Abstract
Platelets are central to thrombosis. Research at the intersection of biological and physical sciences provides proof-of-concept for shear rate-dependent platelet slip at vascular stenosis and near device surfaces. Platelet slip extends the observed biological “slip-bonds” to the boundary of functional gliding without contact. As a result, there is diminished engagement of the coagulation cascade by platelets at these surfaces. Comprehending platelet slip would more precisely direct antithrombotic regimens for different shear environments, including for percutaneous coronary intervention (PCI). In this brief report we promote translation of the proof-of-concept for platelet slip into improved antithrombotic regimens by: (1) reviewing new supporting basic biological science and clinical research for platelet slip; (2) hypothesizing the principal variables that affect platelet slip; (3) applying the consequent construct model in support of-and in some cases to challenge-relevant contemporary guidelines and their foundations (including for urgent, higher-risk PCI); and (4) suggesting future research pathways (both basic and clinical). Should future research demonstrate, explain and control platelet slip, then a paradigm shift for choosing and recommending antithrombotic regimens based on predicted shear rate should follow. Improved clinical outcomes with decreased complications accompanying this paradigm shift for higher-risk PCI would also result in substantive cost savings.
The contemporary evolution of prescribed antithrombotic regimens is driven by the evolution of our understanding of the mechanism of thrombosis, with subsequent influence by associated clinical research.Citation1 Our understanding of the mechanism of thrombosis is based primarily on basic biological science, which increasingly intersects with the physical sciences.Citation2 Effective antithrombotic regimens are then established through randomized controlled trials. These regimens form the principal foundation for practice guidelines. However, practices change when new information becomes available that downgrades or dispatches a regimen (e.g., bivalirudinCitation3 and low molecular weight dextran,Citation4 respectively, for percutaneous coronary intervention [PCI]).
Platelets are central to thrombosis.Citation5 High shear rates activate the multimeric protein von Willebrand factor (vWF) through a conformation change in its A1 domain.Citation6 That conformational change promotes binding to the platelet glycoprotein (GP)Ibα component of the GPIb-IX-V receptor, resulting in platelet adhesion (with subsequent activation, secretion, and aggregation). This high-shear-dependent conformational change and accompanying adhesion is an example of the transition of the A1 domain from a “slip-bond” to a “catch-bond.”Citation7,Citation8 However, further increases in shear rate appear to reverse this transition from catch-bond back to slip-bond.Citation9
Biological slip in the bond context differs from fluid dynamic slip. In fluid dynamics, slip at a fluid-solid interface indicates that the fluid has a non-zero velocity at the surface of the solid ().Citation5 Nevertheless, fluid dynamics provides the opportunity to model biological slip out to the boundary of functional gliding. In parallel, a perfect biological catch-bond at optimal shear would correlate with fluid dynamic no-slip, and a perfect slip-bond would correlate with perfect slip.
Figure 1. Velocity profile of fluid flow through a cylinder.Citation5 Lower left panel: no-slip boundary condition. Middle left panel: partial slip. Upper left panel: perfect slip. True slip (upper right panel) indicates the slip layer at the boundary is of molecular dimension, and effective (or apparent) slip (lower right panel) indicates the local velocity varies over a finite, albeit small, mesoscopic distance. Fluid flow is in the z-direction, steady, laminar and incompressible. R = cylinder inside radius; uR = fluid velocity at cylinder inside surface (“slip velocity”); umax = maximum flow velocity.
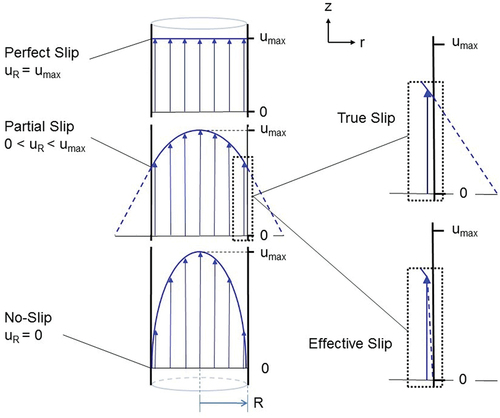
A recently published research article in Platelets described a validated model of platelet slip at vascular stenosis and near PCI device surfaces ().Citation5 In this model the measured slip of microspheres-albeit potentially effective-provides proof-of-concept for platelet slip, and shows that their slip is shear-dependent.
Figure 2. Representative illustrations from the validated model of platelet slip at vascular stenosis and near PCI device surfaces.Citation5 Platelets were modeled using 3.12 μm diameter polystyrene (non-adhesive) microspheres. The model test section was cylindrical, flow was continuous, and the view presented is longitudinal of the horizontal middle plane. The velocities of the microspheres were determined by laser-Doppler velocimetry (blue rings and discs: ○;●). These velocities were compared with COMSOL Multiphysics computer-simulations of plasma velocity profiles (black solid lines). The model had the capacity to insert a central solid cylinder to model a PCI guide wire (a “cylinder-within-a-cylinder”). The was 20–90% by cross sectional area. The Reynolds number of 300 is within the range of coronary flow.Citation25 All velocities were normalized to the mean velocity u0, calculated from direct volumetric measurement of bulk fluid flow. Computer simulations applied the no-slip boundary condition at each surface, noting wall shear stresses in the unobstructed coronary circulation promote minimal plasma slip.Citation56 The blue discs (●) highlight partial or effective slip (i.e.: non-zero velocity) of microspheres immediately adjacent to the surfaces of the stenoses and central cylinder surface. PCI = percutaneous coronary intervention.
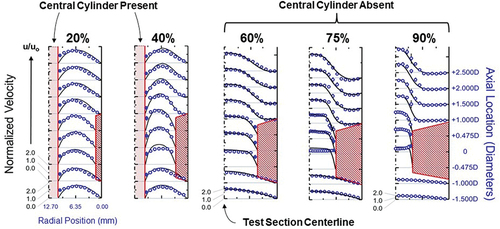
Although this model was very fundamental, it nonetheless extends the slip-bond concept to the boundary of shear-induced functional gliding of platelets along a surface. This model reconciles theslip-/catch-bond concept with classic theory for early experimental observations of increasing-then-decreasing platelet adhesion with monotonically increasing shear.Citation9 Moreover, diminished platelet adhesion results in diminished engagement of the coagulation cascade by platelets at both vascular stenosis and device surfaces. Thus, the perceived necessity of anticoagulation is demoted. Correspondingly, early observational studies indicate disposal of anticoagulation altogether indeed decreases bleeding events without an increase in ischemic events.Citation10,Citation11
In this research,Citation5 for a mean velocity of blood flow down a normal proximal left anterior descending coronary artery at 30 cm×sec−1, the critical threshold fluid shear rate to promote slip was calculated to be 318.3 sec−1. However, this threshold shear rate does not account for confounding biological phenomena.
Here we present new biological science and clinical research that support the concept and presence of platelet slip. We also hypothesize the principal variables that affect platelet slip and apply the consequent construct model to relevant guidelines, including those for urgent, higher-risk PCI. Finally, we suggest future research pathways.
(1) Although unintentional, the new supporting basic biological science is compelling. Arce, et al., reported that the discontinuous autoinhibitory module flanking the A1 domain of vWF resists tensile force (shear), but cooperatively unfolds above a certain threshold to expose A1.Citation12 They also reported that, at very high shear rates (10000 sec−1) and compared with whole blood, the antagonist VHH81 partially suppresses and ARC1172 achieves near-complete suppression of vWF-mediated platelet adhesion.
Arce, et al., confirmed for whole blood the anticipated “catch-bond” increase in platelet adhesion with an increase in shear rate from 1000–2500 sec−1 ( left panelCitation12). However, they then document a significant-and disproportionateCitation13-decrease in platelet adhesion with the further increase in shear rate from 2500–10 000 sec−1. Importantly, these shear rates are above the range for shear-induced platelet detachment.Citation14 Additionally, their parallel-plate flow chamber did not include any ADAMTS13-type protease. Applying our model, the decrease in platelet adhesion indicates a transition from catch-bond to slip-bond toward the boundary of gliding at a critical threshold plasma shear rate between 2500–10000 sec−1. Conveniently, this shear rate range spans the rate identified in early experimental observations (2600 sec−1)Citation9. The addition of VHH81 and ARC1172 caused, respectively, shear-induced amplification of and physiologically near perfect platelet slip compared with whole blood alone, indicating the slip-promoting properties of these antagonists.
Figure 3. Comparison of perfused whole blood platelet adhesion to collagen (% Area covered) at shear rates 1000 –10 000 sec−1 with no inhibitors, VHH81 (14 μg/mL) or ARC1172 (1 μM) mixed with recalcified blood before perfusion.Citation12 Comparison between platelet coverage [adhesion] was analyzed with a two-way ANOVA with mixed effects and Tukey’s multiple comparison correction. p values after multiple comparison corrections are displayed for relevant groups. Data are means±standard deviation with n = number of fields analyzed per condition. Reproduced with permission.
![Figure 3. Comparison of perfused whole blood platelet adhesion to collagen (% Area covered) at shear rates 1000 –10 000 sec−1 with no inhibitors, VHH81 (14 μg/mL) or ARC1172 (1 μM) mixed with recalcified blood before perfusion.Citation12 Comparison between platelet coverage [adhesion] was analyzed with a two-way ANOVA with mixed effects and Tukey’s multiple comparison correction. p values after multiple comparison corrections are displayed for relevant groups. Data are means±standard deviation with n = number of fields analyzed per condition. Reproduced with permission.](/cms/asset/40bbb79a-0738-4a69-948e-9c50e1bf25cc/iplt_a_2353582_f0003_oc.jpg)
The new clinical research supporting platelet slip is two-fold: First, a retrospective study of antiplatelet therapy only, without anticoagulation, for urgent, higher-risk PCI;Citation15 and second, a randomized trial comparing antiplatelet therapies without anticoagulation for the treatment of acute ischemic stroke (AIS).Citation16
The PCI studyCitation15 consisted of 481 consecutive patients receiving an antithrombotic regimen of antiplatelet therapy only (oral aspirin, P2Y12 inhibitor; intravenous GPIIb-IIIa inhibitor), without anticoagulation. This antithrombotic regimen diverges from PCI guidelines which have consistently supported a combination of antiplatelet and anticoagulant therapies.Citation17 Most patients (88.4%) presented with non-ST elevation acute coronary syndrome (NSTE-ACS). Additional high-risk features were prevalent.Citation18 For example, a majority (68.0%) had active New York Heart Association class III-IV congestive heart failure (CHFIII-IV). Also, nearly one-half (48.2%) had diabetes mellitus (DM; insulin dependent-23.3%). All procedures were performed via femoral artery access.
The PCI procedural success rate was high (99.2%). Additionally, there was a low rate of 72-hour and 30-day major adverse cardiovascular events (MACE; combined all-cause death/myocardial infarction/urgent target vessel revascularization: 2.6% [death-0%] and 4.4% [death-0.8%], respectively). Finally, adverse bleeding event rates were low (Bleeding Academic Research Consortium [BARC] 5/deathCitation19: 0% and 0.2%, respectively; BARC 3: 1.2% and 2.1%, respectively).
Moreover, the subgroup of biomarker positive NSTE-ACS patients (N = 228) similarly demonstrated a significant proportion of high-risk clinical features including active CHFIII-IV in 70.6% and DM in 43.4% (insulin dependent-19.7%).Citation20 As anticipated, their 72-hour and 30-day MACE rates were somewhat higher: 4.3% [death-0%] and 7.5% [death-1.8%/cardiac-0.5%], respectively. However, bleeding events remained low (BARC 5/death: 0% and 0.4%, respectively; BARC 3: 1.8% and 2.6%, respectively). Also, urgent target vessel revascularization was low (0% and 1.3%, respectively). These results compare favorably with contemporary-but-lower-risk biomarker positive NSTE-ACS PCI patients receiving combined antiplatelet and anticoagulation therapies (at 30 days: MACE 9.6% [death-1.7%/cardiac-1.1%]; “serious bleeding” [comparable to the summation of BARC 3+5]: 4.3%).Citation21
Shear-dependent platelet slip can account for these positive results, acknowledging the influence of concurrent antiplatelet therapy ( upper panel). The antiplatelet therapy was mandatory in the peri-stenosis high-to-extremely high-shear environment (4,000–40,000 sec−1)Citation22 to limit adhesion, activation and aggregation of platelets by activated plasma vWF. Additionally, the GPIIb-IIIa inhibitor was particularly useful for patients poorly responsive to aspirin and/or P2Y12 inhibitorCitation23 and to disperse preexisting platelet aggregates.Citation24 Also, the GP inhibitor served as a safety net in eliminating any platelet adhesion to surface vWF and other proteins (e.g., adsorbed Factor-1) for the intermediate-to-high shear rate ranges spanning normal coronary arterial flow (200–800 sec−1 Citation22,Citation25). The Achilles’ Heel to the concurrent antiplatelet therapy is that any ADAMTS13 cleaving of unfolded vWF would similarly promote bleeding events. This acquired von Willebrand syndrome would potentially resolve following PCI-induced restoration of normal shear rates.Citation26
Figure 4. Effect of shear rate on platelets at a PCI device surface (upper panel)Citation5 and at a site of endothelial disruption (lower panel). Left-low shear; Right-high shear.
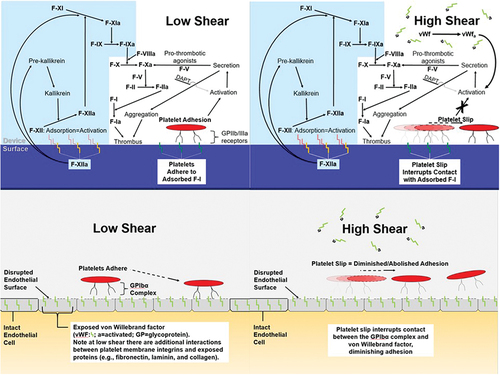
The trialCitation16 randomized 1177 patients with AIS without occlusion of large or medium-sized vessels to either the intravenous GPIIb-IIIa inhibitor tirofiban or oral low-dose aspirin (but no anticoagulation). The principal finding was that tirofiban resulted in a greater likelihood of a positive outcome compared with aspirin at 90 days (modified Rankin score 0 or 1: tirofiban-29.1% versus aspirin-22.2%; p = .02). Moreover, incidences of intracranial hemorrhage were very low (but higher with tirofiban: 1.0% versus 0%, respectively; p = .03).
AIS in the absence of large or medium-sized vessel occlusion generally involves the deep penetrating arteries.Citation16 In this high-shear environment,Citation22 despite the disrupted endothelium and exposed sub-endothelial proteins that characterizes atherothrombosis,Citation27 platelet slip explains the lack of engagement of the coagulation cascade ( lower panel).
(2) The results of the above new supporting research contribute to hypothesizing three principal variables that affect platelet slip. These three variables are physical and biological:
(1) Local shear rate.
(2) Quantitative area/volume/density, composition and catch-/slip-bond propensity of proteins within plasma, on device and exposed sub-endothelial surfaces.
(3) Adhesiveness of platelets (both intrinsic and drug-induced [e.g., downstream effect of P2Y12 inhibitors on platelet P-selectin, decreasing adhesivenessCitation28]).
These three variables provide a construct model that is simply a more explicit version of Virchow’s triad.Citation29 This triad consists of stasis, vessel damage and hypercoagulability in describing the etiology and risk of deep vein thrombosis (DVT).
(3) This construct model of platelet slip generally supports current guidelines regarding antithrombotic regimens, which are based most securely on randomized trials (and occasionally meta-analyses). For example, the management of DVT and most atrial fibrillation (low-shear) focus on anticoagulation.Citation30,Citation31 Conversely, management of AIS (high-shear) focuses on antiplatelet therapies.Citation32
NSTE-ACS and PCI both involve high-shear environments.Citation22 Nonetheless, both sets of those guidelines advocate a combination of antiplatelet and anticoagulation therapies.Citation33,Citation34 Notably, retrospective studies of pre-PCI NSTE-ACS patients indicate that, in the presence of oral dual antiplatelet therapy (aspirin and P2Y12 inhibitor), a reduced doseCitation11 or even zero anticoagulationCitation35 result in no significant increase in ischemic but a decrease in bleeding events.
Thus, despite >45 years of research,Citation36 the optimal antithrombotic regimen for NSTE-ACS PCI patients remains uncertain. The above report for urgent, higher-risk PCICitation15 supports antiplatelet therapy only, without anticoagulation. In the PCI guidelines,Citation34 the level of recommendation for anticoagulation during any PCI is Class I (benefit>>>risk; procedure/treatment should be performed/administered). However, the level of evidence is C (very limited populations evaluated; only consensus opinion of experts, case studies or standards of care). Rather unexpectedly, in those guidelines only a cursory reference is made to the sole randomized trial evaluating antiplatelet therapy only, without anticoagulation, for PCI (qualifying for level of evidence B).
That trial (CIAOCitation37) showed a significant decrease in both ischemic and bleeding events for elective PCI patients receiving oral aspirin and P2Y12 inhibitor (clopidogrel) without versus with unfractionated heparin (p = .034 and 0.048, respectively). In addition to highlighting the efficacy of antiplatelet therapy, these results underscore untoward consequences of heparin. Those consequences include: heparin-based anticoagulants can paradoxically activate platelets and induce other prothrombotic activities;Citation38 increase bleeding in a dose-dependent fashion;Citation39 and cause thrombocytopenia.Citation40 These limitations of the heparin family and the results of CIAO contribute to the continued use of unfractionated heparin in PCI as “the use of an FDA-approved drug for a non-FDA-approved indication.”Citation41
(4) Future research pathways to advance the proof-of-concept for platelet slip should include basic and clinical studies:
(1) Both a contemporary validation of the original model of coronary flow and expanded flow cytometry studies; and
(2) Additional quantitative clinical research.
Unfortunately, to date, there remains no technology that can directly visualize or measure platelet slip in a biological environment. A contemporary model of coronary flow would take advantage of advanced engineering technologies using a true-scale model with physiologic pulsation and platelet-like particles.Citation42 Additionally, microsphere imaging could employ newer particle image velocimetry techniques.Citation43
The flow cytometry studies would build upon prior relevant studiesCitation9,Citation12,Citation23,Citation28 with a focus on determining the critical threshold shear rate for different geometries, pulsatile flow patterns and therapeutic antagonists. Additionally, these studies could clarify mechanisms accounting for prior observations of reduced fibrin formation in higher shear environments near device surfaces.Citation44 These additional studies would therefore contribute to the knowledge base for slip-bonds confined to coagulation factors.Citation45
The quantitative clinical research would logically involve a sequential approach to extend the inference of in-vivo platelet slip and its clinical impact on thrombosis for urgent, higher-risk PCI: (1) a propensity score analysis comparing the urgent PCI patients using antiplatelet therapy onlyCitation15 to a matched set of PCI patients who also received anticoagulation; then (2) a registry; and then, finally, (3) a randomized controlled trial. Each successive step would naturally be predicated upon a positive previous step. Also, the registry and randomized trial would include an independent variable of femoral versus radial artery access to facilitate resolving the accompanying controversy.Citation46
A positive outcome to the future research may promote parallel research into larger-diameter arterial sheath procedures with known concerning adverse bleeding event rates. For example, the bleeding event rate for transcatheter aortic valve replacement is 31.9%.Citation47,Citation48 Additionally-similar to PCICitation19,Citation49-there is a significant increase in subsequent short- and long-term adverse events including death. Also, a positive outcome may facilitate improving therapies for other cardiovascular structures, devices and conditions (e.g., left atrium after appendage occlusionCitation50,Citation51; left ventricular assists devicesCitation52,Citation53).
Moreover, a positive outcome and the resulting attenuation of PCI complications would decrease 30-day MACE for biomarker positive NSTE-ACS patientsCitation21 by at least 9.6–7.5 = 2.1% (net ≥21.9% decrease). Also, BARC 3+5 bleeding would decrease by at least 4.3–3.0 = 1.3% (net ≥30.2% decrease). We estimate the consequent cost-savings for similar PCI patients in the United States during their index hospitalization alone at ≥$200-500 M/year.Citation15,Citation54,Citation55 The cost-savings and novel expanded knowledge of thrombosis should support both the basic and quantitative clinical research despite not involving a new drug or device. Additionally, artificial intelligence may improve and compliment this research while providing financial relief without sacrificing authenticity.Citation1 The positive trials should facilitate translating the proof-of-concept of platelet slip into improved antithrombotic regimens.
Thus, a paradigm shift for choosing and recommending antithrombotic regimens based upon the intersection of basic biological and physical sciences (and directly affecting PCI) may be on the horizon.
Disclosure statement
No potential conflict of interest was reported by the author(s).
Additional information
Funding
References
- Subbiah V. The next generation of evidence-based medicine. Nat Med. 2023;29(1):49–7. doi:10.1038/s41591-022-02160-z.
- NRC:National Academies of Sciences, Engineering, and Medicine. Research at the Intersection of the Physical and Life Sciences. Washington (DC): The National Academies Press; 2010. doi:10.17226/12809.
- Ibanez B, James S, Agewall S, Antunes MJ, Bucciarelli-Ducci C, Bueno H, Caforio ALP, Crea F, Goudevenos JA, Halvorsen S. et al. 2017 ESC guidelines for the management of acute myocardial infarction in patients presenting with ST-segment elevation: the task force for the management of acute myocardial infarction in patients presenting with ST-segment elevation of the European Society of Cardiology (ESC). Eur Heart J. 2018;39(2):119–77. doi:10.1093/eurheartj/ehx393.
- Gruberg L, Dangas G, Leon MB. Coronary artery stents: appropriate use of adjunctive pharmacotherapy to prevent stent thrombosis. Drugs Aging. 1999;15(5):341–8. doi:10.2165/00002512-199915050-00002.
- Denardo SJ, Denardo BC, Carpinone PL, Dean WT, New DM, Estrada LE, Green CL, Yock PG, Karunasiri G. Validated model of platelet slip at stenosis and device surfaces. Platelets. 2020;31(3):373–82. doi:10.1080/09537104.2019.1636021.
- Auton M, Zhu C, Cruz MA. The mechanism of VWF-mediated platelet GPIbalpha binding. Biophys J. 2010;99(4):1192–201. doi:10.1016/j.bpj.2010.06.002.
- Dembo M, Torney DC, Saxman K, Hammer D. The reaction-limited kinetics of membrane-to-surface adhesion and detachment. Proc R Soc Lond B Biol Sci. 1988;234(1274):55–83. doi:10.1098/rspb.1988.0038.
- Marshall BT, Long M, Piper JW, Yago T, McEver RP, Zhu C. Direct observation of catch bonds involving cell-adhesion molecules. Nature. 2003;423(6936):190–3. doi:10.1038/nature01605.
- Turitto VT, Baumgartner HR. Platelet interaction with subendothelium in flowing rabbit blood: effect of blood shear rate. Microvasc Res. 1979;17(1):38–54. doi:10.1016/0026-2862(79)90006-2.
- Denardo SJ, Davis KE, Tcheng JE. Elective percutaneous coronary intervention using broad-spectrum antiplatelet therapy (eptifibatide, clopidogrel, and aspirin) alone, without scheduled unfractionated heparin or other antithrombin therapy. Am Heart J. 2005;149(1):138–44. doi:10.1016/j.ahj.2004.06.002.
- Denardo SJ, Davis KE, Tcheng JE. Effectiveness and safety of reduced-dose enoxaparin in non-ST-segment elevation acute coronary syndrome followed by antiplatelet therapy alone for percutaneous coronary intervention. Am J Cardiol. 2007;100(9):1376–82. doi:10.1016/j.amjcard.2007.06.024.
- Arce NA, Cao W, Brown AK, Legan ER, Wilson MS, Xu ER, Berndt MC, Emsley J, Zhang XF, Li R. Activation of von Willebrand factor via mechanical unfolding of its discontinuous autoinhibitory module. Nat Commun. 2021;12(1):2360. doi:10.1038/s41467-021-22634-x.
- Schneider SW, Nuschele S, Wixforth A, Gorzelanny C, Alexander-Katz A, Netz RR, Schneider MF. Shear-induced unfolding triggers adhesion of von Willebrand factor fibers. Proc Natl Acad Sci USA. 2007;104(19):7899–903. doi:10.1073/pnas.0608422104.
- Jen CJ, Li HM, Wang JS, Chen HI, Usami S. Flow-induced detachment of adherent platelets from fibrinogen-coated surface. Am J Physiol. 1996;270(1 Pt 2):H160–166. doi: 10.1152/ajpheart.1996.270.1.H160. PMID: 8769747.
- Denardo SJ, Parish A, Green CL, Oxendine-Phillips L, Tcheng JE. Antiplatelet therapy only, without anticoagulation, for percutaneous coronary intervention: a translational application of platelet slip. J Transl Sci. 2021;7(6):1–11. doi:10.15761/JTS.1000455.
- Zi W, Song J, Kong W, Huang J, Guo C, He W, Yu Y, Zhang B, Geng W, Tan, et al. Tirofiban for stroke without large or medium-sized vessel occlusion. N Engl J Med. 2023;388(22):2025–36. doi:10.1056/NEJMoa2214299.
- Smith SC Jr, Dove JT, Jacobs AK, Kennedy JW, Kereiakes D, Kern MJ, Kuntz RE, Popma JJ, Schaff HV, Williams DO. et al. ACC/AHA guidelines for percutaneous coronary intervention (revision of the 1993 PTCA guidelines)-executive summary: a report of the American College of Cardiology/American heart association task force on practice guidelines (Committee to revise the 1993 guidelines for percutaneous transluminal coronary angioplasty) endorsed by the society for cardiac angiography and interventions. Circulation. 2001;103(24):3019–41. doi:10.1161/01.cir.103.24.3019.
- Chieffo A, Burzotta F, Pappalardo F, Briguori C, Garbo R, Masiero G, Nicolini E, Ribichini F, Trani C, Álvarez BC. et al. Clinical expert consensus document on the use of percutaneous left ventricular assist support devices during complex high-risk indicated PCI: Italian society of interventional cardiology working group endorsed by Spanish and Portuguese interventional cardiology societies. Int J Cardiol. 2019;293:84–90. doi:10.1016/j.ijcard.2019.05.065.
- Mehran R, Rao SV, Bhatt DL, Gibson CM, Caixeta A, Eikelboom J, Kaul S, Wiviott SD, Menon V, Nikolsky E. et al. Standardized bleeding definitions for cardiovascular clinical trials: a consensus report from the bleeding academic research consortium. Circulation. 2011;123(23):2736–47. doi:10.1161/CIRCULATIONAHA.110.009449.
- Denardo S. PLATELETS_JTS_RETROSPECTIVE_DATA_N228_Biomark_Pos_NSTE_ACS_12072023 [Data set]. Zenodo. 2023. https://doi.org/10.5281/zenodo.10277693.
- Bikdeli B, Erlinge D, Valgimigli M, Kastrati A, Han Y, Steg PG, Stables RH, Mehran R, James SK, Frigoli E. et al. Bivalirudin versus heparin during PCI in NSTEMI: individual patient data meta-analysis of large randomized trials. Circulation. 2023;148(16):1207–19. doi:10.1161/CIRCULATIONAHA.123.063946.
- Sakariassen KS, Orning L, Turitto VT. The impact of blood shear rate on arterial thrombus formation. Future Sci OA. 2015;1(4):FSO30. doi:10.4155/fso.15.28.
- Wu YP, van Breugel HH, Lankhof H, Wise RJ, Handin RI, de Groot PG, Sixma JJ. Platelet adhesion to multimeric and dimeric von Willebrand factor and to collagen type III preincubated with von Willebrand factor. Arterioscler Thromb Vasc Biol. 1996;16(5):611–20. doi:10.1161/01.atv.16.5.611.
- Speich HE, Earhart AD, Hill SN, Cholera S, Kueter TJ, Smith JN, White MM, Jennings LK. Variability of platelet aggregate dispersal with glycoprotein IIb-IIIa antagonists eptifibatide and abciximab. J Thromb Haemost. 2009;7(6):983–91. doi:10.1111/j.1538-7836.2009.03432.x.
- Kajiya F, Matsuoka S, Ogasawara Y, Hiramatsu O, Kanazawa S, Wada Y, Tadaoka S, Tsujioka K, Fujiwara T, Zamir M. Velocity profiles and phasic flow patterns in the non-stenotic human left anterior descending coronary artery during cardiac surgery. Cardiovasc Res. 1993;27(5):845–50. doi:10.1093/cvr/27.5.845.
- Sedaghat A, Kulka H, Sinning JM, Falkenberg N, Driesen J, Preisler B, Hammerstingl C, Nickenig G, Pötzsch B, Oldenburg J. et al. Transcatheter aortic valve implantation leads to a restoration of von Willebrand factor (VWF) abnormalities in patients with severe aortic stenosis - Incidence and relevance of clinical and subclinical VWF dysfunction in patients undergoing transfemoral TAVI. Thromb Res. 2017;151:23–8. doi:10.1016/j.thromres.2016.12.027.
- Badimon L, Vilahur G. Platelets, arterial thrombosis and cerebral ischemia. Cerebrovasc Dis. 2007;24(Suppl 1):30–9. doi:10.1159/000107377.
- Storey RF, Judge HM, Wilcox RG, Heptinstall S. Inhibition of ADP-induced P-selectin expression and platelet-leukocyte conjugate formation by clopidogrel and the P2Y12 receptor antagonist AR-C69931MX but not aspirin. Thromb Haemost. 2002;88(3):488–94. doi: 10.1055/s-0037-1613242. PMID: 12353080.
- Virchow RLK. Gesammelte Abhandlungen zur Wissenschaftlichen Medizin. Kapitel IV [Thrombose und Embolie]. Frankfurt, Germany: Meidinger Sohn & Co; 1856.
- Stevens SM, Woller SC, Baumann Kreuziger L, Bounameaux H, Doerschug K, Geersing GJ, Huisman MV, Kearon C, King CS, Knighton AJ. et al. Executive summary: antithrombotic therapy for vte disease: second update of the CHEST guideline and expert panel report. Chest. 2021;160(6):2247–59. doi:10.1016/j.chest.2021.07.056.
- January CT, Wann LS, Calkins H, Chen LY, Cigarroa JE, Cleveland JC Jr, Ellinor PT, Ezekowitz MD, Field ME, Furie KL. et al. 2019 AHA/ACC/HRS Focused Update of the 2014 AHA/ACC/HRS guideline for the management of patients with atrial fibrillation: a report of the American college of cardiology/American heart association task force on clinical practice guidelines and the heart rhythm society in collaboration with the society of thoracic surgeons. Circulation. 2019;140(2):125–51. doi:10.1161/CIR.0000000000000665.
- Powers WJ, Rabinstein AA, Ackerson T, Adeoye OM, Bambakidis NC, Becker K, Biller J, Brown M, Demaerschalk BM, Hoh B. et al. 2018 Guidelines for the early management of patients with acute ischemic stroke: a guideline for healthcare professionals from the American heart association/American stroke association. Stroke. 2018;49(3):46–110. doi:10.1161/STR.0000000000000158.
- Amsterdam EA, Wenger NK, Brindis RG, Casey DE Jr, Ganiats TG, Holmes DR Jr, Jaffe AS, Jneid H, Kelly RF, Kontos MC. et al. 2014 AHA/ACC Guideline for the management of patients with non–st-elevation acute coronary syndromes: executive summary. Circulation. 2014;130(25):2354–94. doi:10.1161/CIR.0000000000000133.
- Levine GN, Bates ER, Blankenship JC, Bailey SR, Bittl JA, Cercek B, Chambers CE, Ellis SG, Guyton RA, Hollenberg SM. et al. 2011 ACCF/AHA/SCAI Guideline for percutaneous coronary intervention: a report of the American college of cardiology foundation/American heart association task force on practice guidelines and the society for cardiovascular angiography and interventions. Circulation. 2011;124(23):e574–651. doi:10.1161/CIR.0b013e31823ba622.
- Chen JY, He PC, Liu YH, Wei XB, Jiang L, Guo W, Duan CY, Guo YS, Yu XP, Li J. et al. Association of parenteral anticoagulation therapy with outcomes in Chinese patients undergoing percutaneous coronary intervention for non-st-segment elevation acute coronary syndrome. JAMA Internal Medicine. 2019;179(2):186–94. doi:10.1001/jamainternmed.2018.5953.
- Grüntzig A, Schneider HJ. Die perkutane Dilatation chronischer Koronarstenosen–Experiment und Morphologie [The percutaneous dilatation of chronic coronary stenoses–experiments and morphology]. Schweiz Med Wochenschr. 1977;107(44):1588. German. PMID: 918625.
- Stabile E, Nammas W, Salemme L, Sorropago G, Cioppa A, Tesorio T, Ambrosini V, Campopiano E, Popusoi G, Biondi Zoccai G. et al. The CIAO (Coronary Interventions Antiplatelet-based Only) study: a randomized study comparing standard anticoagulation regimen to absence of anticoagulation for elective percutaneous coronary intervention. J Am Coll Cardiol. 2008;52(16):1293–8. doi:10.1016/j.jacc.2008.07.026.
- Xiao Z, Théroux P. Platelet activation with unfractionated heparin at therapeutic concentrations and comparisons with a low-molecular-weight heparin and with a direct thrombin inhibitor. Circulation. 1998 Jan 27;97(3):251–6. 10.1161/01.cir.97.3.251. PMID: 9462526.
- Alexander KP, Chen AY, Roe MT, Newby LK, Gibson CM, Allen-LaPointe NM, Pollack C, Gibler WB, Ohman EM, Peterson ED. CRUSADE Investigators. Excess dosing of antiplatelet and antithrombin agents in the treatment of non-ST-segment elevation acute coronary syndromes. JAMA. 2005;294(24):3108–16. doi:10.1001/jama.294.24.3108.
- Brieger DB, Mak KH, Kottke-Marchant K, Topol EJ. Heparin-induced thrombocytopenia. J Am Coll Cardiol. 1998 June;31(7):1449–59. doi:10.1016/s0735-1097(98)00134-x. PMID: 9626819.
- [accessed 2023 December 7]. https://www.accessdata.fda.gov/drugsatfda_docs/label/2017/017029s140lbl.pdf.
- Sproul EP, Nandi S, Chee E, Sivadanam S, Igo BJ, Schreck L, Brown AC. Development of biomimetic antimicrobial platelet-like particles comprised of microgel nanogold composites. Regen Eng Transl Med. 2020;6(3):299–309. doi:10.1007/s40883-019-00121-6.
- Raben JS, Hariharan P, Robinson R, Malinauskas R, Vlachos PP. Time-resolved particle image velocimetry measurements with Wall Shear Stress and uncertainty quantification for the FDA nozzle model. Cardiovasc Eng Technol. 2016;7(1):7–22. doi:10.1007/s13239-015-0251-9.
- Zhu S, Diamond SL. Contact activation of blood coagulation on a defined kaolin/collagen surface in a microfluidic assay. Thromb Res. 2014;134(6):1335–43. doi:10.1016/j.thromres.2014.09.030.
- Feller T, Connell SDA, Ras A. Why fibrin biomechanical properties matter for hemostasis and thrombosis. J Thromb Haemost. 2022;20(1):6–16. doi:10.1111/jth.15531.
- Gaudino M, Yong CM, Chadow D, Lawton J, Tamis-Holland J. Coronary artery bypass surgery after transradial catheterization: Implementing 2021 ACC/AHA/SCAI revascularization guidelines into clinical practice. JACC Case Rep. 2022;4(1):27–30. doi:10.1016/j.jaccas.2021.09.026.
- Avvedimento M, Real C, Nuche J, Farjat-Pasos J, Galhardo A, Trinh KH, Robichaud M, Delarochellière R, Paradis JM, Poulin A. et al. Incidence, predictors, and prognostic impact of bleeding events after TAVR according to VARC-3 Criteria. JACC Cardiovasc Interv. 2023;16(18):2262–74. doi:10.1016/j.jcin.2023.07.005.
- Généreux P, Piazza N, Alu MC, Nazif T, Hahn RT, Pibarot P, Bax JJ, Leipsic JA, Blanke P, Blackstone EH. et al. Valve academic research consortium 3: updated endpoint definitions for aortic valve clinical research. J Am Coll Cardiol. 2021;77(21):2717–46. doi:10.1016/j.jacc.2021.02.038.
- Mehran R, Pocock SJ, Stone GW, Clayton TC, Dangas GD, Feit F, Manoukian SV, Nikolsky E, Lansky AJ, Kirtane A. et al. Associations of major bleeding and myocardial infarction with the incidence and timing of mortality in patients presenting with non-ST-elevation acute coronary syndromes: a risk model from the ACUITY trial. Eur Heart J. 2009;30(12):1457–66. doi:10.1093/eurheartj/ehp110.
- Coylewright M, Holmes DR, Kapadia SR, Hsu JC, Gibson DN, Freeman JV, Yeh RW, Piccini JP, Price MJ, Allocco DJ. et al. DAPT is comparable to OAC Following LAAC with WATCHMAN FLX: a national registry analysis. JACC Cardiovasc Interv. 2023;16(22):2708–18. doi:10.1016/j.jcin.2023.08.013.
- Grigoriadis GI, Sakellarios AI, Kosmidou I, Naka KK, Ellis C, Michalis LK, Fotiadis DI. Wall shear stress alterations at left atrium and left atrial appendage employing abnormal blood velocity profiles. Annu Int Conf IEEE Eng Med Biol Soc. 2020;2020:2565–8. doi:10.1109/EMBC44109.2020.9175235.
- Mehra MR, Netuka I, Uriel N, Katz JN, Pagani FD, Jorde UP, Gustafsson F, Connors JM, Ivak P, Cowger J. et al. Aspirin and hemocompatibility events with a left ventricular assist device in advanced heart failure: The ARIES-HM3 randomized clinical trial. JAMA. 2023;330(22):2171–81. doi:10.1001/jama.2023.23204.
- Bourque K, Cotter C, Dague C, Harjes D, Dur O, Duhamel J, Spink K, Walsh K, Burke E. Design rationale and preclinical evaluation of the HeartMate 3 left ventricular assist system for hemocompatibility. Asaio J. 2016;62(4):375–83. doi:10.1097/MAT.0000000000000388.
- Tamez H, Généreux P, Yeh RW, Amin AP, Fan W, White HD, Kirtane AJ, Stone GW, Gibson CM, Harrington RA. et al. Cost implications of intraprocedural thrombotic events and bleeding in percutaneous coronary intervention: results from the CHAMPION PHOENIX ECONOMICS Study. Catheter Cardiovasc Interv. 2018;92(5):348–55. doi:10.1002/ccd.27638.
- Jacobson KM, Hall Long K, McMurtry EK, Naessens JM, Rihal CS. The economic burden of complications during percutaneous coronary intervention. Qual Saf Health Care. 2007;16(2):154–9. doi:10.1136/qshc.2006.019331.
- Hershey D, Cho SJ. Blood flow in rigid tubes: thickness and slip velocity of plasma film at the wall. J Appl Physiol. 1966;21(1):27–32. doi:10.1152/jappl.1966.21.1.27.