Abstract
While current oral antiplatelet therapies benefit many patients, they deregulate the hemostatic balance leaving patients at risk of systemic side-effects such as hemorrhage. Dual antiplatelet treatment is the standard approach, combining aspirin with P2Y12 blockers. These therapies mainly target autocrine activation mechanisms (TxA2, ADP) and, more recently, the use of thrombin or thrombin receptor antagonists have been added to the available approaches. Recent efforts to develop new classes of anti-platelet drugs have begun to focus on primary platelet activation pathways such as through the immunoreceptor tyrosine-based activation motif (ITAM)-containing collagen receptor GPVI/FcRγ-chain complex. There are already encouraging results from targeting GPVI, with reduced aggregation and smaller arterial thrombi, without major bleeding complications, likely due to overlapping activation signaling pathways with other receptors such as the GPIb–V–IX complex. An alternative approach to reduce platelet activation could be to inhibit this signaling pathway by targeting the inhibitory pathways intrinsic to platelets. Stimulation of endogenous negative modulators could provide more specific inhibition of platelet function, but is this feasible? In this review, we explore the potential of the two major platelet immunoreceptor tyrosine-based inhibitory motif (ITIM)-containing inhibitory receptors, G6b-B and PECAM-1, as antithrombotic targets.
Historical Perspective
The immunoreceptor tyrosine-based inhibition motif (ITIM) comprises a conserved sequence of amino acids that were initially identified in the cytoplasmic tails of selected receptors on the surface of immune cells [Citation1]. The ITIM consensus sequence (L/I/V/S)xYxx(L/V) (single letter abbreviation where x can denote any amino acid) [Citation1,Citation2] is commonly found in pairs separated by 15 to 30 amino acid residues. ITIMs were named due to their role opposing the activity of immunoreceptor tyrosine-based activation motif (ITAM) bearing receptors in immune cell function [Citation3,Citation4]. The ITAM consensus sequence (YxxL/Ix6-12YxxL/I) is distinct from the ITIM most notably for being a dual tyrosine containing sequence [Citation5,Citation6]. However, in more recent years, a related motif, the hemITAM, has also been described (consensus sequence DEDGYxxL) [Citation7].
The first ITIM was identified in 1990s in the cytoplasmic tail of the immunoglobulin G (IgG) receptor in B cells FcγRIIB (CD32B) and shown to negatively regulate cell activation, proliferation, endocytosis, phagocytosis, and degranulation. Activation of FcγRIIB was reported to oppose the activation of the ITAM-bearing receptors FcγRIIA (CD32A) and the B-cell receptor (BCR) [Citation8–10]. Since then, ITIM-containing receptors have been identified in several cell types of the hematopoietic lineage such as mast cells, NK cells, T cells, macrophages, megakaryocytes, and platelets [Citation11].
Since ITIM receptors were discovered, some controversy has surrounded whether they possess inhibitory function alone, or whether they can also positively regulate pathways. This was recently reviewed by Coxon et al. [Citation12]. Briefly, the similar, but distinct immunoreceptor tyrosine-based switch motif (ITSM) has been described as an ITIM-like motif, with a consensus sequence was defined as TxYxxV/I [Citation13]. An ITSM can potentially confer activatory and/or inhibitory properties to a receptor in specific cells and scenarios [Citation13], depending on the associated signaling proteins. ITSMs were identified for the first time in the signaling lymphocyte adhesion molecule (SLAM) CD150. The small SH2-containing adaptor protein SH2 domain protein 1A (SH2D1A) binds to the CD150 ITSMs and regulates the association of receptors with SH2-containing molecules, and in this way serves as a signaling ‘switch’ for activation or inhibition [Citation13]. Notably, all identified ITIM-containing receptors on platelets bear an ITIM consensus sequence followed by an ITSM, which gives them the potential for both inhibitory and activatory roles.
ITIM-Containing Receptors in Megakaryocytes and Platelets
Three ITAM-containing receptors have been described in human megakaryocytes and platelets: GPVI/FcRγ-chain, FcγRIIA and CLEC-2 (hemITAM); and five ITIM-containing receptors (). Platelet Endothelial Cell Adhesion Molecule-1 (PECAM-1) or CD31 was thought to be the only ITIM-containing receptor in megakaryocytes and platelets [Citation14] until proteomics and transcriptomics studies revealed other structurally distinct ITIM-containing receptors: Carcino Embryonic Antigen-related Cell Adhesion Molecule 1 (CEACAM-1) and CEACAM-2, are both expressed at low levels on platelet surface; TREM-like transcript-1 (TLT-1), which is the most highly expressed and is stored in the α-granules [Citation15] and released upon platelet activation; Leukocyte-Associated Immunoglobulin-like Receptor-1 (LAIR-1), which is present on a variety of immune cells, while it is found on megakaryocytes, this protein has not been detected in platelets [Citation16]; and G6b-B. G6b-B is uniquely expressed in the platelet/megakaryocyte lineage [Citation17]. In this review we explore the potential of the ITIM-containing receptors as antithrombotic targets focusing on G6b-B and PECAM-1, since these have been more extensively characterized in this context [Citation12], but the principles and ideas discussed herein may apply to a number of other platelet ITIM receptors.
Figure 1. ITAM- and ITIM-bearing receptors on resting platelets. Immunoreceptor tyrosine-based activation motif (ITAM)-bearing platelet receptors are represented on the left together with the hemITAM receptor, CLEC-2. On the right, immune-receptor tyrosine-based inhibitory-motif (ITIM)-bearing platelet receptors are shown with their characteristic features: IgV-like domains, one in the case of G6b-B and six on PECAM-1; a transmembrane domain (TMD), a proline-rich region (PRR), an immune-receptor tyrosine-based inhibitory-motif (ITIM), and an immune-receptor immunoreceptor tyrosine-based switch-motif (ITSM). On PECAM-1 D1 and D2 are implicated for homophilic interaction, meanwhile, D6 for heterophilic binding
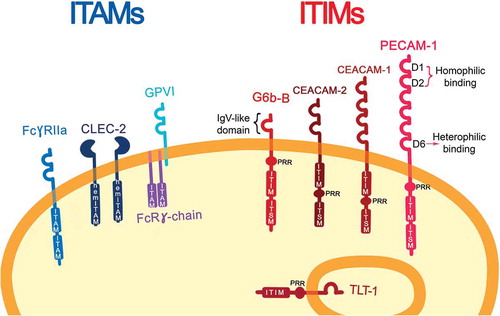
ITAM-ITIM Signaling
After ligand binding, the ITAM receptors GPVI/FcRγ-chain, and FcγRIIA, are tyrosine phosphorylated on the ITAM tyrosine residues, by Src family kinases (SFK, Fyn and Lyn) [Citation18]. GPVI has a conserved proline-rich region (PxxP) where SFKs bind and become activated [Citation18]. SFK phosphorylation takes place in lipid rafts, to where GPVI translocates upon ligand engagement [Citation19,Citation20]. This allows the recruitment and activation of the tyrosine kinase Syk which binds to the ITAM tyrosines via its tandem SH2 domains (). Src and Syk kinases are also required for activation of the hemITAM receptor CLEC-2. A hemITAM targets a similar downstream signaling pathway.
Figure 2. ITAM-ITIM activation. (A) ITAM-bearing receptor (GPVI/FcRγ-chain/CLEC-2/FcγRIIA) are activated by their respective ligands (collagen/podoplanin/antibodies). (B) Receptors are then translocated to lipid rafts where SFKs phosphorylate them. Syk is recruited, activated, and subsequently propagates the signal through the LAT signalosome, where p85/p110 are recruited to form PI3K. This results in PLCγ2 activation and further platelet activation. (C) On the bottom, ITIM-bearing receptor activation (PECAM-1/G6b-B) recruits SFK, phosphorylating the ITIM/ITSM motifs, providing docking sites for the phosphatases (SHP1/2 and SHIP1/2). This results in relocation of molecules, such as p85, away from lipid rafts and therefore a reduction in the activation of Syk and the LAT signalosome, leading to platelet inactivation/maintenance of the resting state
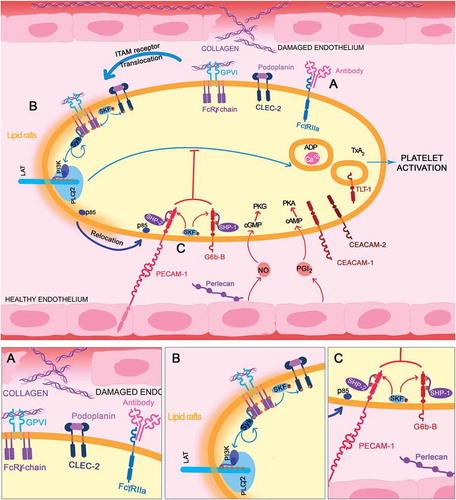
Once activated, Syk propagates the signal by phosphorylating the transmembrane scaffolding protein, linker for activation of T-cells (LAT). LAT has many tyrosine residues that allow the binding of different kinases, such as PI3K (p85/p110) via the SH2 domains of the p85 subunit and adaptor molecules, such as Grb-2-associated binding protein-1 (Gab1). Therefore, LAT phosphorylation allows the formation of a multi-protein complex that leads to the activation of phospholipase Cγ2 (PLCγ2) [Citation21] and, as a consequence, intracellular calcium increases leading to secretion and affinity modulation of the integrin αIIbβ3. This allows the binding of fibrinogen, platelet aggregation and thrombus formation. Secondary agonists, including ADP, TxA2 and serotonin, among others, are released from activated platelets and bind G protein-coupled receptors to activate surrounding resting platelets, synergizing to enhance the response.
SFKs, such as Fyn and Lyn, also phosphorylate tyrosine residues in ITIM/ITSMs and are therefore also able to mediate activation of the ITIM-receptor. This phosphorylation provides docking sites for SH2 domain(s) of phosphatases, such as the tyrosine phosphatases SHP1/SHP2 and the inositol phosphatase SHIP1/SHIP2 [Citation22]. These two phosphatase families lead to inactivation of various components of the ITAM stimulated pathway [Citation22]. The association of proteins with ITIM receptors can also sequester the proteins away from their action site, such as PECAM-1 that recruits p85, preventing its translocation into lipid rafts. This reduces the association of PI3K with Gab1 and LAT [Citation23] that are located in lipid rafts, leading into a reduction on PI3K signaling (). Contrastingly, ITIM-bearing receptors have been also implicated in the positive regulation of integrin outside-in signaling, which is dependent on associated proteins [Citation24–27] which we speculate may be consistent with the presence of an ITSM.
Although PECAM-1 and G6b-B are both ITIM-bearing receptors on platelets they are reported to differ in how and when they function. G6b-B is highly phosphorylated and associated with SHP1/SHP2 on resting platelets, while PECAM-1 phosphorylation is undetectable under resting conditions [Citation28]. This might suggest that G6b-B would be important to prevent unwanted activation, whereas PECAM-1 may be more important to restore resting conditions in response to platelet activation.
PECAM-1
PECAM-1 or CD31 is a 130-kDa transmembrane glycoprotein belonging to the immunoglobulin (Ig) gene superfamily [Citation29,Citation30] and was the first ITIM-containing receptor identified on platelets [Citation14]. PECAM-1 expression has been detected on the surface of both vascular endothelial cells, and a number of hematopoietic cells, including platelets, monocytes, neutrophils, T-cells, and B-cells [Citation31]. Proteomic studies have reported that PECAM-1 is expressed at ~9,400 copies per platelet in human [Citation32], and ~5,500 copies in mouse [Citation33], although other studies in human platelets suggest expression levels ranging from 5,000–20,000 copies per cell [Citation32–34] (). A key function of platelet PECAM-1 is to inhibit signaling downstream of the collagen receptor GPVI, and other platelet activation pathways, such as those mediated by ADP and thrombin [Citation35], thereby inhibiting platelet aggregation and thrombus formation in vitro [Citation36] and in vivo [Citation37].
Table I. Summary table comparing the main features of PECAM-1 and G6b-B
Structure
The Pecam1 gene is found on human chromosome 17. It is a single-copy gene of 65 kb composed of 16 exons [Citation38]. The gene is expressed as multiple isoforms in different cell types as a result of alternative splicing of encoding exons of the cytoplasmic domain; the most prevalent isoform in human platelets is the Δ15 isoform, which lacks exon 15 [Citation39]. The PECAM-1 extracellular domain (574 amino acids) is comprised of six Ig constant 2 (IgC2)-like domains (each encoded by a single exon) and is highly glycosylated with nine potential N-glycosylation sites [Citation29,Citation40]. The mature protein contains a short transmembrane domain of 19 amino acid residues and a 118 amino acid cytoplasmic domain encoded by eight different exons (exons 9–16) [Citation38] ().
The PECAM-1 cytoplasmic tail contains two tyrosine residues (conserved at positions 663, and 686 in human), that serve as sites for phosphorylation and the docking of cytosolic signaling molecules; a palmitoylation site (C595), and a proline-rich region (PRR) [Citation41]. Y663 and Y686 are located within the ITIM and ITSM, respectively. The PECAM-1 ITIM sequence is VQY663TEV and it is separated by 17 amino acid residues from the ITSM sequence, TVY686SEV [Citation42] (). The PECAM-1 cytoplasmic tail is associated to the lipid membrane by two separated regions [Citation43]. Serine 702 phosphorylation releases the ITSM motif from the membrane allowing its phosphorylation by Lyn and the sequential ITIM phosphorylation provides a docking site for SH2 domain phosphatases [Citation43]. Interestingly, Y663 is much less efficiently phosphorylated by either Src or Csk kinases than Y686 is [Citation44,Citation45] suggesting that its phosphorylation may be a rate-limiting step in PECAM-1–mediated signal transduction.
Ligands
Homophilic Binding
PECAM-1 has been shown to be activated upon homophilic binding forming large clusters [Citation46]. This interaction is involved in the maintenance of vascular endothelial cell barrier function [Citation47]. Through the use of domain deletions, and human/mouse domain swapping experiments, it has been shown that Ig-like domains 1 and 2 are responsible for this homophilic interaction () and consequent cell signaling, in addition to the level of surface expression [Citation46,Citation48–50]. Furthermore, homophilic binding interactions are dependent on the sialylation state of the PECAM‐1 ectodomain [Citation51]; this binding in human PECAM-1 may be promoted by α2,3-linked sialic acid residues whereas α2,6 sialic acids have been proposed to inhibit homophilic binding [Citation52].
Heterophilic Binding
PECAM-1 has also been described to bind other molecules (heterophilic binding) including glycosaminoglycans [Citation53], integrin αVβ3 [Citation46,Citation54,Citation55] and CD38 on lymphocytes [Citation56] but the functional relevance of these interactions is unknown. The only ligand for which a physiological role has been identified is the neutrophil-specific CD177/PR3 complex [Citation57], which binds Ig-like domain 6 () triggering neutrophil transmigration [Citation58].
G6b-B
G6b-B is a transmembrane protein expressed exclusively on megakaryocytes and platelets, with approximately ~14,000 copies per platelet in human [Citation32], and ~30,000 in mouse [Citation33], making it one of the most highly expressed platelet cell surface proteins (). G6b-B constitutively inhibits platelet activation by the ITAM-bearing receptors GPVI and CLEC-2 [Citation59].
Structure
In the human genome Mpig6b gene is located on chromosome 6 and composed of 6 exons which encode a 26 kDa protein comprised of 241 amino acids [Citation60]. G6b-B is also a member of the immunoglobulin superfamily and is expressed as several splice-variants [Citation60]. G6b-A and B contain transmembrane regions while the remaining three (C, D, E) are secreted isoforms [Citation60]. G6b-B is the only family member that contains a transmembrane region, along with a cytoplasmic region containing the ITIM and an ITSM, and therefore the only isoform capable of intracellular signaling. The G6b-B ITIM/ITSM is constitutively phosphorylated by SFKs on residues that act as docking sites for the SH2 domains of the cytoplasmic protein tyrosine phosphatases SHP-1 and SHP-2 [Citation17,Citation60,Citation61]. This leads to their activation, and the subsequent deactivation of tyrosine kinases such as Syk and of downstream signaling pathways.
G6b-B consists of a single variable-type Ig-like (IgV) domain which is N-glycosylated (1 site in humans and 2 in mice), a PRR (proline-rich region) in the juxtamembrane region, an ITIM and an ITSM [Citation17,Citation60,Citation62] (). The separation between the ITIM and ITSM on G6b-B is 26 amino acids [Citation60] and their sequences are LLY194ADL and TIY220AVV, respectively ().
Ligands
G6b-B has been described to bind the extracellular matrix heparan sulfate (HS), a subgroup of glycosaminoglycan defined by a basic disaccharide unit [Citation63] and heparin [Citation64]. Data from size-exclusion chromatography and X-ray crystallography suggest that ligand binding induces ectodomain dimerization [Citation63]. However, this dimerization is not enough to cluster G6b-B sufficiently into higher-order oligomers to induce robust downstream signaling [Citation63]. HS chains of vessel-wall perlecan may facilitate further G6b-B phosphorylation and downstream signaling via the tyrosine phosphatases SHP1 and SHP2, resulting in the inhibition of platelet activation [Citation63].
Potential as Therapeutic Targets
In order to be an ‘ideal therapeutic target’, a molecule should satisfy a number of properties: to be biologically relevant for the pathological process that requires modulation; specific to the target disease, biologically available and accessible, low sequence variability, minimum side effects (i.e. playing roles in multiple pathways), and toxicity; drugability, ability to bind a drug; and the potential to be combined with other therapies to obtain a synergistic effect [Citation65]. In this section we will explore which of these properties PECAM-1 and G6b-B meet ().
Table II. Summary table comparing PECAM-1 and G6b-B potential as therapeutic targets
PECAM-1
The antithrombotic potential of PECAM-1 is suggested by studies showing its ability to reduce platelet activation. Previous work has shown that PECAM-1 activation by antibody cross-linking has no effect on the early signaling events of GPVI activation, such as FcRγ-chain and Syk phosphorylation [Citation23]. However, approximately 80% of PECAM-1 is located outside of lipid rafts [Citation66] and this seems to be relevant after PECAM-1 activation, when SHP2 recruits p85, a regulatory subunit of PI3K, reducing the association of PI3K with Gab1 and LAT which are located within lipid rafts [Citation23]. This leads to a reduction of PI3K signaling and platelet activation decreases as a consequence. Additionally, PECAM-1 was shown to decrease platelet responses beyond ITAM signaling alone [Citation35,Citation67,Citation68]. Other cross-linking experiments showed that PECAM-1 cross-linking reduced GPIb-IX-V complex levels on the platelet surface leading to reduced binding of thrombin at the platelet surface and thereby lower levels of thrombin-stimulated platelet activation [Citation69].
The antithrombotic effects of PECAM-1 are relatively weak, particularly in comparison to PGI2 and NO [Citation70], and this inhibition can also be overcome at higher concentrations of the platelet agonists [Citation71,Citation72], which may be beneficial to reduce the risk of bleeding. One argument is that it could play a role in the context of atherosclerosis when the loss of healthy endothelium results in a decrease of PGI2 and NO concentrations [Citation70]. However, targeting PECAM-1 may reduce the risk of bleeding, which is the major risk factor with current antithrombotic therapies [Citation73,Citation74].
Importantly, the biological relevance of PECAM-1 in the regulation of platelet reactivity has been emphasized in human studies. PECAM-1 expression levels on the human platelet surface have been estimated to be ~9,400 copies per cell, in a proteomic study [Citation32], and between 5,000 and 8,800 copies per cell in other studies [Citation75]. However, these levels vary extensively up to 20,000 copies per cell in around 20% of the population, and high levels of expression are associated with a decrease in platelet response to CRP-XL, ADP and thrombin [Citation35]. Whether these latter individuals are less likely to suffer thrombosis remains to be studied. Additionally, further studies on allelic isoforms of PECAM-1 have established a relationship between some alleles and cardiovascular diseases (CVD). There are three main polymorphisms located in domains that are crucial for homophilic binding (Ig-like domain 1, L98V), heterophilic binding (Ig-like domain 6, S536N), and signal transduction (cytoplasmic domain, R643G) [Citation76]. These polymorphisms were identified in four major human PECAM-1 alleles (L98S536R643 and V98N536G643, associated with mutations within the 3′UTR, a2479 or g2479), which have been related with myocardial infarction and coronary artery disease, respectively [Citation76]. Deep vein thrombosis has also been associated with the L98V polymorphism of PECAM-1 [Citation77]. This relationship between some alleles and a link with CVD emphasizes the potential importance of PECAM-1 in regulating hemostasis and thrombosis.
In vivo studies using Pecam1 deficient transgenic mice have been used to explore the role of this protein in thrombopoiesis, hemostasis and thrombosis. Pecam1 knockout mice showed a delay in the kinetics of recovery of platelet number following antibody-induced thrombocytopenia, which was attributed to altered migration of megakaryocytes [Citation78]. Nevertheless, megakaryocyte maturation, proplatelet, and platelet formation are normal in Pecam1 deficient mice which have normal platelet counts [Citation78,Citation79]. Other in vivo studies on PECAM-1 knockout mice, have shown its contribution to thrombus size and stability. Thrombus size following laser injury to arterioles in PECAM-1-deficient mice formed more rapidly and were larger [Citation37]. Notably, the impact of overexpressing PECAM-1 on thrombosis and hemostasis has not been explored. As high levels of PECAM-1 on human platelets are associated with diminished platelet activation [Citation35], it would be particularly interesting to determine whether these latter individuals are less prone to thrombosis. These studies would support the notion that stimulation of PECAM-1 could be used as a therapy.
G6b-B
One of the advantages of G6b-B as a potentially effective and safe antithrombotic target relies on the fact that it is highly expressed on the cell surface and this is restricted to platelets and megakaryocytes. Potentially, this provides high specificity and low risk of off-target effects in other cell types. G6b-B, as with PECAM-1, is a cell-surface receptor; which increases the chances of successful drug delivery, as the drug does not have to cross the membrane to reach the target.
The majority of studies on G6b-B function have been based on transgenic knockout mice. It has been shown that megakaryocytes lacking G6b-B have reduced proplatelet formation leading to oversize platelets, a low platelet count and bleeding [Citation62]. In addition, G6b-B-deficient megakaryocytes display an increase of metalloproteinase production, responsible for cell-surface receptor shedding, such as GPVI [Citation62]. This appears to be a compensatory mechanism to downregulate the receptors regulated by G6b-B, since G6b-B constitutively inhibits platelet activation by ITAM-like receptors, GPVI and CLEC-2 (NB CLEC-2 lacks the cleavage site and therefore is not shed in G6b-B-deficient platelets) [Citation59]. Furthermore, G6b-B is constitutively phosphorylated under resting conditions [Citation17], indicating that it may play an important role preventing activation of circulating platelets. However, very few studies have explored the impact of G6b-B stimulation. Over 10 years ago, G6b-B cross-linking with polyclonal antibodies was shown to exert inhibition of both platelet activation and aggregation in vitro [Citation80]. This points to its potential as a target for antiplatelet therapy. Further studies in this direction using in vivo models and with monoclonal antibodies or other tools would clarify whether G6b-B stimulation could lead to less reactive platelets, reducing the risk, or severity of thrombosis.
Studies on humans have shown that G6b-B deletion and loss-of-function mutations lead to megakaryocytic and myelofibrotic disorders [Citation81], highlighting the importance of this receptor not only for platelet regulation but also for megakaryocyte function. Further studies should be performed to investigate whether, as with PECAM-1, there are differences in expression between individuals. If there is a link between higher expression of G6b-B and a lower rate of thrombotic events, this would support the notion that stimulation of G6b-B could be used therapeutically.
How to Target PECAM-1 and G6b-B?
We can conclude therefore, that both, PECAM-1 and G6b-B are biologically relevant for hemostasis, thrombosis, and platelet responsiveness, and therefore worthy of consideration as targets for new antiplatelet therapy. A pertinent question then, would be how could we achieve PECAM-1 and G6b-B activation therapeutically/pharmacologically?
The classical way to address a molecular target would be to develop a small molecule suitable for oral therapy. Small molecules, however, tend to be less successful when targeting protein-protein interactions such as the homophilic binding of PECAM-1 [Citation82], but there are notable exceptions such as Tirofiban, and Maraviroc. Tirofiban binds to the platelet integrin αIIbβ3 and inhibits platelet aggregation, while Maraviroc inhibits interaction between human CCR5 and HIV-1 gp120 [Citation83].
Another approach would be to use a monoclonal antibody that activates PECAM-1 or G6b-B to prevent platelet aggregation. Specifically, a Fab or Fab2 fragment of a humanized antibody would most likely be required to avoid impaired interactions with the immune system. This approach has been applied successfully with Abciximab, the first anti-integrin αIIbβ3 antigen-binding fragment approved to inhibit platelet aggregation in cardiovascular disease [Citation84]. The numbers of antibodies approved as therapeutic agents rise every year, with their success likely due to their high specificity, affinity and stability [Citation85]. Nevertheless, a key disadvantage of antibody therapy is that they are not suitable for oral therapy. Nanobodies could be an alternative that may be suitable for oral therapy due to their proteolytic resistance thereby retaining their activity as they pass through the gastrointestinal tract. An example of this is V565, an anti-TNFα oral nanobody currently in phase II of clinical trials [Citation86]. However, the first nanobody approved by the FDA in 2019, Cablivi™ is an intravenous therapy [Citation87], although this may reflect the nature of the target disorder, thrombotic thrombocytopenic purpura, and that it is used in combination with plasma exchange and immunosuppressive therapy [Citation87]. A single nanobody may not cause clustering, however it would be possible to fuse them, for example, to generate a bispecific nanobody, binding both PECAM-1 and G6b-B. The incorporation of the G6b-B nanobody would allow selective activation of PECAM-1 on platelets over other cell types expressing PECAM-1.
A newer approach to target PECAM-1 or G6b-B would be affimers; non-antibody binding proteins derived from a scaffold engineered from human stefin A and a cystatin consensus sequence [Citation88]. While affimers are in the early stages of development, promising results have been accomplished stabilizing fibrin networks with potential reduction on bleeding risk, with a fibrinogen-binding affimer [Citation89]. Activatory affimers targeting G6b-B/PECAM-1 would be promising therapy, although this has yet to be attempted.
Further research is needed to explore these ideas, and fully determine the potential success of targeting these receptors to prevent thrombotic disease without causing substantial bleeding.
Concluding Remarks
In summary, new antiplatelet agents and targets that overcome current oral antiplatelet therapies side-effects are needed and are actively sought by pharmaceutical companies. One approach would be to target primary platelet activation pathways such as the activation provided by the ITAM-containing receptors GPVI and CLEC-2. Here we have outlined how conversely targeting ITIM-containing receptors may provide an alternative approach for targeted platelet inhibition due to the role of these receptors in the downregulation of platelet ITAM-receptor signaling. There are already encouraging results targeting the GPVI pathway; the humanized Fc fusion protein of the GPVI ectodomain, commercially known as Revacept [Citation90], and the human GPVI-blocking F(ab) ACT017 [Citation91,Citation92] both currently in phase II of clinical trials.
Targeting ITIM-containing receptors may provide a new selective approach to downregulate ITAM dependent platelet activation, and potentially activation mediated through other pathways. However, further studies are needed to shed light on the outcome of activating PECAM-1 and/or G6b-B and their potential as targets for antiplatelet therapy. Whether it would downregulate platelet activation, whether it may overcome current antiplatelet therapies preventing bleeding and whether this can be achieved therapeutically to prevent cardiovascular diseases are important questions for the future.
Declaration Of Interest
The authors report no conflict of interest.
Acknowledgements
This work was supported by the European Union’s Horizon 2020 research and innovation programme under the Marie Skłodowska-Curie grant agreement No 766118. The authors would like to thank Chukiat Tantiwong for useful discussions.
Additional information
Funding
References
- Vivier E, Daeron M. Immunoreceptor tyrosine-based inhibition motifs. Immunol Today 1997;186:p.286–91. DOI:https://doi.org/10.1016/S0167-5699(97)80025-4
- Burshtyn DN, Yang W, Yi T, Long EO. A novel phosphotyrosine motif with a critical amino acid at position −2 for the SH2 domain-mediated activation of the tyrosine phosphatase SHP-1. J Biol Chem 1997;27220:p.13066–13072. DOI:https://doi.org/10.1074/jbc.272.20.13066
- Daeron M. Intracytoplasmic sequences involved in the biological properties of low-affinity receptors for IgG expressed by murine macrophages. Braz J Med Biol Res 1995;283:p. 263–74.
- D’Ambrosio D, Hippen K, Minskoff S, Mellman I, Pani G, Siminovitch K, Cambier J. Recruitment and activation of PTP1C in negative regulation of antigen receptor signaling by Fc gamma RIIB1. Science 1995;2685208:p.293–297. DOI:https://doi.org/10.1126/science.7716523
- Reth M. Antigen receptor tail clue. Nature 1989;3386214:p.383–384. DOI:https://doi.org/10.1038/338383b0
- Cambier JC. New nomenclature for the Reth motif (or ARH1/TAM/ARAM/YXXL). Immunol Today 1995;162:p.110. DOI:https://doi.org/10.1016/0167-5699(95)80105-7
- Hughes CE, Sinha U, Pandey A, Eble JA, O’Callaghan CA, Watson SP. Critical Role for an acidic amino acid region in platelet signaling by the HemITAM (hemi-immunoreceptor tyrosine-based activation motif) containing receptor CLEC-2 (C-type lectin receptor-2). J Biol Chem 2013;2887:p. 5127–35. DOI:https://doi.org/10.1074/jbc.M112.411462
- Amigorena S, Bonnerot C, Drake JR, Choquet D, Hunziker W, Guillet JG, Webster P, Sautes C, Mellman I, Fridman WH. Cytoplasmic domain heterogeneity and functions of IgG Fc receptors in B lymphocytes. Science 1992;2565065:p. 1808–12. DOI:https://doi.org/10.1126/science.1535455
- Muta T, Kurosaki T, Misulovin Z, Sanchez M, Nussenzweig MC, Ravetch JV. A 13-amino-acid motif in the cytoplasmic domain of Fc gamma RIIB modulates B-cell receptor signalling. Nature 1994;3696478:p. 340. https://doi.org/10.1038/369340a0
- Daeron M, Latour S, Malbec O, Espinosa E, Pina P, Pasmans S, Fridman WH. The same tyrosine-based inhibition motif, in the intracytoplasmic domain of Fc gamma RIIB, regulates negatively BCR-, TCR-, and FcR-dependent cell activation. Immunity 1995;35:p. 635–46. https://doi.org/10.1016/1074-7613(95)90134-5
- Newman PJ. Switched at birth: a new family for PECAM-1. J Clin Invest 1999;1031:p. 5–9. DOI:https://doi.org/10.1172/JCI5928
- Coxon CH, Geer MJ, Senis YA. ITIM receptors: more than just inhibitors of platelet activation. Blood 2017;12926:p. 3407–3418. DOI:https://doi.org/10.1182/blood-2016-12-720185
- Sidorenko SP, Clark EA. The dual-function CD150 receptor subfamily: the viral attraction. Nat Immunol 2003;41:p. 19–24. https://doi.org/10.1038/ni0103-19
- Gibbins JM. The negative regulation of platelet function: extending the role of the ITIM. Trends Cardiovasc Med 2002;125:p. 213–9. https://doi.org/10.1016/S1050-1738(02)00164-0
- Washington AV, Schubert RL, Quigley L, Disipio T, Feltz R, Cho EH, McVicar DW. A TREM family member, TLT-1, is found exclusively in the alpha-granules of megakaryocytes and platelets. Blood 2004;1044:p.1042–7. https://doi.org/10.1182/blood-2004-01-0315
- Steevels TA, Westerlaken GHA, Tijssen MR, Coffer PJ, Lenting PJ, Akkerman JWN, Meyaard L. Co-expression of the collagen receptors leukocyte-associated immunoglobulin-like receptor-1 and glycoprotein VI on a subset of megakaryoblasts. Haematologica 2010;9512:p. 2005–12. https://doi.org/10.3324/haematol.2010.026120
- Senis YA, Tomlinson MG, García Á, Dumon S, Heath VL, Herbert J, Cobbold SP, Spalton JC, Ayman S, Antrobus R, et al. A comprehensive proteomics and genomics analysis reveals novel transmembrane proteins in human platelets and mouse megakaryocytes including G6b-B, a novel immunoreceptor tyrosine-based inhibitory motif protein. Mol Cell Proteomics 2007;63:p. 548–64. https://doi.org/10.1074/mcp.D600007-MCP200
- Suzuki-Inoue K, Tulasne D, Shen Y, Bori-Sanz T, Inoue O, Jung SM, Moroi M, Andrews RK, Berndt MC, Watson SP, et al. Association of Fyn and Lyn with the proline-rich domain of glycoprotein VI regulates intracellular signaling. J Biol Chem 2002;27724:p. 21561–6. https://doi.org/10.1074/jbc.M201012200
- Locke D, Chen H, Liu Y, Liu C, Kahn ML. Lipid rafts orchestrate signaling by the platelet receptor glycoprotein VI. J Biol Chem 2002:27721:p. 18801–9. https://doi.org/10.1074/jbc.M111520200
- Wonerow P, OBERGFELL A, WILDE JI, BOBE R, ASAZUMA N, BRDIČKA T, LEO A, SCHRAVEN B, HOŘEJŠÍ V, SHATTIL SJ, et al. Differential role of glycolipid-enriched membrane domains in glycoprotein VI- and integrin-mediated phospholipase Cgamma2 regulation in platelets. Biochem J 2002:3643:p. 755–65. https://doi.org/10.1042/bj20020128
- Pasquet JM, Gross B, Quek L, Asazuma N, Zhang W, Sommers CL, Schweighoffer E, Tybulewicz V, Judd B, Lee JR, et al. LAT is required for tyrosine phosphorylation of phospholipase cgamma2 and platelet activation by the collagen receptor GPVI. Mol Cell Biol 1999;1912:p. 8326–34. https://doi.org/10.1128/MCB.19.12.8326
- Billadeau DD, Leibson PJ. ITAMs versus ITIMs: striking a balance during cell regulation. J Clin Invest 2002;1092:p. 161–8. https://doi.org/10.1172/JCI0214843
- Moraes LA, Barrett NE, Jones CI, Holbrook LM, Spyridon M, Sage T, Newman DK, Gibbins JM.. Platelet endothelial cell adhesion molecule-1 regulates collagen-stimulated platelet function by modulating the association of phosphatidylinositol 3-kinase with Grb-2-associated binding protein-1 and linker for activation of T cells. J Thromb Haemost 2010;811:p. 2530–41. https://doi.org/10.1111/j.1538-7836.2010.04025.x
- Wee JL, Jackson DE. The Ig-ITIM superfamily member PECAM-1 regulates the “outside-in” signaling properties of integrin alpha(IIb)beta3 in platelets. Blood 2005;10612:p. 3816–23. https://doi.org/10.1182/blood-2005-03-0911
- Mazharian A, Mori J, Wang Y-J, Heising S, Neel BG, Watson SP, Senis YA. Megakaryocyte-specific deletion of the protein-tyrosine phosphatases Shp1 and Shp2 causes abnormal megakaryocyte development, platelet production, and function. Blood 2013;12120:p. 4205–20. https://doi.org/10.1182/blood-2012-08-449272
- Yip J, Alshahrani M, Beauchemin N, Jackson DE. CEACAM1 regulates integrin alphaIIbbeta3-mediated functions in platelets. Platelets 2016;272:p. 168–77. https://doi.org/10.3109/09537104.2015.1064102
- Alshahrani MM, Kyriacou RP, O’Malley CJ, Heinrich G, Najjar SM, Jackson DE. CEACAM2 positively regulates integrin alphaIIbbeta3-mediated platelet functions. Platelets 2016;278:p. 743–750. https://doi.org/10.3109/09537104.2016.1171834
- Stefanini L, Bergmeier W. Negative regulators of platelet activation and adhesion. J Thromb Haemost 2018;162:p. 220–230. https://doi.org/10.1111/jth.13910
- Newman PJ, Berndt M, Gorski J, White G, Lyman S, Paddock C, Muller W. PECAM-1 (CD31) cloning and relation to adhesion molecules of the immunoglobulin gene superfamily. Science 1990;2474947:p. 1219–22. https://doi.org/10.1126/science.1690453
- Stockinger H, Gadd SJ, Eher R, Majdic O, Schreiber W, Kasinrerk W, Strass B, Schnabl E, Knapp W. Molecular characterization and functional analysis of the leukocyte surface protein CD31. J Immunol 1990;14511:p. 3889–97.
- Albelda SM, Oliver PD, Romer LH, Buck CA. EndoCAM: a novel endothelial cell-cell adhesion molecule. J Cell Biol 1990;110(4):p. 1227–37. https://doi.org/10.1083/jcb.110.4.1227
- Burkhart JM, Vaudel M, Gambaryan S, Radau S, Walter U, Martens L, Geiger J, Sickmann A, Zahedi RP. The first comprehensive and quantitative analysis of human platelet protein composition allows the comparative analysis of structural and functional pathways. Blood 2012;120(15):p. e73–82. https://doi.org/10.1182/blood-2012-04-416594
- Zeiler M, Moser M, Mann M. Copy number analysis of the murine platelet proteome spanning the complete abundance range. Mol Cell Proteomics 2014;1312:p. 3435–45. https://doi.org/10.1074/mcp.M114.038513
- Novinska M, Rathore V, Newman DK, Newman PJ. Chapter 11—Pecam-1 Platelets. 2007. Academic Press,Pages 221-230,ISBN 9780123693679, https://doi.org/10.1016/B978-012369367-9/50773-4.
- Jones CI, Garner SF, Moraes LA, Kaiser WJ, Rankin A, Ouwehand WH, Goodall AH, Gibbins JM. PECAM-1 expression and activity negatively regulate multiple platelet signaling pathways. FEBS Lett 2009;58322:p. 3618–24. https://doi.org/10.1016/j.febslet.2009.10.037
- Patil S, Newman DK, Newman PJ. Platelet endothelial cell adhesion molecule-1 serves as an inhibitory receptor that modulates platelet responses to collagen. Blood 2001;976:p. 1727–32. https://doi.org/10.1182/blood.V97.6.1727
- Falati S, Patil S, Gross PL, Stapleton M, Merrill-Skoloff G, Barrett NE, Pixton KL, Weiler H, Cooley B, Newman DK, et al. Platelet PECAM-1 inhibits thrombus formation in vivo. Blood 2006;1072:p. 535–41. https://doi.org/10.1182/blood-2005-04-1512
- Kirschbaum NE, Gumina RJ, Newman PJ. Organization of the gene for human platelet/endothelial cell adhesion molecule-1 shows alternatively spliced isoforms and a functionally complex cytoplasmic domain. Blood 1994;8412:p. 4028–37. https://doi.org/10.1182/blood.V84.12.4028.bloodjournal84124028
- Bergom C, Paddock C, Gao C, Holyst T, Newman DK, Newman PJ. An alternatively spliced isoform of PECAM-1 is expressed at high levels in human and murine tissues, and suggests a novel role for the C-terminus of PECAM-1 in cytoprotective signaling. J Cell Sci 2008;1218:p. 1235–42. https://doi.org/10.1242/jcs.025163
- Newton JP, Hunter AP, Simmons DL, Buckley CD, Harvey DJ. CD31 (PECAM-1) exists as a dimer and is heavily N-glycosylated. Biochem Biophys Res Commun 1999;2612:283–291. https://doi.org/10.1006/bbrc.1999.1018
- Newman PJ, Newman DK. Signal transduction pathways mediated by PECAM-1: new roles for an old molecule in platelet and vascular cell biology. Arterioscler Thromb Vasc Biol 2003;236:p. 953–64. https://doi.org/10.1161/01.ATV.0000071347.69358.D9
- Jackson DE, Kupcho KR, Newman PJ. Characterization of phosphotyrosine binding motifs in the cytoplasmic domain of platelet/endothelial cell adhesion molecule-1 (PECAM-1) that are required for the cellular association and activation of the protein-tyrosine phosphatase, SHP-2. J Biol Chem 1997;27240:p. 24868–75. https://doi.org/10.1074/jbc.272.40.24868
- Paddock C, Lytle BL, Peterson FC, Holyst T, Newman PJ, Volkman BF, Newman DK. Residues within a lipid-associated segment of the PECAM-1 cytoplasmic domain are susceptible to inducible, sequential phosphorylation. Blood 2011;11722:p. 6012–23. https://doi.org/10.1182/blood-2010-11-317867
- Osawa M, Masuda M, Harada N, Lopes RB, Fujiwara K. Tyrosine phosphorylation of platelet endothelial cell adhesion molecule-1 (PECAM-1, CD31) in mechanically stimulated vascular endothelial cells. Eur J Cell Biol 1997;723:p. 229–37.
- Cao MY, Huber M, Beauchemin N, Famiglietti J, Albelda SM, Veillette A. Regulation of mouse PECAM-1 tyrosine phosphorylation by the Src and Csk families of protein-tyrosine kinases. J Biol Chem 1998;27325:p. 15765–72. https://doi.org/10.1074/jbc.273.25.15765
- Sun QH, DeLisser HM, Zukowski MM, Paddock C, Albelda SM, Newman PJ. Individually distinct Ig homology domains in PECAM-1 regulate homophilic binding and modulate receptor affinity. J Biol Chem 1996;27119:p. 11090–8. https://doi.org/10.1074/jbc.271.19.11090
- Privratsky JR, Paddock CM, Florey O, Newman DK, Muller WA, Newman PJ. Relative contribution of PECAM-1 adhesion and signaling to the maintenance of vascular integrity. J Cell Sci 2011;124Pt 9:p. 1477–85. https://doi.org/10.1242/jcs.082271
- Sun J, Williams J, Yan H-C, Amin KM, Albelda SM, DeLisser HM. Platelet endothelial cell adhesion molecule-1 (PECAM-1) homophilic adhesion is mediated by immunoglobulin-like domains 1 and 2 and depends on the cytoplasmic domain and the level of surface expression. J Biol Chem 1996;27131:p. 18561–70. https://doi.org/10.1074/jbc.271.31.18561
- Newton JP, Buckley CD, Jones EY, Simmons DL. Residues on both faces of the first immunoglobulin fold contribute to homophilic binding sites of PECAM-1/CD31. J Biol Chem 1997;27233:p. 20555–63. https://doi.org/10.1074/jbc.272.33.20555
- Paddock C, Zhou D, Lertkiatmongkol P, Newman PJ, Zhu J. Structural basis for PECAM-1 homophilic binding. Blood 2016;1278:p. 1052–61. https://doi.org/10.1182/blood-2015-07-660092
- Lee C, Liu A, Miranda-Ribera A, Hyun SW, Lillehoj EP, Cross AS, Passaniti A, Grimm PR, Kim B-Y, Welling PA, et al. NEU1 sialidase regulates the sialylation state of CD31 and disrupts CD31-driven capillary-like tube formation in human lung microvascular endothelia. J Biol Chem 2014;28913:9121–9135. https://doi.org/10.1074/jbc.M114.555888
- Lertkiatmongkol P, Paddock C, Newman DK, Zhu J, Thomas MJ, Newman PJ. The role of sialylated glycans in human platelet endothelial cell adhesion molecule 1 (PECAM-1)-mediated trans homophilic interactions and endothelial cell barrier function. J Biol Chem 2016;29150:p. 26216–26225. https://doi.org/10.1074/jbc.M116.756502
- DeLisser HM, Yan HC, Newman PJ, Muller WA, Buck CA, Albelda SM. Platelet/endothelial cell adhesion molecule-1 (CD31)-mediated cellular aggregation involves cell surface glycosaminoglycans. J Biol Chem 1993;26821:p. 16037–46. https://doi.org/10.1016/S0021-9258(18)82354-7
- Buckley CD, Doyonnas R, Newton JP, Blystone SD, Brown EJ, Watt SM, Simmons DL. Identification of alpha v beta 3 as a heterotypic ligand for CD31/PECAM-1. J Cell Sci 1996;109Pt 2:p. 437–45.
- Piali L, Hammel P, Uherek C, Bachmann F, Gisler RH, Dunon D, Imhof BA. CD31/PECAM-1 is a ligand for alpha v beta 3 integrin involved in adhesion of leukocytes to endothelium. J Cell Biol 1995;130(2):p. 451–60. https://doi.org/10.1083/jcb.130.2.451
- Deaglio S, Morra M, Mallone R, Ausiello CM, Prager E, Garbarino G, Dianzani U, Stockinger H, Malavasi F. Human CD38 (ADP-ribosyl cyclase) is a counter-receptor of CD31, an Ig superfamily member. J Immunol 1998;160(1):p. 395–402.
- Kuckleburg CJ, Newman PJ. Neutrophil proteinase 3 acts on protease-activated receptor-2 to enhance vascular endothelial cell barrier function. Arterioscler Thromb Vasc Biol 2013;332:p. 275–84. https://doi.org/10.1161/ATVBAHA.112.300474
- Sachs UJ, Andrei-Selmer CL, Maniar A, Weiss T, Paddock C, Orlova VV, Choi EY, Newman PJ, Preer KT, Chavakis T, et al. The neutrophil-specific antigen CD177 is a counter-receptor for platelet endothelial cell adhesion molecule-1 (CD31). J Biol Chem 2007;28232:p. 23603–12. https://doi.org/10.1074/jbc.M701120200
- Mori J, Pearce AC, Spalton JC, Grygielska B, Eble JA, Tomlinson MG, Senis YA, Watson SP. G6b-B inhibits constitutive and agonist-induced signaling by glycoprotein VI and CLEC-2. J Biol Chem 2008;28351:p. 35419–27. https://doi.org/10.1074/jbc.M806895200
- de Vet EC, Aguado B, Campbell RD. G6b, a novel immunoglobulin superfamily member encoded in the human major histocompatibility complex, interacts with SHP-1 and SHP-2. J Biol Chem 2001;27645:p. 42070–6. https://doi.org/10.1074/jbc.M103214200
- Senis YA, Antrobus R, Severin S, Parguiña AF, Rosa I, Zitzmann N, Watson SP, García A. Proteomic analysis of integrin alphaIIbbeta3 outside-in signaling reveals Src-kinase-independent phosphorylation of Dok-1 and Dok-3 leading to SHIP-1 interactions. J Thromb Haemost 2009;710:p. 1718–26. https://doi.org/10.1111/j.1538-7836.2009.03565.x
- Mazharian A, Wang Y-J, Mori J, Bem D, Finney B, Heising S, Gissen P, White JG, Berndt MC, Gardiner EE, et al. Mice lacking the ITIM-containing receptor G6b-B exhibit macrothrombocytopenia and aberrant platelet function. Sci Signal 2012;5248:ra78. https://doi.org/10.1126/scisignal.2002936
- Vogtle T, Sharma S, Mori J, Nagy Z, Semeniak D, Scandola C, Geer MJ, Smith CW, Lane J, Pollack S, et al. Heparan sulfates are critical regulators of the inhibitory megakaryocyte-platelet receptor G6b-B. Elife 2019;8. https://doi.org/10.7554/eLife.46840
- de Vet EC, Newland SAB, Lyons PA, Aguado B, Campbell RD. The cell surface receptor G6b, a member of the immunoglobulin superfamily, binds heparin. FEBS Lett 2005;57911:p. 2355–8. https://doi.org/10.1016/j.febslet.2005.03.032
- Gashaw I, Ellinghaus P, Sommer A, Asadullah K. What makes a good drug target? Drug Discov Today 2011;1623–24:p. 1037–43. https://doi.org/10.1016/j.drudis.2011.09.007
- Sardjono CT, Harbour S, Yip J, Paddock C, Tridandapani S, Newman P, Jackson D. Palmitoylation at Cys595 is essential for PECAM-1 localisation into membrane microdomains and for efficient PECAM-1-mediated cytoprotection. Thromb Haemost 2006;966:p. 756–66. https://doi.org/10.1160/TH06-08-0459
- Rathore V, Stapleton MA, Hillery CA, Montgomery RR, Nichols TC, Merricks EP, Newman DK, Newman PJ. PECAM-1 negatively regulates GPIb/V/IX signaling in murine platelets. Blood 2003;10210:p. 3658–64. https://doi.org/10.1182/blood-2003-06-1888
- Crockett J, Newman DK, Newman PJ. PECAM-1 functions as a negative regulator of laminin-induced platelet activation. J Thromb Haemost 2010;87:p. 1584–93. https://doi.org/10.1111/j.1538-7836.2010.03883.x
- Jones CI, Sage T, Moraes LA, Vaiyapuri S, Hussain U, Tucker KL, Barrett NE, Gibbins JM. Platelet endothelial cell adhesion molecule-1 inhibits platelet response to thrombin and von Willebrand factor by regulating the internalization of glycoprotein Ib via AKT/glycogen synthase kinase-3/dynamin and integrin alphaIIbbeta3. Arterioscler Thromb Vasc Biol 2014;349:p. 1968–76. https://doi.org/10.1161/ATVBAHA.114.304097
- Dhanjal TS, Ross EA, Auger JM, Mccarty OJT, Hughes CE, Senis YA,Buckley CD, Watson SP. Minimal regulation of platelet activity by PECAM-1. Platelets 2007;181:p. 56–67. https://doi.org/10.1080/09537100600881396
- Jones KL, Hughan SC, Dopheide SM, Farndale RW, Jackson SP, Jackson DE. Platelet endothelial cell adhesion molecule-1 is a negative regulator of platelet-collagen interactions. Blood 2001;985:p. 1456–63. https://doi.org/10.1182/blood.V98.5.1456
- Cicmil M, Thomas JM, Leduc M, Bon C, Gibbins JM. Platelet endothelial cell adhesion molecule-1 signaling inhibits the activation of human platelets. Blood 2002;991:137–144. https://doi.org/10.1182/blood.V99.1.137
- Gurbel PA,Myat A, Kubica J, Tantry US. State of the art: oral antiplatelet therapy. JRSM Cardiovasc Dis 2016;5:2048004016652514.
- Yeung J, Holinstat M. Newer agents in antiplatelet therapy: a review. J Blood Med 2012;3:33–42. https://doi.org/10.2147/JBM.S25421
- Jones CI, Barrett NE, Moraes LA, Gibbins JM, Jackson DE. Endogenous inhibitory mechanisms and the regulation of platelet function. Methods Mol Biol 2012;788:341–366.
- Novinska MS, Pietz BC, Ellis TM, Newman DK, Newman PJ. The alleles of PECAM-1. Gene 2006;3761:95–101. https://doi.org/10.1016/j.gene.2006.02.016
- Li G, Han ZL, Dong HG, Zhang X, Kong XQ, Jin X. Platelet endothelial cell adhesion molecule-1 gene 125C/G polymorphism is associated with deep vein thrombosis. Mol Med Rep 2015;122:2203–2210. https://doi.org/10.3892/mmr.2015.3586
- Dhanjal TS, Pendaries C, Ross EA, Larson MK, Protty MB, Buckley CD, Watson SP. A novel role for PECAM-1 in megakaryocytokinesis and recovery of platelet counts in thrombocytopenic mice. Blood 2007;10910:4237–4244. https://doi.org/10.1182/blood-2006-10-050740
- Mahooti S, Graesser D, Patil S, Newman P, Duncan G, Mak T, Madri JA. PECAM-1 (CD31) expression modulates bleeding time in vivo. Am J Pathol 2000;1571:75–81. https://doi.org/10.1016/S0002-9440(10)64519-1
- Newland SA, Macaulay IC, Floto AR, de Vet EC, Ouwehand WH, Watkins NA, Lyons PA, Campbell DR. The novel inhibitory receptor G6B is expressed on the surface of platelets and attenuates platelet function in vitro. Blood 2007;10911:4806–4809. https://doi.org/10.1182/blood-2006-09-047449
- Hofmann I, Geer MJ, Vögtle T, Crispin A, Campagna DR, Barr A, Calicchio ML, Heising S, van Geffen JP, Kuijpers MJE, et al. Congenital macrothrombocytopenia with focal myelofibrosis due to mutations in human G6b-B is rescued in humanized mice. Blood 2018;13213:1399–1412. https://doi.org/10.1182/blood-2017-08-802769
- Gurevich EV, Gurevich VV. Therapeutic potential of small molecules and engineered proteins. Handb Exp Pharmacol 2014;219:1–12.
- Buchwald P. Small-molecule protein-protein interaction inhibitors: therapeutic potential in light of molecular size, chemical space, and ligand binding efficiency considerations. IUBMB Life 2010;6210:724–731. https://doi.org/10.1002/iub.383
- Faulds D, Sorkin EM. Abciximab (c7E3 Fab). A review of its pharmacology and therapeutic potential in ischaemic heart disease. Drugs 1994;484:583–598. https://doi.org/10.2165/00003495-199448040-00007
- Chames P, Van Regenmortel M, Weiss E, Baty D. Therapeutic antibodies: successes, limitations and hopes for the future. Br J Pharmacol 2009;1572:220–233. https://doi.org/10.1111/j.1476-5381.2009.00190.x
- Nurbhai S, Roberts KJ, Carlton TM, Maggiore L, Cubitt MF, Ray KP, Reckless J, Mohammed H, Irving P, MacDonald TT, et al. Oral anti-tumour necrosis factor domain antibody V565 provides high intestinal concentrations, and reduces markers of inflammation in ulcerative colitis patients. Sci Rep 2019;91:14042. https://doi.org/10.1038/s41598-019-50545-x
- Duggan S. Caplacizumab: first global approval. Drugs 2018;7815:1639–1642. https://doi.org/10.1007/s40265-018-0989-0
- Tiede C, Bedford R, Heseltine SJ, Smith G, Wijetunga I, Ross R, AlQallaf D, Roberts AP, Balls A, Curd A, et al. Affimer proteins are versatile and renewable affinity reagents. Elife 2017;6. https://doi.org/10.7554/eLife.24903
- Kearney KJ, Pechlivani N, King R, Tiede C, Phoenix F, Cheah R, Macrae FL, Simmons KJ, Manfield IW, Smith KA, et al. Affimer proteins as a tool to modulate fibrinolysis, stabilize the blood clot, and reduce bleeding complications. Blood 2019;13311:1233–1244. https://doi.org/10.1182/blood-2018-06-856195
- Ungerer M, Rosport K, Bültmann A, Piechatzek R, Uhland K, Schlieper P, Gawaz M, Münch G. Novel antiplatelet drug revacept (Dimeric Glycoprotein VI-Fc) specifically and efficiently inhibited collagen-induced platelet aggregation without affecting general hemostasis in humans. Circulation 2011;12317:1891–1899. https://doi.org/10.1161/CIRCULATIONAHA.110.980623
- Lebozec K, Jandrot-Perrus M, Avenard G, Favre-Bulle O, Billiald P. Design, development and characterization of ACT017, a humanized Fab that blocks platelet’s glycoprotein VI function without causing bleeding risks. MAbs 2017;96:945–958. https://doi.org/10.1080/19420862.2017.1336592
- Voors-Pette C, Lebozec K, Dogterom P, Jullien L, Billiald P, Ferlan P, Renaud L, Favre-Bulle O, Avenard G, Machacek M, et al. Safety and tolerability, pharmacokinetics, and pharmacodynamics of ACT017, an antiplatelet GPVI (glycoprotein VI) Fab. Arterioscler Thromb Vasc Biol 2019;395:956–964. https://doi.org/10.1161/ATVBAHA.118.312314