liver [Citation29]. The current study had also expected an increased degree of adipocyte accumulation in the liver tissue of the subject mice, but histologic examination of the liver showed no significant difference in adipocyte accumulation among the study groups (data not shown). In this study, relatively young female mice – even before sexual maturation – were used, and their metabolic rate and recovery capability of possible hepatic damage probably outweighed the negative effect of HFD [Citation33]. Moreover, in order to deliberate the effect of underlying diseases on POI status per se, different murine strains for establishing POI animal models may be needed in future studies. Furthermore, extended duration of E2 treatment and related intervention should be considered, evaluating the long-term metabolic and cardiovascular risk later in the murine reproductive lifespan. Finally, future studies on the premenarche POI mouse model may be required to incorporate a comparative control group of older but not senescent female mice.
In conclusion, on-time, continuous treatment of E2 immediately after the biologic estrogen deprivation event significantly reduce metabolic and cardiovascular risks in a young, premenarche female mouse model of POI, confirming decreased serum level of pro-inflammatory cytokines, as summarized in . Based on the results of the current study, more studies on the complex mechanism of E2 has on various perspectives of metabolic and cardiovascular risks, according to various initiation time points and durations, are required in conducting further clinical and epidemiologic studies on child and adolescent POI patients, in order to advance the current therapeutic guideline for such important patients with misinterpreted rarity.
Supplemental Material
Download MS Word (15.5 KB)Acknowledgements
Authors of the study would like to express vast gratitude to all those who provided the authors with valuable opinions and suggestions for writing the paper.
Disclosure statement
No potential conflict of interest was reported by the authors.
Additional information
Funding
References
- Huhtaniemi I, Hovatta O, La Marca A, et al. Advances in the molecular pathophysiology, genetics, and treatment of primary ovarian insufficiency. Trends Endocrinol Metab. 2018;29(6):1–9. doi: 10.1016/j.tem.2018.03.010.
- Clark RA, Mostoufi-Moab S, Yasui Y, et al. Predicting acute ovarian failure in female survivors of childhood cancer: a cohort study in the childhood cancer survivor study (CCSS) and the St jude lifetime cohort (SJLIFE). Lancet Oncol. 2020;21(3):436–445. doi: 10.1016/S1470-2045(19)30818-6.
- Golezar S, Ramezani Tehrani F, Khazaei S, et al. The global prevalence of primary ovarian insufficiency and early menopause: a meta-analysis. Climacteric. 2019;22(4):403–411. doi: 10.1080/13697137.2019.1574738.
- Upton CE, Daniels JP, Davies MC. Premature ovarian insufficiency: the need for evidence on the effectiveness of hormonal therapy. Climacteric. 2021;24(5):453–458. doi: 10.1080/13697137.2021.1902496.
- ACOG. Committee Opinion No. 698: hormone therapy in primary ovarian insufficiency. Obstet Gynecol. 2017;129(5):e134–e41.
- Webber L, Davies M, Anderson R, et al. ESHRE guideline: management of women with premature ovarian insufficiency†. Hum Reprod. 2016;31(5):926–937.
- Baber RJ, Panay N, Fenton A, IMS Writing Group. 2016 IMS recommendations on women’s midlife health and menopause hormone therapy. Climacteric. 2016;19(2):109–150. doi: 10.3109/13697137.2015.1129166.
- NAMS. The 2017 hormone therapy position statement of the North American menopause society. Menopause. 2017;24(7):728–753.
- Lee SR, Cho MK, Cho YJ, et al. The 2020 menopausal hormone therapy guidelines. J Menopausal Med. 2020;26(2):69–98. doi: 10.6118/jmm.20000.
- Almeida M, Laurent MR, Dubois V, et al. Estrogens and androgens in skeletal physiology and pathophysiology. Physiol Rev. 2017;97(1):135–187. doi: 10.1152/physrev.00033.2015.
- Dubey RK, Imthurn B, Barton M, et al. Vascular consequences of menopause and hormone therapy: importance of timing of treatment and type of estrogen. Cardiovasc Res. 2005;66(2):295–306. doi: 10.1016/j.cardiores.2004.12.012.
- Graziottin A, Leiblum SR. Biological and psychosocial pathophysiology of female sexual dysfunction during the menopausal transition. J Sex Med. 2005;2 Suppl 3(s3):133–145. doi: 10.1111/j.1743-6109.2005.00129.x.
- Virdis A, Ghiadoni L, Pinto S, et al. Mechanisms responsible for endothelial dysfunction associated with acute estrogen deprivation in normotensive women. Circulation. 2000;101(19):2258–2263. doi: 10.1161/01.cir.101.19.2258.
- Lee HJ, Park MJ, Joo BS, et al. Effects of coenzyme Q10 on ovarian surface epithelium-derived ovarian stem cells and ovarian function in a 4-vinylcyclohexene diepoxide-induced murine model of ovarian failure. Reprod Biol Endocrinol. 2021;19(1):59. doi: 10.1186/s12958-021-00736-x.
- Sullivan SD, Sarrel PM, Nelson LM. Hormone replacement therapy in young women with primary ovarian insufficiency and early menopause. Fertil Steril. 2016;106(7):1588–1599. doi: 10.1016/j.fertnstert.2016.09.046.
- Webber L, Anderson RA, Davies M, et al. HRT for women with premature ovarian insufficiency: a comprehensive review. Hum Reprod Open. 2017;2017(2):1–11.
- Cleemann L, Holm K, Kobbernagel H, et al. Dosage of estradiol, bone and body composition in turner syndrome: a 5-year randomized controlled clinical trial. Eur J Endocrinol. 2017;176(2):233–242. doi: 10.1530/EJE-16-0582.
- Van Dorp W, Mulder RL, Kremer LCM, et al. Recommendations for premature ovarian insufficiency surveillance for female survivors of childhood, adolescent, and young adult cancer: a report from the international late effects of childhood cancer guideline harmonization group in collaboration with the PanCareSurFup consortium. J Clin Oncol. 2016;34(28):3440–3450. doi: 10.1200/JCO.2015.64.3288.
- Koebele SV, Bimonte-Nelson HA. Modeling menopause: the utility of rodents in translational behavioral endocrinology research. Maturitas. 2016;87:5–17. doi: 10.1016/j.maturitas.2016.01.015.
- Brinton RD. Minireview: translational animal models of human menopause: challenges and emerging opportunities. Endocrinology. 2012;153(8):3571–3578.
- Cao LB, Leung CK, Law PW-N, et al. Systemic changes in a mouse model of VCD-induced premature ovarian failure. Life Sci. 2020;262:118543. doi: 10.1016/j.lfs.2020.118543.
- Reis F, Pestana-Oliveira N, Leite CM, et al. Hormonal changes and increased anxiety-like behavior in a perimenopause-animal model induced by 4-vinylcyclohexene diepoxide (VCD) in female rats. Psychoneuroendocrinology. 2014;49:130–140. doi: 10.1016/j.psyneuen.2014.06.019.
- Christensen A, Pike CJ. Age-dependent regulation of obesity and alzheimer-related outcomes by hormone therapy in female 3xTg-AD mice. PLoS One. 2017;12(6):e0178490. doi: 10.1371/journal.pone.0178490.
- Ebenebe OV, Ashley Z, Erickson JR, et al. A timing effect of 17-β estradiol on atherosclerotic lesion development in female ApoE−/− mice. Int J Mol Sci. 2020;21(13):4710. doi: 10.3390/ijms21134710.
- Pollow DP, JrRomero-Aleshire MJ, Sanchez JN, et al. ANG II-induced hypertension in the VCD mouse model of menopause is prevented by estrogen replacement during perimenopause. Am J Physiol Regul Integr Comp Physiol. 2015;309(12):R1546–R1552. doi: 10.1152/ajpregu.00170.2015.
- Yin W, Maguire SM, Pham B, et al. Testing the critical window hypothesis of timing and duration of estradiol treatment on hypothalamic gene networks in reproductively mature and aging female rats. Endocrinology. 2015;156(8):2918–2933. doi: 10.1210/en.2015-1032.
- López-Grueso R, Gambini J, Abdelaziz KM, et al. Early, but not late onset estrogen replacement therapy prevents oxidative stress and metabolic alterations caused by ovariectomy. Antioxid Redox Signal. 2014;20(2):236–246. doi: 10.1089/ars.2012.5112.
- Noh H, Lee H, Kim S, et al. The efficacy of body mass index and total body fat percent in diagnosis obesity according to menopausal status. J Menopausal Med. 2019;25(1):55–62. doi: 10.6118/jmm.2019.25.1.55.
- Brooks HL, Pollow DP, Hoyer PB. The VCD mouse model of menopause and perimenopause for the study of sex differences in cardiovascular disease and the metabolic syndrome. Physiology (Bethesda). 2016;31(4):250–257. doi: 10.1152/physiol.00057.2014.
- Meng Q, Li Y, Ji T, et al. Estrogen prevent atherosclerosis by attenuating endothelial cell pyroptosis via activation of estrogen receptor α-mediated autophagy. J Adv Res. 2021;28:149–164. doi: 10.1016/j.jare.2020.08.010.
- Chadwick CC, Chippari S, Matelan E, et al. Identification of pathway-selective estrogen receptor ligands that inhibit NF-κB transcriptional activity. Proc Natl Acad Sci U S A. 2005;102(7):2543–2548. doi: 10.1073/pnas.0405841102.
- Straub RH. The complex role of estrogens in inflammation. Endocr Rev. 2007;28(5):521–574. doi: 10.1210/er.2007-0001.
- Nishikawa S, Yasoshima A, Doi K, et al. Involvement of sex, strain and age factors in high fat Diet-Induced obesity in C57BL/6J and BALB/cA mice. Exp Anim. 2007;56(4):263–272. doi: 10.1538/expanim.56.263.