Abstract
Immunomodulatory and radioprotective properties of Haberlea rhodopensis extract (HRE) in relation to the primary and secondary specific antibody production were evaluated in New Zealand white rabbits. Rabbits were immunised with keyhole limpet hemocyanin (KLH) and were treated with HRE before irradiation with single dose of 2Gy. Control group without irradiation were also treated with HRE. Rabbits were reimmunised and treated again with HRE on Day 36. KLH-specific total IgM, G, A and KLH-specific IgG antibody production were determined during primary and secondary antibody response by enzyme-linked immunosorbent assay (ELISA). We observed a well-defined γ-radiation-induced immunosuppression on the total primary antibody production. Application of HRE restored primary total KLH-specific antibody as well as significantly increased primary KLH-specific IgG antibody response. We also established that HRE strongly enhanced KLH-specific antibody production after reimmunisation. We concluded that HRE exhibits immunostimulatory properties and could play protective role against γ-irradiation damage of immune response.
Introduction
The immune system plays a central role in host defence against infectious micro-organisms. In many cases during weakened and ineffective defence, immunomodulating agents are used as an alternative therapy. Exogenous agents that are capable of interacting with the immune system to upregulate or downregulate specific aspects of the host immune response can be classified as immunomodulators (Tzianabos, Citation2000). Plant extracts are the attractive sources of new immunomodulating drugs. A large number of plant extracts and their isolated constituents have shown beneficial therapeutic effects, including antioxidant, anti-inflammatory, anticancer, antimicrobial and immunomodulatory effects (Sharififar, Pournourmohammadi, & Arabnejad, Citation2009).
Ionising radiation interacts with biological systems to produce reactive oxygen species and reactive nitrogen species which attack various cellular components. These reactive species damage various biomacro-molecules, like the DNA, lipids and proteins (Shirazi, Mihandoost, Mahdavi, & Mohseni, Citation2012). High-dose ionising radiation (≥1Gy; Manda, Glasow, Paape, & Hildebrandt, Citation2012) leads to immune suppression due to the killing of large numbers of immune cells, especially dividing cells in both antigen independent and antigen-dependent phase of immune response. Past research on synthetic radioprotectors have little success as these have failed in the transition to human applications due to toxicity and increasing risk of side effects (Yamini & Gopal, Citation2010). Recently published studies showed that certain medicinal herbs and their active constituents may be useful as radioprotective agents because they are less toxic than their non-herbal counterparts. Plant constituents, especially those with free radical-scavenging antioxidant properties, and immunostimulatory effects have been extensively evaluated for their radioprotective effects (Arora et al., Citation2005; Baliga & Rao, Citation2010; Mishima et al., Citation2004).
Haberlea rhodopensis (Friv.) is a Ballkan endemic relict that grows in rocky areas in the Rhodope Mountains and some regions of the Sredna Gora and Balkan Mountains. Haberlea rhodopensis belongs to the group of extremely desiccation-tolerant (resurrection) plants which are capable of withstanding long periods of almost full desiccation and recover quickly after rehydration. Carbohydrates and phenols have been found to play an important role in the survival of plants under extreme conditions (Müller, Sprenger, Bortlik, Boller, & Wiemken, Citation1997). Phenolic compounds accumulated in high amounts in resurrection plants are assumed to protect their membranes against desiccation and free radical-induced oxidation (Sgherri, Stevanovic, & Navari-Izzo, Citation2004).
The total extract of H. rhodopensis was found to possess strong antioxidant and antibacterial activities (Radev et al., Citation2009). Recently, Berkov et al. (Citation2011) have revealed more than 100 compounds (amino acids, fatty acids, phenolic acids, sterols, glycerides, saccharides, etc.) and five ingredients possessing antiradical activity – syringic, vanillic, caffeic, dihydrocaffeic and p-coumaric acids. The main compounds isolated from H. rhodopensis are myconoside, paucifloside and flavone 8-C glycosides (Ebrahimi, Gafner, Dell'Acqua, Schweikert & Hamburger, Citation2011; Jensen, Citation1996). Moreover, evidence showed that myconoside have strong antioxidant and hepatoprotective effect (Kondeva-Burdina, Zheleva-Dimitrova, Nedialkov, Girreser, & Mitcheva, Citation2013). Further, a significant linear relationship was found between the antioxidant activity and the phenolic content, indicating that phenolic compounds are major contributors to the antioxidant activity of HRE (Mihailova, Bahchevanska, & Toneva, Citation2011). The antimutagenic potential of H. rhodopensis was reported in a previous study (Popov, Radev, & Georgieva, Citation2010). The present study investigated the immunostimulatory and radioprotective properties of a HRE with regard to the specific primary and secondary antibody response in rabbits.
Materials and methods
Preparation of an ethanolic extract of H. rhodopensis (HRE)
Leaves of H. rhodopensis were collected from plants growing in their natural habitat (the vicinity of Bachkovo, Bulgaria) during the flowering period in May–June. Leaves were cut into small pieces and were dried at room temperature for 1 month. After grinding, the dry matter was macerated for 6 h in 70% ethyl alcohol and was percolated for 48 h. The primary extract was concentrated by evaporation of ethanol in a vacuum environment in order to reach a ratio of 5% ethanol and 95% water. The obtained extract was filtered through a 0.25 µm cellulose-acetate membrane to remove emulsified substances, chlorophyll and other particles. The amount of extracted substance was between 0.098 and 0.142 g/cm3 (average 0.120 g/cm3). According to the toxicity tests in mice performed in laboratory LD50 of HRE was >1250 mg/kg body weight. In this study, a dose of 1/10 of 1250 mg/kg was used.
Experimental animals
Twenty male New Zealand white rabbits (bred in the animal facilities of the Agricultural Faculty of Trakia University) were used in the study. They were healthy, 5-month-old rabbits with a body weight between 3.5 and 4.0 kg at the beginning of the experiment. The experimental protocol was approved by the Department of Animal Care in conformity with the European Community Guiding Principles for the Care and Use of Animals. The animals were fed on a standard rabbit diet, had access to water ad libitum and were synchronised by maintaining controlled environmental conditions (light, temperature, feeding time, etc.) for at least two weeks prior to the beginning of the experiment.
Immunisation protocol
Primary antibody response
A subcutaneous injection of 50 µg keyhole limpet hemocyanin (KLH) from Megatura crenulata (Sigma) as an antigen in 0.5 ml saline was done to each rabbit on Day 0. Booster doses of 50 µg KLH in saline were administered on Day 8.
Secondary antibody response
At the end of the primary response, reimmunisation dose of 50 µg KLH in 0.5 ml saline was administered to each rabbit on Day 36.
Immunomodulation protocol
Rabbits were treated 24 h before and simultaneously with the first immunisation with HRE (120 mg/kg, each time). Along with the reimmunisation, they were treated again with 2 × 120 mg/kg HRE administered intramuscularly.
Irradiation protocol
Animals were exposed to a single dose of γ-radiation of 2Gy 2 h before antigen challenge. This dose was chosen because it is the threshold for high-dose irradiation (HD-IR≥1) (Manda et al., Citation2012) to produce immune suppression without any mortality. The source of radiation was a cobalt teletherapy unit (Rocus M, 60Co) at the Inter-District Cancer Dispensary, Stara Zagora, Bulgaria. Animals were irradiated individually in specially designed, well-ventilated wood cages without anaesthesia and exposed to whole body radiation at a dose rate 89.18 cGy/min.
Study design
Rabbits were divided into the following four groups of five animals each. Gr. 1 – irradiated rabbits treated with KLH; Gr.2 – irradiated rabbits treated with KLH + HRE; Gr.3 – non-irradiated rabbits treated with KLH; Gr. 4 – non-irradiated rabbits treated with KLH + HRE. Blood samples were taken from each rabbit by venipuncture of ear veins on Day 0 (before immunisation – non-immune sera), 12, 20 and 27 for primary antibody response, and on Day 43, 50, 58 (Day 7, 14 and 22 after reimmunisation, respectively) for secondary antibody response. Sera were collected after coagulation and centrifugation at 1800 rpm for 10 min and stored at −70°C.
ELISA for anti-KLH antibodies
The specific KLH IgG and total KLH-specific antibody production defined here as IgM + IgG + IgA was determined in sera, by homemade ELISA. The wells of Maxisorp flat-bottom immunomodules (Nunc, Roskilde, Denmark) were coated with 0.1 ml of 10 µg/ml solution of KLH (from Megatura crenulata; Sigma, USA) in 0.05 M sodium carbonate buffer, pH 9.6, and incubated overnight at 4°C. Then, the immunomodules were washed and blocked for 20 min with PBS containing 1% BSA. Next, the samples (0.1 ml per well) were diluted 1:50 in sample buffer (0.15 M PBS, pH 7.2 containing 1% BSA and 0.2% Tween) were added, and immunomodules were incubated for 1 h at room temperature. After three washes, the detecting antibodies (diluted 1:15000 in wash buffer, 0.1 ml per well) were added. For the detection of rabbit KLH-specific IgG, mouse monoclonal anti-rabbit IgG conjugated with peroxidase, γ-chain specific (Sigma, USA, product number A1949) was used. The total KLH-specific antibody production was revealed after the addition of mouse monoclonal anti-rabbit Ig, peroxidase-conjugated, recognising an epitope located on the heavy chain of rabbit IgG, IgM and IgA (Sigma, USA, product number A2074). After incubation of 1 h at room temperature, the immunomodules were washed, and 0.1 ml of substrate solution (containing 10 µl 35% H2O2 and 4 mg chromogene – o-phenylenediamine (OPD), dissolved to 20 ml 0.1 M citric acid buffer (pH 5.0), was added to each well. The subsequent colour reaction was stopped with 2M H2SO4, and the optical density was measured at 492 nm on an ELISA plate reader. All samples were duplicated. The results were expressed, as a percentage of mean OD492 value of non-immune sera.
Statistical analysis
The data are expressed as a mean ± SD. Student's t-test of statistical program Statistica 6 was used to determine the statistical differences between groups. The differences were considered significant when the p-value was less than 0.05.
Results
Primary total KLH-specific antibody production
During the primary immune response irradiated rabbits immunised with KLH showed significantly lower total KLH-specific antibody production than non-irradiated rabbits treated with KLH, p = 0.013 on Day 12 and p = 0.032 on Day 20 (, Columns 1 and 2). The results demonstrated that in the sera of irradiated rabbits treated with KLH + HRE, the total anti-KLH antibody production was increased compared to irradiated rabbits treated with KLH alone. This difference reached statistical significance on Day 12 (120% vs. 172%, p = 0.0008) and Day 20 (137% vs. 95%, p = 0.032). On Day 27, we did not find a significant difference between these groups.
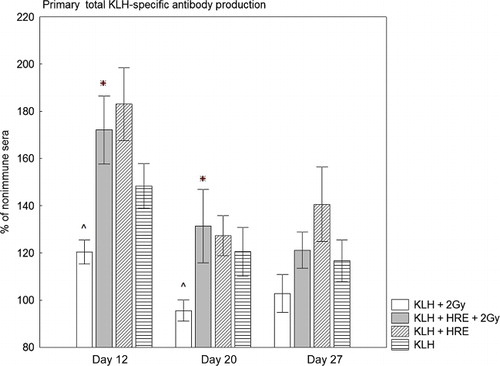
After primary immunisation, the total anti-KLH antibodies enhanced to 148% on Day 12, 120% on Day 20 and 116% on Day 27 (, Columns 3 and 4) in non-irradiated rabbits treated with KLH alone. The application of HRE increased the level of KLH-specific antibodies to 183% on Day 12, 127% on Day 20 and 140% on Day 27, but there were no significant differences between the groups.
Primary IgG KLH-specific antibody production
Results showed that on Day 12 irradiated rabbits immunised with KLH produced a significantly lower quantity of KLH-specific IgG antibodies than non-irradiated rabbits treated with KLH, p = 0.047 (120% vs. 154%) (, Columns 1 and 2). On Days 20 and 27 these two groups produced almost equal quantities of KLH-specific IgG antibodies. When irradiated rabbits were treated with HRE the anti-KLH IgG production increased from 134% to 178%, p = 0.049 on Day 12; from 139% to 176%, p = 0.026 on Day 20 and from 134% to 170%, p = 0.047 on day 27. Compared to non-irradiated rabbits treated with KLH, irradiated rabbits protected with HRE produced significantly higher anti-KLH IgG antibodies on Day 20 (139% vs. 176%, p = 0.05) and Day 27 (131% vs. 170%, p = 0.01).
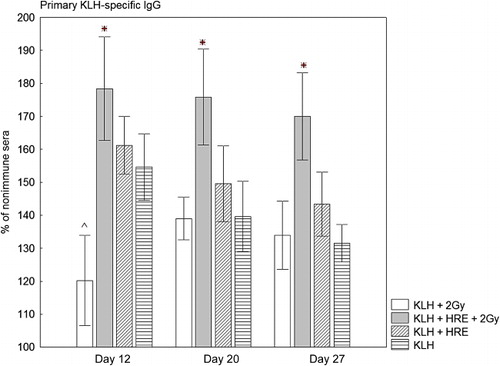
IgG anti-KLH antibodies in non-irradiated rabbits treated with KLH were raised to 154% on Day 12, 139% on Day 20 and 131% on Day 27 (, Columns 3 and 4). We found that the application of HRE enhanced KLH-specific IgG antibody production to 161% on Day 12, 149% on Day 20 and 143% on Day 27. However, we did not detect a significant difference between the groups.
Secondary total KLH-specific antibody production
The irradiated group of rabbits treated with KLH alone produced a lower quantity of total KLH-specific antibodies than the similarly treated non-irradiated group on Day 50 and Day 58, but there were no significant differences (, Columns 1 and 2). The results also demonstrated that in the irradiated group treated with HRE the level of total KLH-specific antibodies significantly rose in comparison with irradiated rabbits treated with KLH alone − 180% vs. 140%, p = 0.039 on Day 43; 180% vs. 115%, p = 0.000004 on Day 50; 147% vs. 97%, p = 0.000004 on Day 58. Moreover, irradiated rabbits treated with HRE produced significantly higher total KLH-specific antibodies than non-irradiated rabbits treated with KLH alone − 180% vs. 137%, p = 0.043 on Day 43, 185% vs. 126%, p = 0.001 on Day 50 and 147% vs. 108%, p = 0.006 on Day 58.
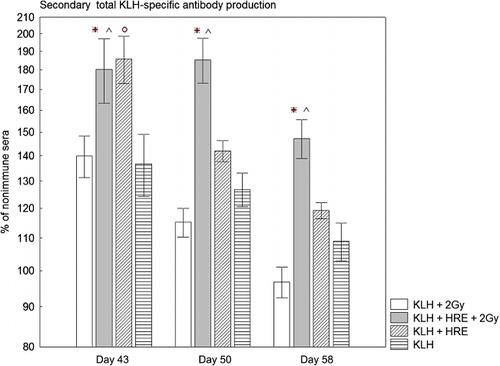
Seven days after the reimmunisation (Day 43) the non-irradiated group treated with KLH + HRE showed significantly higher total anti-KLH antibody production than the group treated with KLH alone (186% vs. 137%, p = 0.009) (, Columns 3 and 4). The total KLH-specific antibody production in the rabbits treated with HRE quickly decreased and on Day 50 and Day 58 we not find statistically significant enhancement.
Secondary IgG KLH-specific antibody production
On Day 43 the irradiated rabbits treated with KLH alone produced lower KLH-specific IgG, in comparison with the corresponding non-irradiated group (, Columns 1 and 2). On Day 50 and Day 58 we did not detect significant differences between these two groups. During the secondary antibody response, irradiated rabbits protected with HRE had a significantly higher KLH-specific IgG in their sera in comparison with the irradiated group treated with KLH alone. Protection with HRE increased KLH-specific IgG from 158% to 228%, p = 0.02 on Day 43; from 149% to 207%, p = 0.009 on Day 50 and from 142% to 184%, p = 0.04 on Day 58. Irradiated rabbits treated with HRE produced significantly higher KLH-specific IgG than non-irradiated rabbits treated with KLH alone − 206% vs. 149%, p = 0.008 on Day 50 and 184% vs. 122% on Day 58, p = 0.00004.
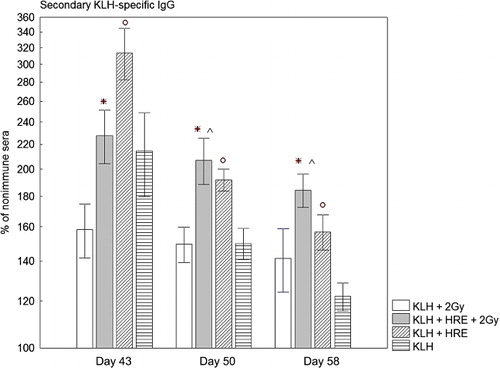
Reimmunisation increased KLH-specific IgG antibody production in non-irradiated rabbits treated with KLH alone to 214%, 149% and 122% on Day 43, 50 and 58 (, Columns 3 and 4). Treatment with HRE led to a significant enhancement of KLH-specific IgG antibody production of all three tested days compared to the group treated with KLH alone. The KLH-specific IgG rose to 314%, 191% and 157%, and p was 0.04, 0.015 and 0.008 at Day 43, 50 and 58, respectively.
Discussion
This study was designed to investigate the immunostimulatory properties of the H. rhodopensis extract on the primary and secondary antibody response. Enhanced specific antibody production is one of the basic biomarkers determining immunostimulatory activity of the tested agents.
Humoral immunity is mediated by the antibodies which are produced by plasma cells – terminally differentiated cells as a result of the activation and proliferation of antigen-specific B lymphocytes. The physiologic function of antibodies is to eliminate the antigens that induce their formation. Primary humoral immune responses leads mainly to IgM antibody production followed by IgG antibodies and memory B cell formation. Secondary response is characterised by memory B cell activation and rapid production of IgG antibodies, followed by IgA and IgE antibodies (Boes, Citation2000).
In the present study, we demonstrated that the application of the HRE at the beginning of the secondary response has a strong enhancing effect on KLH-specific IgG antibody production and a temporary short-term increasing effect on secondary total anti-KLH antibody production in non-irradiated rabbits. These facts allowed us to conclude that in normal physiological conditions, HRE has immunostimulatory properties strongly orientated to secondary humoral immune response, more exactly, secondary IgG antibody response. Secondary humoral immune response is initiated by the activation of antigen-specific memory B cells helped by cell–cell interaction with antigen-specific memory Th cells and secreted interleukins. This initial step is happening very quickly and is followed by the proliferation of activated memory B cells and its differentiation to antibody secreting plasma cells. From this perspective, several explanations are possible for the detected immunostimulatory activity of the HRE as follows: (1) HRE induces IFN-γ secretion thus enhancing IgG class switching and IgG memory B cells formation; (2) HRE may influence the process of activation of memory B cells by increasing the expression of MHC II and costimulatory molecules and thus may lead to enhancing antigen presentation; (3) HRE stimulates the release of cytokines from APC and Th cells (IL-2, IL-6 and IL-10) leading to increased proliferation of activated memory B cells.
Most plant-derived immunostimulators have the ability to activate APC (macrophages, dendritic cells and B cells) enhancing both antigen presentation and cytokine production. For example, Panax ginseng polysaccharide Ginsan profoundly enhanced the expression of co-stimulatory CD86 and non-significantly the expression of MHC Class II on the surface of dendritic cells (Kim et al., Citation2009). These polysaccharide modulated directly the cytokine production from DCs enhancing IL-12, IL-10 and TNF-alpha secretion. The mechanism of action of C3bgp, a well-defined immunostimulator on specific antibody production (Stanilova, Miteva, & Tanchev, Citation2005), involves the activation of macrophages/monocytes and the induction of specific cytokine production in vivo (in mice) and in vitro (Dobreva, Stanilova, & Miteva, Citation2008; Stanilova, Dobreva, Slavov, & Miteva, Citation2005; Stanilova, Dobreva, & Zhelev, Citation2001). In light of these data we suppose that HRE activated APC-inducing cytokines that affects the type and magnitude of the immune response.
Humoral immune response can be depressed by various external influences including emotional stress, physical stressors, chemical exposure, viral or bacterial infections, certain drug therapies, blood transfusions, surgery and radiation. In these cases, alternative therapies with immunostimulatory agents of plant origin may improve impaired immune response. An essential part of our study was answering of the question whether the extract possesses protective/stimulating properties on the specific antibody production in the context of immunosuppression induced by γ-irradiation.
Results obtained showed that initial γ-radiation exposure induced a well-defined immunosuppression on the primary total antibody production and a weaker one on the primary IgG antibody response. We observed that HRE application has a compensatory effect on the primary total KLH-specific antibody production because its application restored antibody production to the normal level which is detected in non-irradiated rabbits treated with KLH. Moreover, in the irradiated group treated with HRE primary KLH-specific IgG antibody synthesis was significantly increased compared to the normal level. It should be noted that in healthy, non-irradiated rabbits HRE did not show a well-defined immunostimulatory activity on the primary total and IgG antibody response. Briefly, we have found that the immunostimulatory activity of HRE regarding primary antibody response is more pronounced in irradiated rabbits than in non-irradiated rabbits. This may be explained by the inflammatory micro-environment created by radiation, accompanied by altered MHC and co-stimulatory molecule expression and secretion of soluble mediators like cytokines TNF-alpha, IFN-gamma and IL-10 (Friedman, Citation2002; Manda et al., Citation2012).
As is well known γ-radiation has a strong harmful effect on the activated and proliferating immune cells. Gamma-rays induced a significant depression of the lymphocyte count, blastogenesis and anti-SRBC antibody titers (Kjioka et al., Citation1999). Acute radiation syndrome occurs after a whole body irradiation of more than 1Gy. Higher radiation doses (>2 Gy) result in a massive killing of lymphocytes and even in a halting of the proliferation of haematopoietic progenitors (4). According to Kjioka et al. (Citation1999) B lymphocytes were the most radiosensitive and back to the normal levels on Day 15 after the irradiation of 3 Gy (Kjioka et al., Citation1999). Therefore, our results – increased specific antibody synthesis in groups with γ-irradiation-induced immunosuppression, and HRE application indicated that a plant extract has radioprotective properties regarding immune cells. Moreover, on the background of immunosuppression induced by γ-rays, HRE is a powerful immunostimulator of the primary specific antibody response as shown by the significant increase of the antibody levels in irradiated rabbits with HRE application compared to non-irradiated rabbits without HRE treatment.
Damage of DNA resulting from ionising radiation is the most important factor in cell death. Such damage is responsible for the decreased number of B cells in the spleen and lymphocyte progenitors in the bone marrow, for altered cell division of activated B cells and decreased number of antibody producing plasma cells. Previous studies showed that HRE confers protection against γ-radiation-induced DNA damage of rabbit's lymphocytes (Georgieva, Popov, & Bonev, Citation2013). The current study indicates that radioprotection by HRE restores humoral response and leads to normal or even to increased antibody levels.
A study has demonstrated the ability of free radical scavengers like antioxidants to protect immune cells against ionising radiation (Mishima et al., Citation2004). Plants and herbs are attractive sources of antioxidant compounds with radioprotective activity since they are complex mixtures of many chemicals (Jagetia, Citation2007; Shirazi et al., Citation2012). There was evidence that HRE contains a high level of flavonoid and other phenolic antioxidants. For example, several phenolic compounds were identified in the stems and leaves, mainly phenolic acids (ferulic acid, sinaric acid, caffeic acid, p-coumaric acid, etc.); flavonoid-aglycones and glycosides (luteolin, myricetin, hesperidin, rutin, etc.; Mihailova et al., Citation2011). These authors also suggest that the major antioxidant activity of H. rhodopensis was due to the phenolic compounds. The free radical scavenging and antioxidant properties of HRE have also been reported by Berkov et al. (Citation2011). Recently, new evidence showed that phenolic compounds isolated from H. rhodopensis possess significant cytoprotective, radical scavenging potential, and inhibit lipid peroxidation. Moreover, myconoside was a powerful natural antioxidant (Kondeva-Burdina et al., Citation2013). These properties of the compounds isolated from the herb suggested that H. rhodopensis extract may have radioprotective activities. For example, Birosova, Mikulasova, and Vaverkova (Citation2005) reported that the feluric and caffeic acids extracted from Echinacea have radioprotective activity and decreased the mutagenic effect of 5NFAA (Birosova et al., Citation2005). Several other studies also suggest that flavonoids may act as antioxidants, free radical scavengers and radioprotectors. Protection of DNA from oxidative stress by genestein, quercetin, luteolin and hesperidin is reported (Horvatova, Novothy, Tothova, & Vachalkova, Citation2004; Kalpana, Devipriya, Srinivasan, & Menon, Citation2009). Previous studies of our team relating to antioxidant activities of HRE showed decreased activity of antioxidant enzymes superoxide dismutase (SOD) and catalase (CAT) and an increased level of terminal metabolites of lipid peroxidation – malondialdehyde (MDA), in γ-irradiated rabbits. Treatment with HRE increased SOD and CAT activity and decreased MDA level (Georgieva et al., Citation2013). Polyphenols present in the plants and herbs may upregulate mRNAs of antioxidant enzymes such as CAT and SOD (Jagetia, Citation2007). Free radical-scavenging activities and upregulated activities of antioxidant enzymes, polyphenols and flavonoids, could explain its radioprotective activities regarding the specific antibody response observed in the current study.
A growing body of evidence shows that in addition to their radioprotective properties, flavonoids and other phenolic compounds possess strong immunostimulatory activity. Immunostimulatory activity expressed by increased lymphocyte activation and IFN-γ secretion by human peripheral blood mononuclear cells was reported for flavonoids and coumarins from vegetables and spices of Umbeliferae by Cherng, Chiang, and Chiang (Citation2008). Yu et al. using in vitro cell proliferation assay and cytotoxic assay detected immunomodulatory and anticancer activities of phenolics from Garcinia mangostana fruit pericarp (Yu, Zhao, Yang, & Bai, Citation2009). Stimulatory effects on splenocyte proliferation, on mouse splenocytes and cytotoxicities against human breast cancer cell line MCF-7 were evaluated by Zhao et al. (Citation2007) for flavonoids extracted from Litchi chinensis.
Our study is the first investigation on the immunomodulatory properties of H. rhodopensis, but the exact mechanisms by which HRE stimulates and protects the humoral immune response remains to be elucidated. However, our results and those of the above-mentioned authors, allow us to conclude that the main part of immunostimulatory and radioprotective properties of HRE are the result of the antioxidant properties of flavonoids and other polyphenols present in the extract.
In conclusion, this study demonstrated that the H. rhodopensis leaf extract has immunostimulatory and radioprotective properties regarding the specific antibody response. In the tested concentration HRE strongly augmented secondary KLH-specific IgG antibody response in healthy rabbits. HRE possesses radioprotective properties against γ-irradiation-induced immunosuppression and its application led to significant enhancement of the primary total and IgG KLH-specific antibodies. We hypothesise that the antioxidant activity of the constituents present in the extract is responsible for the immunostimulatory and radioprotective action of HRE.
References
- Arora, R., Gupta, D., Chawla, R., Sagar, R., Sharma, A., Kumar, R., … Sharma, R. K. (2005). Radioprotection by plant products: Present status and future prospects. Phytotherapy Research, 19(1), 1–22. doi:10.1002/ptr.1605
- Baliga, M. S., & Rao, S. (2010). Radioprotective potential of mint: A brief review. Journal of Cancer Research and Therapeutics, 6, 255–262. doi:10.4103/0973-1482.73336
- Berkov, S. H., Nikolova, M. T., Hristozova, N. I., Momekov, G. S., Ionkova, I., & Djilianov, D. (2011). GC-MS profiling of bioactive extracts from Haberlea rhodopensis: An endemic resurrection plant. Journal of the Serbian Chemical Society, 76, 211–220. doi:10.2298/JSC100324024B
- Birosova, L., Mikulasova, M., & Vaverkova, S. (2005). Antimutagenic effect of phenolic acids. Biomedical Papers of Medical Faculty University Palacky Olomouc Czech Repub, 149, 489–491. doi:10.5507/bp.2005.087
- Boes, M. (2000). Role of natural and immune IgM antibodies in immune responses. Molecular Immunology, 37, 1141–1149. doi:10.1016/S0161-5890(01)00025-6
- Cherng, J. M., Chiang, W., & Chiang, L. C. (2008). Immunomodulatory activities of common vegetables and spices of Umbelliferae and its related coumarins and flavonoids. Food Chemistry, 106, 944–950. doi:10.1016/j.foodchem.2007.07.005
- Dobreva, Z. G., Stanilova, S. A., & Miteva, L. D. (2008). Differences in the inducible gene expression and protein production of IL-12p40, IL-12p70 and IL-23: Involvement of p38 and JNK kinase pathways. Cytokine, 43, 76–82. doi:10.1016/j.cyto.2008.04.003
- Ebrahimi, S. N., Gafner, F., Dell'Acqua, G., Schweikert, K., & Hamburger, M. (2011). Flavone 8-C-glycosides from Haberlea rhodopensis Friv. (Gesneriaceae). Helvetica Chimica Acta, 94, 38–45. doi:10.1002/hlca.201000378
- Friedman, E. J. (2002). Immune modulation by ionizing radiation and its application for cancer immunotherapy. Current Pharmaceutical Design, 8, 1765–1780. doi:10.2174/1381612023394089
- Georgieva, S., Popov, B., & Bonev, G. (2013). Radioprotective effect of Haberlea rhodopensis (Friv.) leaf extract on gamma-radiation-induced DNA damage, lipid peroxidation and antioxidant levels in rabbit blood. Indian Journal of Experimental Biology, 51, 29–36.
- Horvatova, K., Novothy, L., Tothova, D., & Vachalkova, A. (2004). Determination of free radical scavenging activity of quercetin, rutin, luteolin and apigenin in H2O2-treated human ML cells K562. Neoplasma, 51, 395–399.
- Jagetia, G. C. (2007). Radioprotective potential of plants and herbs against the effects of ionizing radiation. Journal of Clinical Biochemistry and Nutrition, 40, 74–81. doi:10.3164/jcbn.40.74
- Jensen, S. (1996). Caffeoyl phenylethanoid glycosides in Sanango racemosum and in the gesneriaceae. Phytochemistry, 43, 777–783. doi:10.1016/0031-9422(96)00186-0
- Kalpana, K. B., Devipriya, N., Srinivasan, M., & Menon, V. P. (2009). Investigation of the radioprotective efficacy of hesperidin against gamma-radiation induced cellular damage in cultured human peripheral blood lymphocytes. Mutation Research/Genetic Toxicology Environmental Mutagenesis, 676, 54–61. doi:10.1016/j.mrgentox.2009.03.005
- Kim, M. N., Byon, Y. Y., Ko, E. J., Song, J. Y., Yun, Y. S., Shin, T., & Hong-Joo, H. G. (2009). Immunomodulatory activity of Ginsan, a polysaccharide of Panax Ginseng, on dendritic cells. The Korean Journal of Physiology & Pharmacology, 13, 169–173. doi:10.4196/kjpp.2009.13.3.169
- Kjioka, E. H., Gheorghe, C., Andres, M. L., Abell, G. A., Folz-Holbeck, J., Slater, J. M., … Gridley, D. S. (1999). Effects of proton and gamma radiation on lymphocyte populations and acute response to antigen. In Vivo, 13, 525–533.
- Kondeva-Burdina, M., Zheleva-Dimitrova, D., Nedialkov, P., Girreser U., & Mitcheva, M. (2013). Cytoprotective and antioxidant effects of phenolic compounds from Haberlea rhodopensis Friv. (Gesneriaceae). Pharmacognosy Magazine, 9, 294–301. doi:10.4103/0973-1296.117822
- Manda, K., Glasow, A., Paape, D., & Hildebrandt, G. (2012). Effect of ionizing radiation on the immune system with special emphasis on the interaction of dendritic and T cells. Frontiers in Oncology, 2, 102. 10.3389/fonc.2012.00102
- Mihailova, D., Bahchevanska, S., & Toneva, V. (2011). Microwave-assisted extraction of flavonoid antioxidants from leaves of Haberlea rhodopensis. Journal of International Scientific Publications: Materials, Methods & Technologies, 5, 104–114.
- Mishima, S., Saito, K., Maruyama, H., Inoue, M., Yamashita, T., Ishida, T., & Gu, Y. (2004). Antioxidant and immuno-enhancing effects of Echinacea purpurea. Biological and Pharmaceutical Bulletin, 27, 1004–1009. doi:10.1248/bpb.27.1004
- Müller, J., Sprenger, N., Bortlik, K., Boller, T., & Wiemken, A. (1997). Desiccation increases sucrose levels in Ramonda and Haberlea, two genera of resurrection plants in the Gesneriaceae. Physiologia Plantarum, 100, 153–158. doi:10.1111/j.1399-3054.1997.tb03466.x
- Popov, B., Radev, R., & Georgieva, S. (2010). In vitro incidence of chromosome aberrations in gamma-irradiated rabbit lymphocytes treated with Haberlea rhodopensis extract and vitamin C. Bulgarian Journal of Veterinary Medicine, 13, 148–153.
- Radev, R., Lazarova, G., Nedialkov, P., Sokolova, K., Rukanova, D., & Tsokeva, Z. H. (2009). Study of antibacterial activity of Haberlea rhodopensis. Trakia Journal of Science, 7, 34–36.
- Sgherri, C., Stevanovic, B., & Navari-Izzo, F. (2004). Role of phenolics in the antioxidative status of the resurrection plant Ramonda serbica during dehydration and rehydration. Physiologia Plantarum, 122, 478–485. doi:10.1111/j.1399-3054.2004.00428.x
- Sharififar, F., Pournourmohammadi, S., & Arabnejad, M. (2009). Immunomodulatory activity of aquaeous extract of Achillea wilhelmsii C. Koch in mice. Indian Journal of Experimental Biology, 47, 668–671.
- Shirazi, A., Mihandoost, E., Mahdavi, S. R., & Mohseni, M. (2012). Radio-protective role of antioxidant agents. Oncology Reviews, 6(2), 130–134. doi:10.4081/oncol.2012.e16
- Stanilova, S. A., Dobreva, Z. G., Slavov, E. S., & Miteva, L. D. (2005). C3 binding glycoprotein from Cuscuta europea induced different cytokine profiles from human PBMC compared to other plant and bacterial immunomodulators. International Immunopharmacology, 5, 723–734. doi:10.1016/j.intimp.2004.12.003
- Stanilova, S., Dobreva, Z., & Zhelev, Z. (2001). Changes in serum levels of cytokines in mice injected with an immunostimulator C3bgp isolated from Cuscuta europea. International Immunopharmacology, 1, 1597–1604. doi:10.1016/S1567-5769(01)00074-1
- Stanilova, S., Miteva, L. D., & Tanchev, S. G. (2005). Immunomodulatory effects of C3bgp on the antibody response to hemocyanin in outbred rabbits and F1 generation of breeding with siblings. Veterinary Immunology and Immunopathology, 106, 15–21. doi:10.1016/j.vetimm.2004.12.018
- Tzianabos, A. (2000). Polysaccharide immunomodulators as therapeutic agents: Structural aspects and biologic function. Clinical Microbiology Reviews, 13, 523–533. doi:10.1128/CMR.13.4.523-533.2000
- Yamini, K., & Gopal, V. (2010). Natural radioprotective agents against ionizing radiation – An overview. International Journal of PharmTech Research, 2, 1421–1426.
- Yu, L., Zhao, M., Yang, B., & Bai, W. (2009). Immunomodulatory and anticancer activities of phenolics from Garcinia mangostana fruit pericarp. Food Chemistry, 116, 969–973. doi:10.1016/j.foodchem.2009.03.064
- Zhao, M., Yang, B., Wang, J., Liu, Y., Yu, L., & Jiang, Y. (2007). Immunomodulatory and anticancer activities of flavonoids extracted from litchi (Litchi chinensis Sonn.) pericarp. International Immunopharmacology, 7, 162–166. doi:10.1016/j.intimp.2006.09.003