Abstract
A survey of fungal contamination and presence of aflatoxins (AFs) and fumonisins (FBs) in 30 feed samples collected from 10 tilapia farms during three seasons in Nayarit State, located in north-western Mexico, was carried out using ELISA as screening and High-Performance Liquid Chromatography (HPLC) as confirmatory method. Mycobiota included Aspergillus flavus and Fusarium spp. AFs were detected in 63.3% of samples using ELISA, but confirmation by HPLC revealed that all samples were under the detection limit. Regarding to FBs, positive samples were detected using both methods, with 19 positive samples (60% of total) by ELISA and 14 positive samples (46.6% of total) by HPLC and levels ranging from 0.148 to 2.587 mg/kg. Correlation was observed between both methods (r = 0.516, p = 0.004) for FBs results. No sample exceeded the European maximum levels for any of the mycotoxins. Water activity of samples ranged from 0.345 to 0.655, suggesting that mycotoxin occurrence is probably related to raw material contamination.
1. Introduction
Mycotoxins are toxic substances, which are produced by filamentous fungi as secondary metabolites. Natural contamination of food and feed with mycotoxins is a common fact, and affects from raw materials to final products throughout the manufacturing process and even at transporting and storage. Mycotoxin production depends not solely on environmental conditions, but also on the products water activity (aw) and composition (Sweeney & Dobson, Citation1998). Tropical and subtropical climates, such as the Pacific coast of Mexico, offer temperate and ideal conditions for the development of endemic fungal genera such as Aspergillus and Fusarium (Coker, Citation1997). These genera include well-documented aflatoxins (AFs) and fumonisins (FBs) producing species, respectively (D'Mello & McDonald, Citation1997). Fungal contamination affects on the nutritional overall value of feed, and represents a serious risk to animal health. High levels of mycotoxins can cause decay and death, whereas, low levels can provoke asymptomatic chronic effects, as mycotoxin consumption triggers a varied range of collateral diseases resulting in decrease of animal production. AFs affect on a plethora of farm animals with commercial importance such as poultry (Leeson, Diaz, & Summers, Citation1995), swine, several monogastrics (Smith, Griffin, & Hamilton, Citation1976) and fish (Santacroce et al., Citation2008). However, FBs have swine and horses as main targets, but also could reach birds and fish (Denli & Pérez, Citation2006). Secondary mycotoxicosis can also take place through the trophic chain. The International Agency for Research on Cancer (IARC, Citation1993) considers aflatoxin B1 (AFB1) and aflatoxin M1 (AFM1) as carcinogenic and fumonisin B1 (FB1) as potentially carcinogenic.
In Mexico, Nile tilapia (Oreochromis niloticus) is cultured under extensive and intensive methods. Since, this organism has shown great adaptability to virtually all environmental conditions present along this territory and has become the main fishery within continental waters in this country (Fitzsimmons, Citation2000). Besides, its great adaptation skills, tilapia can survive on low oxygen concentration scenarios and high salinity intervals without any problem and still maintain its top productivity ratio (Zaitseva, Santerre-Lucas, Casas-Solis, Peregrina-Sandoval, & León-Sánchez, Citation2006). However, common health problems can affect this organism causing mortalities and reducing growth during cultivation, including parasite microorganisms (Jiménez et al., Citation1988), or toxic compounds, such as mycotoxins (Nguyen, Grizzle, Lovell, Manning, & Rottinghaus, Citation2002).
Several studies have been performed dealing with contaminants on corn-based food and feed, mostly on the mycotoxin issue (Pittet, Citation1998), since maize meal is present in at least 27% of all fish feed formulations as crude protein (Food and Agriculture Organization of the United Nations [FAO], Citation1980) and some commercial tilapia feeds contain up to 45% protein (Cargill, Incorporated, Citation2011).
Since AFs and FBs have different toxicity mechanisms at cellular level, determination of the contamination level on tilapia feed could provide valuable information (1) to set the concentration to use in further acute or chronic toxicity tests for tilapia, or any other teleost research model and (2) to predict future effects on the feeding organism. Several studies have surveyed mycotoxins in maize-based feed but few or none have dealt with the rating of fish feed as in the present work.
The aim of this study was to determine the main fungal contamination of tilapia feed samples from Nayarit (Mexico) and the contamination level of AFs and FBs, using an ELISA method as screening with a later HPLC confirmatory determination.
2. Materials and methods
Feed samples from 10 of the major tilapia farms from the north-western state of Nayarit, Mexico () were taken during three seasons, comprising from September 2009 to April 2010. Sampling was carried out following the European Union Commission Regulation 401/2006 regarding mycotoxin sampling (European Union Commission, Citation2006a). Samples were obtained directly from tilapia farms located in six municipal populations (Tecuala, Santiago Ixcuintla, San Blas, Tepic, Santa María del Oro and Bahía de Banderas) using a calibrated probe with an average weight of 1 kg. Samples were on the pellet form with different sizes, depending on the feed manufacturer and protein content. They were finely ground and homogenised for both mycological and analytical analysis. For the grinding process, a Romer Analytical Sampling Mill (RAS® Mill; Coring-System Diagnostix GmbH, Gernsheim, Germany) was used.
2.1. Water activity determination
Water activity of samples was determined using an Aqualab® water activity meter (Decagon Devices®) before storage at –20°C for further determinations.
2.2. Mycological analysis
Total fungal counts were performed on dichloran rose-bengal chloramphenicol (DRBC) medium, a general medium used for estimating total culturable fungi, dichloran 18% glycerol agar (DG18), a medium with lower water activity (aw=0.955) that favours xerophilic fungi development, and malachite green (MG) medium, a medium specifically designed for isolation of Fusarium spp. (Castellá, Bragulat, Rubiales, & Cabañes, Citation1997). Quantitative enumeration was done using the surface-spread method. Ten grams of each sample were homogenised in 90 ml of peptone water solution. Serial dilutions were made and 0.1 ml aliquots were inoculated in duplicate onto the culture media. All the plates were incubated in the dark at 25°C for 7 days, except for DG18 cultures that were extended up to 15 days. Only plates containing 15–150 colony forming units (CFU) were used for counting and the results were expressed as CFU per gram of sample. All colonies were transferred for sub-culturing to plates of Czapek yeast extract agar and incubated 7 days at 25°C. Taxonomic identification of the different genera was made according to macroscopic and microscopic criteria with appropriate keys (Pitt & Hocking, Citation1997), using different media as malt extract agar, Czapek medium (CZ), glycerol 25% nitrate agar (G25N) and coconut agar medium.
2.3. ELISA screening test
Fifty grams of ground sample were extracted with 250 ml of 70% methanol by mixing vigorously on a magnetic stirrer for 3 minutes. After filtering the extract through a Whatman No. 1 filter, the extract was diluted 1:1 (AFs) or 1:14 (FBs) with deionised water, and mycotoxin analysis was developed using a competitive ELISA commercial kit for total AFs (RIDASCREEN® Aflatoxin Total; R-Biopharm AG, Darmstadt, Germany) or total FBs (RIDASCREEN® Fumonisin; R-Biopharm AG, Darmstadt, Germany) strictly following manufacturer's procedure. Detection limits of the kits were 1.75 µg/kg (AFs) and 0.222 mg/kg (FBs).
2.4. Mycotoxins analysis by HPLC
For AFs analysis, 5 g of previously ground and homogenised feed sample were mixed with 15 ml of methanol/water (60/40 v/v) on a magnetic stirrer for 10 minutes. After filtering, 2 ml of extract was diluted with 14 ml of phosphate buffered saline (PBS) and purified by using an immunoaffinity column (Easy-extract® Aflatoxin; R-Biopharm Rhône Ltd., Glasgow, Scotland) following manufacturer's procedure. For FBs analysis, 5 g of sample was mixed with 0.5 g of sodium chloride and 25 ml of methanol/acetonitrile/water (25/25/50 v/v) and magnetically stirred for 20 minutes. Supernatant was filtered and 10 ml of extract was diluted with 40 ml of PBS and purified through an immunoaffinity column (Fumoniprep®; R-Biopharm Rhône Ltd., Glasgow, Scotland) strictly using the manufacturer's procedure.
For AFs HPLC analysis, mobile phase was water:methanol:acetonitrile (70:17:17) pumped at 1.0 ml/minute through a Symmetry® C18 steel column connected to a Waters Spherisorb® S5ODS2 precolumn. A LC Tech photochemical derivatizer (Dorfen, Germany) was placed between the column and a Waters Multi λ Fluorescence Detector 2475 set to an excitation wavelength of 362 nm and an emission wavelength of 440 nm. The injection volume was 100 µl, and the detection limit of the method was 0.0788 µg total AFs/kg.
In the case of FBs HPLC analysis, mobile phase was methanol:sodium dihydrogen phosphate 0.1 M (77:23) pH 7.4 pumped at 1.0 ml/minute, using a Spherisorb® ODS2 steel column connected to a Spherisorb® S50DS2 pre-column. Waters Multi λ Fluorescence Detector 2475 was set to an excitation wavelength of 335 nm and an emission wavelength of 440 nm. Approximately 50 µl of the extract was derivatised with 450 µl o-phthaldialdehyde reagent, prepared according to the method of Shephard, Sydenham, Thiel, and Gelderblom (Citation1990). The samples were injected into HPLC within 1 minute after derivatisation. The injection volume was 100 µl and the detection limit was 0.1576 mg total FBs/kg.
2.5. Statistical analysis
Data results were checked for normal distribution, followed by a post hoc test (Pearson's Test) for correlation between ELISA and HPLC values. The SigmaStat 3.5 (Systat Software Inc., Chicago, IL, USA) software package was used for statistical purposes.
3. Results and discussion
3.1. Fungal contamination of samples
Water activity of samples ranged from 0.345 to 0.655. At this aw, fungal growth is extremely difficult (only possible for strictly xerophilic strains in the upper limit). These values are far from suitable conditions for mycotoxin production, since most toxin producing genera synthesise mycotoxins in the 0.85–0.97 aw range and only in some exceptional cases can produce them poorly in the 0.77–0.88 range (Sweeney & Dobson, Citation1998).
Fungal contamination found in samples was usually low, mainly composed by yeasts and some species of Mucorales. With regard to mycotoxigenic fungi, Aspergillus flavus was detected only in two samples and Fusarium spp. was isolated also from two different samples (), representing a 6.7% contamination rate in both cases.
Table 1. Total counts, water activity and isolated genera/species from tilapia feeds from Nayarit, Mexico.
The low aw found in these products, together with the heat treatment suffered during feed production, could explain the low fungal contamination found, as pre-existing mycobiota from raw materials can hardly survive the conditions which take place during feed processing, due to the heat and pressure involved in pellet making. However, although fungal counts were low, the presence of mycotoxins cannot be excluded, as they are more likely pre-harvest contaminants of feed raw materials and their thermal stability can allow them to resist the feed manufacturing process.
3.2. Mycotoxins in tilapia feed samples
Aflatoxins were detected in 63.3% of the samples on ELISA assays but when confirmed by HPLC all samples were reported as negative or below detection limit, although the detection limit in the latter case was lower (data not shown). With regard to FBs, these toxins were detected by ELISA (19 positive samples, 60% of total) as well as by HPLC (14 positive samples, 46.6% of total), with contamination ranging from 0.148 to 2.587 mg FBs/kg. No sample exceeded the European maximum levels for AFs in complete feeding stuffs, according to Commission Directive 2003/100/EC, or the recommended maximum levels for FBs, according to 2006/576 Commission Recommendation (European Union Commission, Citation2003, Citation2006b). There was no seasonal trend in mycotoxin contamination of samples ().
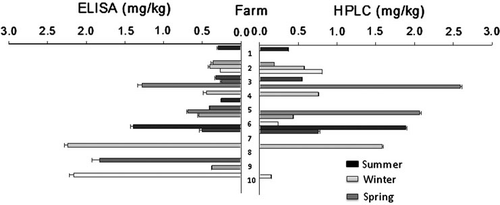
ELISA methods are nowadays commonly used as screening tools for mycotoxins contamination, however, discrepancies on aflatoxin values between ELISA and HPLC could be related to the detection limit set on the screening test (1.75 µg/kg) since the results were close to such limit. This particular issue has been in discussion fairly often and in different studies, reporting higher values obtained by ELISA kits than those obtained in HPLC analysis (Rojas-Jaimes, Citation2008). A correlation between methods after fumonisin determination was assessed (r=0.516, p = 0.004), once again with ELISA values being higher. Other studies also on fumonisin reported similar correlation data that vary from 0.51 (p<0.05; Pestka et al., Citation1994) to 0.97 (p<0.001; Sydenham et al., Citation1996). Overall, this type of comparisons have generally shown a trend for the concentrations of total FBs determined with ELISA to be greater than those determined in the same samples by HPLC. Nevertheless, ELISA as screening test eases the task of selection of the number of samples to confirm on the more elaborate and time consuming quantitative method of HPLC. Finally, also on FBs values, no correlation was observed between the reported aw and mycotoxin results.
Since tilapia feed could fit as a maize-based by-product, one of the major factors that determine its final mycotoxin concentration is whether a dry or wet maize milling process is used (Stack & Eppley, Citation1992). Analysis of the fractions obtained from laboratory-scale wet milling of maize contaminated with FBs revealed that FBs content in the gluten, fibre and germ fractions represented 10–40% of the concentration found in the original maize, and diminished in the order gluten > fibre > germ (Patel, Hazel, Winterton, & Gleadle, Citation1997). Although milling and pellet making represent a critical point in mycotoxin contamination, in Mexico little can be done by the tilapia producers, since these consideration escapes from their control; however, postpellet-manufacturing stages that could be controlled by fish producers include transportation and storage.
Transportation is an in-between stage in which temperature changes due to day–night and seasonal variations could cause condensation inside the inner container walls; this is a fair reason to keep a humidity level below 12%, or else an extra inner protection on feed container is recommended (Manning, Citation2010). Fish feed storage recommendations include room specification like the using of tilt ceiling and solid impermeable walls, wood or triply ground base with a 1.5- to 2.0-m distance from real ground and a physical and visual analysis on feed surface on a weekly basis (Arias, Citation1993). As to aforementioned critical stages that depends mostly on the tilapia producer considerations, on this particular study, it was found that few, if any, of these precautions were usually taken into account, since only a few of the facilities had a specially assigned storage room. They did not have the measures recommended; in some cases, storage places included indoors accommodation of families and pets.
Taking into account, the aw range observed in our samples (0.345–0.655), far from the minimum values for the production of FBs, usually never lower than 0.77 (Sweeney & Dobson, Citation1998), the presence of the studied mycotoxins in our samples indicates a pre-processing contamination, that is, a contamination of the raw material. As detailed above, heat stability may allow mycotoxins to resist the processing steps. Stability of mycotoxins during food processing is affected by many factors, including the moisture content of the product, the toxin concentration and its location, the presence of additives and the type of food matrix (Scott, Citation1984). These factors should, therefore, be considered with respect to each type of processing (e.g. milling, roasting, canning, oil extraction) when estimating the fate of the mycotoxin. After decomposition or destruction of mycotoxins, the resulting compound(s) should be identified to ensure that undesirable materials (with harmful biological effects) do not contaminate the food.
The role of mycotoxins in aquaculture is still poorly understood and only few studies have been developed on their acute effects. The chronic effect of these toxic compounds on fishes has been even more scarcely studied. Recently a deep review on the AFB1 toxic effects on immune response on teleost fishes has been published (Rodríguez-Cervantes et al., Citation2010) and data on AFB1 toxicity to tilapia are very scarce. Exposure to AFB1 has resulted in a reduced phagocytic capacity of the macrophages of Nile tilapia, as seen in the research of El-Enbaawy, Adel, Marzouk, and Salem (Citation1994).
With regard to FBs, early studies revealed that FB1 is toxic to tilapia and channel catfish by suppressing growth and/or causing histopathological lesions. However, the fish survive dietary levels up to 150 mg/kg of this mycotoxin. Reduction of the percentage of survival of channel catfish was observed for diets containing 240 mg/kg FB1 (Li, Raverty, & Robinson, Citation1994). Results reported by Tuan, Manning, Lovell, and Rottinghaus (Citation2003) demonstrated that adding FB1 up to 150 mg/kg to fish feed for 8 weeks affected growth performance of Nile tilapia fingerlings. Adverse effects of fumonisin contaminated diets have also been reported in carps. One-year carps indicated that signs of toxicity can be observed with 10 mg FB1/kg feed (Petrinec, Pepeljnjak, Kovacic, & Krznaric, Citation2004). In these experiments scattered lesions in the exocrine and endocrine pancreas, and inter-renal tissue, probably due to ischemia and/or increased endothelial permeability were reported. In 1-year old carps, consumption of pellets contaminated with 0.5 and 5.0 mg FB1 per kg body weight resulted in a loss of body weight and alterations of haematological and biochemical parameters in target organs (Pepeljnjak, Petrinec, Kovacic, & Segvic, Citation2003).
Considering the results obtained in this study, the FBs levels were far from being in the acutely toxic and lethal range for several species. Nevertheless, low concentrations could induce silent repercussions chronically (Arkoosh & Kaattari, Citation1987; El-Enbaawy et al., Citation1994; Ottinger & Kaattari, Citation2000; Sahoo & Mukherjee, Citation2001).
4. Conclusion
This study, the first on mycotoxin contamination of fish feedstuffs developed in Mexico, has shown that fungal contamination can occur, but it is low and mainly composed by yeast and some other non-mycotoxigenic fungi. As for aflatoxin and fumonisin producers, A. flavus and Fusarium spp. were detected and isolated in only two samples. AFs were detected in 63.3% of the samples by ELISA but were reported as negative or below the detection limit by confirmatory HPLC. However, FBs were detected in higher levels by ELISA (19 positive samples, 60% of total) than by HPLC (14 positive samples, 46.6% of total), with contamination ranging from 0.148 to 2.587 mg FBs/kg.
Aflatoxins and FBs contamination of tilapia feeds, despite the season and the often inadequate storage conditions, did not reach worrying levels. Thus, in no case did contamination reach values exceeding the maximum tolerable (AFs) or recommended (FBs) limits according to European standards. It was relatively common to find occurrence of both types of mycotoxins, which suggests a horizon of concern given the possible synergistic effect of both toxins. Given the low water activity of the feeds tested, mycotoxin contamination must have been probably caused by contamination of the raw material that resisted the feed manufacturing process. The present survey represents a starting point for analysis of the fish feed matrix, an essential analytical need because of the ever-growing importance of aquaculture.
Acknowledgements
The authors wish to thank Programa Iberoamericano de Ciencia y Tecnología para el Desarrollo (109AC0371 CYTED Action) for the financial support. C.H. Rodríguez-Cervantes wishes to thank the University of Lleida for the mobility grant awarded. The first author is currently a student in the CBAP Ph.D. Program from Nayarit Autonomous University and received a CONACyT scholarship through the project Nayarit-2008/C01/93389.
References
- Arias, C. (1993). Manual de manejo postcosecha de granos a nivel rural [Handbook of Post-harvest management of grains at agrarian level]. Santiago, Chile: Oficina Regional de la FAO para América Latina y el Caribe.
- Arkoosh, M.R., & Kaattari, S.L. (1987). Effect of early aflatoxin B1 exposure on in vivo and in vitro antibody responses in rainbow trout, Salmo gairdneri. Journal of Fish Biology, 31, 19–22.
- Cargill, Incorporated. (2011). Purina. Alimentos para peces de clima cálido. Tilapia. Retrieved October 2011, from http://www.nutrimentospurina.com.mx/Screens/gruposproductospeces/desarrollo.aspx
- Castellá, G., Bragulat, M.R., Rubiales, M.V., & Cabañes, F.J. (1997). Malachite green agar, a new selective medium for Fusarium spp. Mycopathologia, 137, 173–178.
- Coker, R.D. (1997). NRI Bulletin 73. Chatman: Natural Resources Institute.
- Denli, M., & Pérez, J.F. (2006). Contaminación por micotoxinas en los piensos: Efectos, tratamiento y prevención. Paper presented at XXII Curso de especialización FEDNA, Barcelona, Spain.
- D'Mello, J.P.F., & McDonald, A.M.C. (1997). Mycotoxins. Animal Feed Science and Technology, 69, 155–166.
- El-Enbaawy, M., Adel, M., Marzouk, M.S., & Salem, A.A. (1994). The effects of acute and chronic aflatoxicosis on the immune functions of Oreochromis niloticus in Egypt. Veterinary Medical Journal Giza, 42, 47–52.
- European Union Commission. (2003). Commission Directive 2003/100/EC of 31 October 2003 amending Annex I to Directive 2002/32/EC of the European Parliament and of the Council on undesirable substances in animal feed. Official Journal of the European Union, L285, 33–37.
- European Union Commission. (2006a). Commission Regulation (EC) No 401/2006 of 23 February, laying down the methods of sampling and analysis for the official control of the levels of mycotoxins in foodstuffs. Official Journal of the European Union, L70, 12–34.
- European Union Commission. (2006b). Commission Recommendation 2006/576/EC of 17 August 2006 on the presence of deoxynivalenol, zearalenone, ochratoxin A, T-2 and HT-2 and fumonisins in products intended for animal feeding. Official Journal of the European Union, L229, 7–9.
- Fitzsimmons, K. (2000). Tilapia aquaculture in Mexico. In B.A. Costa-Pierce & J.E. Rakocy (Eds.), Tilapia aquaculture in the Americas (Vol. 2, pp. 171–183). Baton Rouge, Louisiana: The World Aquaculture Society.
- Food and Agriculture Organization of the United Nations. (1980). Fish feed technology. Lectures presented at the FAO/UNDP Training Course in Fish Feed Technology. Seattle, WA: College of Fisheries, University of Washington.
- International Agency for Research of Cancer (IARC). (1993). Aflatoxins. In IARC Monographs on the evaluation of carcinogenic risks to humans Vol. 56, pp. 245–395. Lyon: Author
- Jiménez, F., Garza, H.F., Segovia, F.S., Galaviz, L.S., Iruegas, F.B. Manuel J.A., et al. (1988). Parásitos y enfermedades de la tilapia (Publicación técnica No. 3)Nuevo León, México: Universidad Autónoma de Nuevo León.
- Leeson, S., Diaz, G.J., & Summers, J.D. (1995). Poultry metabolic disorders and mycotoxins, (pp. 249–298). Guelph, Ontario: University Books.
- Li, M.H., Raverty, S.A., & Robinson, E.H. (1994). Effects of dietary mycotoxins produced by the mold Fusarium moniliforme on channel catfish (Ictalurus punctatus). Journal of the World Aquaculture Society, 25, 512–516.
- Manning, B.B. (2010). Mycotoxins in aquaculture feeds. Mississippi: Southern Regional Aquaculture Center Publication.
- Nguyen, A., Grizzle, J., Lovell, B., Manning, B., & Rottinghaus, G. (2002). Growth and hepatic lesions of Nile Tilapia (Oreochromis niloticus) fed diets containing aflatoxin B1. Aquaculture, 212, 311–319.
- Ottinger, C.A., & Kaattari, S.L. (2000). Long-term immune dysfunction in rainbow trout (Oncorhynchus mykiss) exposed as embryos to aflatoxin B1. Fish and Shellfish Immunology, 10, 101–106.
- Patel, S., Hazel, C.M., Winterton, A.G.M., & Gleadle, A.E. (1997). Surveillance of fumonisins in UK maize-based foods and other cereals. Food Additives and Contaminants, 14, 187–191.
- Pepeljnjak, S., Petrinec, Z., Kovacic, S., & Segvic, M. (2003). Screening toxicity study in young carp (Cyprinus carpio L.) on feed amended with fumonisin B1. Mycopathologia, 156, 139–145.
- Pestka, J.J., Azcona-Olivera, J.I., Plattner, R.D., Minervini, F., Doko, M.B., & Visconti, A. (1994). Comparative assessment of fumonisin in grain-based foods by ELISA, GC–MS, and HPLC. Journal of Food Protection, 57, 169–172.
- Petrinec, Z., Pepeljnjak, S., Kovacic, S., & Krznaric, A. (2004). Fumonisin B1 causes multiple lesions in common carp (Cyprinus carpio). Deutsche Tierarztliche Wochenschrift, 111, 358–363.
- Pitt, J.I., & Hocking, A.D. (1997). Fungi and food spoilage (2nd ed.). London: Blackie Academic Press.
- Pittet, A. (1998). Natural occurrence of mycotoxins in foods and feeds. Revue de Médecine Vétérinaire, 149, 479–492.
- Rodríguez-Cervantes, C.H., Ramos, A.J., Robledo-Marenco, M.L., Marín, S., Velázquez-Fernández, J.B., & Medina-Díaz, I.M., et al. (2010). Aflatoxin B1 and its toxic effects on immune response on teleost fishes: a review. World Mycotoxin Journal, 3, 193–199.
- Rojas-Jaimes, J. (2008). Evaluation of the level of contamination by the “ochratoxine” mycotoxine in samples of Capsicum annuum “Paprika” using ELISA and high Performance liquid chromatography “HPLC”. Revista Horizonte Médico, 8, 48–52.
- Sahoo, P.K., & Mukherjee, S.C. (2001). Immunosuppresive effects of aflatoxin B1 in Indian major carp (Labeo rohita). Comparative Immunology, Microbiology and Infectious Diseases, 24, 143–149.
- Santacroce, M.P., Conversano, M.C., Casalino, E., Lai, O., Zizzadoro, C., Centoducati, G., et al. (2008). Aflatoxins in aquatic species: metabolism, toxicity and perspectives. Reviews in Fish Biology and Fisheries, 18, 99–130.
- Scott, P.M. (1984). Effect of food processing on mycotoxins. Journal of Food Protection, 47, 489–499.
- Shephard, G.S., Sydenham, E.W., Thiel, P.G., & Gelderblom, W.C.A. (1990). Quantitative determination of fumonisins B1 and B2 by high-performance liquid chromatography with fluorescence detection. Journal of Liquid Chromatography, 13, 2077–2087.
- Smith, R.B., Griffin, J.M., & Hamilton, P.B. (1976). Survey of aflatoxicosis in farm animals. Applied and Environmental Microbiology, 31, 385–388.
- Stack, M.E., & Eppley, R.M. (1992). Liquid chromatographic determination of fumonisin B1 and B2 in corn and corn products. Journal of the Association of Official Analytical Chemists International, 75, 834–837.
- Sweeney, M.J., & Dobson, D.W. (1998). Mycotoxin production by Aspergillus, Fusarium and Penicillium species. International Journal of Food Microbiology, 43, 141–158.
- Sydenham, E.W., Stockenström, S., Thiel, P.G., Rheeder, J.P., Doko, M.B., Bird, C., et al. (1996). Polyclonal antibody-based ELISA and HPLC methods for the determination of fumonisins in corn: A comparative study. Journal of Food Protection, 59, 893–897.
- Tuan, N.A., Manning, B.B., Lovell, R.T., & Rottinghaus, G.E. (2003). Responses of Nile tilapia (Oreochromis niloticus) fed diets containing different concentrations of moniliformin or fumonisin. Aquaculture, 217, 515–528.
- Zaitseva, G.P., Santerre-Lucas, A., Casas-Solis, J., Peregrina-Sandoval, J., & León-Sánchez, R. (2006). Tilapia: aspectos biológicos y productivos (pp. 37–52. Guadalajara, Jalisco, México: Universidad de Guadalajara