Abstract
F0F1-ATPase molecular motor biosensors were constructed by connecting specific probes to F0F1-ATPase’s ε-subunit through the avidin–biotin system. The test sample and negative sample were combined with the biosensors, respectively, and the DNA of the pathogenic bacteria can be tested by the comparison of the production of catalytically synthesized ATP after 10 min. The amount of synthesized ATP can be indirectly determined by the fluorescence intensity of luciferase–luciferin testing reagent. Through the comparison of the detection results of actual samples using three different methods, it was found that the method described in this study showed excellent agreement with the traditional detection method and polymerase chain reaction (PCR) detection method. The results also indicated that the F0F1-ATPase molecular motor biosensor could specifically detect bacterial DNA at a very low concentration level, and it would be a convenient, quick, and promising tool for pathogens detection.
Introduction
In recent years, food safety incidents happen frequently all over the world and the incidence rate of foodborne pathogens in animal-derived food is at an all-time high, so the detection of these pathogens has becoming the research focus worldwide (Punyokun, Hongprayoon, Srisapoome, & Sirinarumitr, Citation2013). Animal pathogenic bacterium, the source of the outbreak of related diseases, is one of the main factors causing food contamination. In recent decades, more and more research interest has been attracted to the detection of pathogenic bacterium (Feng et al., Citation2013; Nalbantsoy, Citation2013). All kinds of biochemical and molecular biology methods have been explored and gradually becoming the research focuses.
Among the numerous pathogenic bacterium, Cronobacter sakazakii and Shigella spp. are two common bacterium found in all kinds of foods. C. sakazakii is a kind of Gram-negative sporeless bacterium which is parasitic in the intestines of human and animals. C. sakazakii can cause not only severe neonatal meningitis, enterocolitis, and bacteremia, but also nervous system sequela and death. The infection mortality rate of this bacterium is as high as 50% (Pei & Liu, Citation2004). Although the host and propagation model of this bacterium have not been determined during the investigation of some of the neonatal C. sakazakii infection accidents, it was found that the infant formula was the main infection channel (Himelright et al., Citation2002; Van Acker et al., Citation2001). These potential or existing factors can undoubtedly cause problem to the health of the masses. The needless lose can be avoided if there is a method facilitating the quick detection and discovery of potential C. sakazakii in food. At the same time, Shigella spp. known as dysentery bacillus is a kind of highly contagious intestinal pathogenic bacteria. The Shigellosis, which spreads mainly through the digestive tract, affects the intestinal epithelial cells and gives rise to dysenteric symptoms with the typical symptoms of abdominal pain and diarrhea, and causes more severe harm to human health (Lin, Lai, & Zhang, Citation2009). According to statistics, 164.7 million people have been infected with malaria, causing 11,000 people to be dead. And most of the dead were children not more than five years old (Kotloff et al., Citation1999). With regard to the adult patients, 10–100 CFU of Shigella spp. is enough to cause pathopoiesia through intestinal infection and leads to a severe inflammatory response (Golovliiov, Sjostedt, Mokrievich, & Pavlov, Citation2003). According to China’s food safety law, Shigella spp. cannot be detected in food. However, the Shigellosis influences most area of our country in different extent and causes relatively large potential threat to people’s lives and health. Nowadays, many countries including China have adopted the traditional detection method to detect C. sakazakii and Shigella spp. However, this method suffers from the tedious and labor-consuming problems and it will take six days to obtain the detection report. Consequently, this has become a heavy burden to the inspection departments and cannot satisfy the requirement of rapid development of import and export trade (US Food and Drug Administration [FDA], Citation2002).
This study uses the F0F1-ATPase biosensor to detect C. sakazakii and Shigella spp. with high sensitivity while the detection time was dramatically reduced. F0F1-ATPase is a kind of rotary molecular motor. It can not only synthesize ATP through the transmembrane H+ gradient but also reversely transport H+ through hydrolysis of ATP. F0F1-ATPase generally exists in the mitochondrial membrane of animal, the thylakoid membranes of plant chloroplasts, and plasma membrane of bacteria. The main function of F0F1-ATPase is taking part in the oxidative phosphorylation or photophosphorylation, catalyzing the synthesis of ATP (Cui, Zhang, Yue, & Jiang, Citation2004). For example, the chromatophore adopted in this study exists in the plasma membrane of thermophilic bacteria. During the synthesis of ATP, H+ could be pumped from the inside to the outside of chromatophore membrane, causing variation to the H+ concentration of inner membrane microenvironment solution. The study found that the verticity of F0F1-ATPase could be influenced if a specific probe was attached to its ε-subunit, causing change to the synthesis rate of ATP (Wei et al., Citation2010; Zhang et al., Citation2005). Additionally, luciferase–luciferin luminous intensity has a linear relation with the concentration of ATP. Based on the two theories mentioned above, by immobilizing the target detection object (DNA) on ATP synthase and taking the fluorescence intensity of detection reagent (luciferase–luciferin) as single to reflect the load level, the goal of C. sakazakii and Shigella spp. detection was realized in this study.
Materials and methods
Chemicals and materials
C. sakazakii (ATCC 29544), Bacillus breslaviensis (ATCC 14028), Vibrio parahaemolyticus (ATCC 17802), V. cholerae (ATCC 14035), Escherichia coli (ATCC 25922) were purchased from ATCC (USA). Shigella spp. (CMCC 51630) was purchased from CMCC (China). Buffered peptone water (BPW), modified lauryl sulfate tryptone broth-vancomycin, tryptose soya agar, shigella enrichment broth-novobiocin BPW, Makanke agar, and Xylose lysine deoxycholate (XLD) agar were purchased from Beijing Luqiao Technology Co., Ltd. Biotin (Biotin-AC5-Sulfo-Osu) was obtained from Dojindo Company (Japan). Streptococcus antimicrobial protein (Streptavidin) was purchased from Sigma Company. The luciferase–luciferin was achieved from Promega Company, while the DNA isolation kit DP320 (Centrifugal type column) was purchased from Beijing Tiangen Company. The PBS buffer, synthesis buffer, and ultrapure water were self-made in our laboratory. All other reagents were at the analytically pure grade made in China.
Culture of microbes and extraction of DNA
C. sakazakii, Shigella spp., B. breslaviensis, V. parahaemolyticus, V. cholerae, and E. coli were first incubated in liquid medium, then inoculated on selective medium, and followed by inoculated on nutrient agar successively. The resulted bacteria were harvested to extract DNA.
DNA extraction was performed according to the instruction of DNA extraction kit (DP 320). The obtained DNA suspensions were stored at –20°C. The extracted DNA was used as the template of polymerase chain reaction (PCR) and detection target of F0F1-ATPase sensor.
The preparation of chromatophore
The chromatophore was prepared from the cells of thermophilic bacteria, Thermomicrobium roseum. The T. roseum strains were inoculated into liquid medium (the ratio was 1:100) and cultivated under 60°C for 24 h at 150 r/min, followed by 4000 r/min for 30 min, and centrifugal collection of thallus at 4°C. Then the thallus was resuspended in extraction buffer (20 mM Tris–HCl pH 8.0 containing 100 mM NaCl, 2 mM MgCl2, and 1 mM DTT) and harvested centrifugation at 6000 r/min under 4°C for 10 min. The thallus was suspended again in extraction buffer (10 mL of extraction buffer was added for each gram of thallus) and phenylmethanesulfonylfluoride was added into the system at a final concentration of 1 mM, followed by ultrasonic disruption on ice for 30 min (ultrasonication for 5 s and stop for 8 s). The disrupted thallus was centrifuged (6000 r/min, 30 min, 4°C) to remove the precipitate and take the supernatant. Then the supernatant was ultracentrifuged (40,000 r/min, 1 h, 4°C) and the precipitate was chromatophores. Finally, the chromatophore was suspended in extraction buffer with the addition of glycerol at a final concentration of 50% and stored in refrigerator under –80°C.
Synthesis of probes
According to the published sequence, the specific C. sakazakii gene ITS and the specific Shigella spp. gene IpaH were determined as target genes fragment for the synthesis of primers and probes while the synthesized probes’ 5′-position was labeled with biotin, as shown in . These probes were designed by using the LightCycler Probe Design Software 2.0 (Roche Diagnostics, Indianapolis, IN) and synthesized by Shanghai JIMA Pharmaceutical Technology Co., Ltd. Additionally, two primers for C. sakazakii, 5′-CCG GAA CAA GCT GAA AAT TGA-3′ and 5′-TCT TCG TGC TGC GAG TTT G-3′ with two other primers for Shigella spp. 5′-TCC GAT ACC GTC TCT GCA CGC AAT-3′ and 5′-AGC GAA AGA CTG TCG AAG CTC-3′ were synthesized for PCR verification. These primers were synthesized by Beijing Huada Genomics Co., Ltd.
Table 1. Specific probes for bacteria.
Construction of F0F1-ATPase molecular motor biosensor
The molecular motor biosensors were constructed following the method by Zhang et al. (Citation2012). N-biotin labeled ε-subunit antibody (40 μL) was added to an Eppendorf tube (1.5 mL) containing 200 μL of chromatophore. After being added up to 1 mL with PBS buffer, the mixture reacted under 37°C for 1 h. Then, the mixture was added up to 1.4 mL with PBS buffer and ultracentrifuged (4°C, 30,000 r/min, 10 min) to remove the supernatant. The precipitate was suspended in 500 μL of PBS buffer, followed by the addition of 2 μL of Streptococcus antimicrobial protein (2 mg/mL) as well as addition up to 1 mL with PBS buffer. The solution was placed on a shaker (50–100 r/min) to react for 10 min at room temperature. After reaction, each tube was added up to 1.4 mL and ultracentrifuged (4°C, 30,000 r/min, 10 min) to remove the supernatant. The precipitate was suspended in 500 μL of PBS buffer, followed by the addition of 50 μL of N-biotin labeled nucleic acid probe (ITS probe or IpaH probe; 10 μM) as well as addition up to 1 mL with PBS buffer. The solution was placed on a shaker (50–100 r/min) to react for 10 min at room temperature. After reaction, to each tube 0.4 mL of PBS buffer was added. Then, the product was in each tube was harvested with ultracentrifugation at 30,000 r/min under 4°C for 10 min and the supernatant was discarded. The precipitate was just the probe (Chro ITS or Chro IpaH), as shown in . The synthesized probe was then suspended in 150 μL PBS buffer containing 30% glycerol. Then, the tube containing the synthesized probe (Chro ITS or Chro IpaH) was stored in the refrigerator under –20°C with the information including preparation date, connection system, and producer labeled on the tube wall.
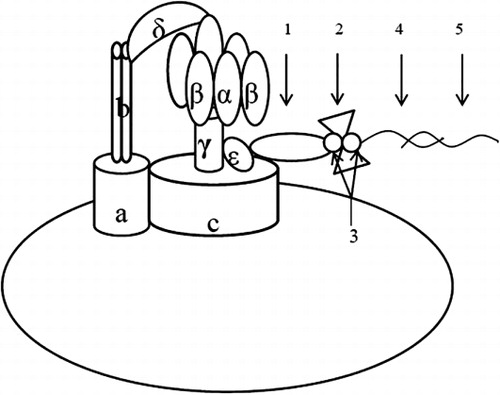
The construction of F0F1-ATPase molecular motor testing system
The F0F1-ATPase molecular motor testing system was constructed according to the method by Kaewphinit (Chen & Li, Citation2007; Liu, Zhang, Yue, Jiang, & Zhang, Citation2006). To a 1.5 mL Eppendorf tube (a), 10 μL of the DNA to be detected was added. Then the tube was placed in water bath at 95°C for 5 min, followed by in water bath at 50°C for 1 min. Then, diluted probe solution (2 μL of probe, Chro ITS or Chro IpaH, was diluted by certain times with synthesis buffer) was added. To another Eppendorf tube (b), 10 μL water was added, followed by the addition of 10 μL of synthesis buffer with blending. Then tube (b) was placed in water bath at 95°C for 5 min, followed by in water bath at 50°C for 1 min successively. The solution in tube (b) was used as background control.
Subsequently, 30 μL of starting buffer (prepared by mixing 1.3 M ADP and synthesis buffer at 1:3 ratio) was added to tube (a) and tube (b), respectively, with blending. The two tubes were centrifuged immediately to let the drops on the tube wall to fall down the bottom of the tube. The two tubes were incubated in a shaker for 10 min at 37°C to start the reaction. After reaction, the tubes were taken out of the shaker and 200 μL of PBS buffer was added into the two tubes, respectively, with blending. The reaction solution in the two tubes was dropped into a clean 96-well plate, three orifices for each reaction solution, and 50 μL for each orifice. Then, to each of the orifices containing reaction solution, 30 μL of luciferase solution was added with pipetting repeatedly for several times. Finally, the 96-well plate was placed into Chemiluminescence detector for detection. The data were recorded and the date in each group was averaged. The actual fluorescence values of samples were obtained by the values of samples minus those of background.
Results and discussions
The specificity of the probes
In this study, Chro ITS was adopted to detect different pathogenic bacterium and the detection of C. sakazakii was realized specifically. In this experiment, the DNA of C. sakazakii, Salmonella typhimurium, V. parahemolyticus, V. cholerae, and E. coli were detected by Chro ITS. The initial concentration of Chro ITS was determined to be 1.558 mg/mL using BCA protein assay kit. Through the concentration gradient experiment, the testing conditions were determined to be that the concentration of Chro ITS was 0.019 mg/mL and the DNA concentration was 40 ng/mL. The testing results were shown in . As shown in , the fluorescence detection value of C. sakazakii was obviously higher than those of other subjects indicating that the molecular motor showed excellent specificity toward C. sakazakii and could discriminate different subgenus to achieve the expected detection effect.
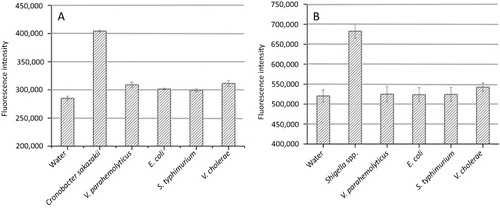
At the same time, Chro IpaH was adopted to discriminate different pathogenic bacterium too, realizing the detection of Shigella spp. specifically (IpaH is carried by all four Shigella species). In this experiment, the DNA of Shigella spp., B. breslaviensis, V. parahaemolyticus, V. cholerae, E. coli, and C. sakazakii were detected by Chro IpaH. The initial concentration of Chro IpaH was determined to be 1.558 mg/mL. In this study, the experiment was started for 10 min under the conditions that the concentration of Chro IpaH was 0.031 mg/mL and the DNA concentration was 50 ng/mL at 37°C. The fluorescence values of the final reaction system were then recorded. As shown in , Chro IpaH demonstrated excellent specificity toward Shigella, being able to discriminate different species.
The testing results comparison among F0F1-ATPase molecular motor testing technology, traditional testing method, and PCR method
Comparison was made among the F0F1-ATPase biosensor technology, traditional testing method, and PCR detection method. Two strains of C. sakazakii obtained under the experiment conditions described in the specificity of the probes section were tested and were determined to be positive strains. The ITS gene of the two strains was amplified and the electrophoresis results were shown in . According to the method described in National Standard, GB 4789.40-2010, the two standard bacteria strains underwent chromogenic culture and biochemical identification and were identified to be C. sakazakii. The comparison among the results of the three methods was listed in . It could be seen that the three methods could detect C. sakazakii with excellent consistency demonstrating the feasibility of detection of C. sakazakii with F0F1-ATPase biosensor technology as well as good detection effect.
Table 2. Result comparison of different detection methods.
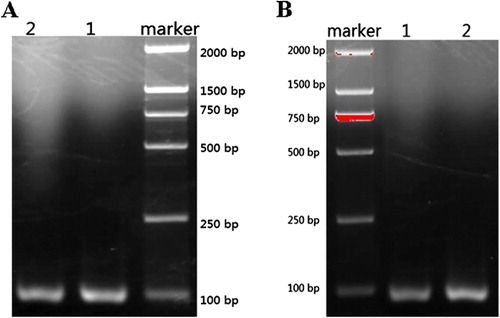
Before the comparison was made, two key variables, the concentration of the Chro IpaH and the DNA concentration of Shigella spp., were optimized and the optimal concentrations for the two variables were 0.031 mg/mL for Chro IpaH and 50 ng/mL for the DNA of Shigella spp. So, when the comparison was made, the concentration of Chro IpaH and the DNA of Shigella spp. were determined to be 0.031 mg/mL and 50 ng/mL, respectively. Under the optimal conditions, two standard bacteria strains were inspected and the results showed that they were positive. demonstrated the electrophoresis results of the IpaH gene of the two standard bacteria strains after being amplified by PCR method. listed the testing results of three methods toward the same target bacteria strains and the comparison indicated that F0F1-ATPase molecular motor method showed excellent testing effect.
Conclusion
This study showed that using F0F1-ATPase biosensor technology for the detection of C. sakazakii and Shigella spp., the testing sensitivity was higher than that for the traditional methods, being able to meet the testing requirements of inspection and quarantine departments for the detection of C. sakazakii and Shigella spp. Many steps, such as strain accumulation and selective culture which were indispensable in traditional biochemical identification test method and PCR method, could be avoided using this method for the detection of C. sakazakii and Shigella spp. in food, so the testing periodic time could be reduced from original six days to two days. This reduction of testing time could extend the preservation and shelf life of animal source food, facilitating the improvement of food import and export trade. Through the comparison of the detection results of two standard bacteria strains using detection method and traditional method, PCR method and F0F1-ATPase biosensor method, respectively, it was found that the consistency of the results was 100%.
Due to the adoption of liquid phase method in this study, the rotating speed of liquid phase reaction system will be influenced when the sample was not pure or containing impurities. Further, the results judgment would be affected. For this reason, we envisaged that the F0F1-ATPase biosensor could be immobilized on the surface of membrane and impurities could be filtered out through feeding buffer, reducing the interference to the experiment (Liu et al., Citation2006). F0F1-ATPase biosensor possessed the virtues of excellent specificity, high sensitivity, and short testing periodic time. It not only showed extensive application value in the areas of clinical diagnosis, the treatment of cardiovascular and cerebrovascular diseases, industrial control, environmental conservation, and biotechnology, but also has further extensive application prospects in the fields of rapid and high-throughput detection and typing of foodborne pathogenic bacterium and virus in Entry–Exit food of China.
Acknowledgment
We are grateful to Prof Jiachang Yue, Institute of Biophysics, Chinese Academy of Sciences, for his help and valuable suggestions.
Disclosure statement
No potential conflict of interest was reported by the authors.
References
- Chen, C. S., & Li, X. J. (2007). Application of a biosensor for super-sensitive detector of clenbuterol. New Zealand Journal of Agricultural Research, 5, 689–695.
- Cui, Y. B., Zhang, Y. H., Yue, J. C., & Jiang, P. D. (2004). Direct observation of the clockwise light-driven rotation of F0F1-ATP synthase complex. Chinese Science Bulletin, 49, 1342–1347. doi:10.1360/04wc0009
- Feng, M., Yong, Q., Wang, W., Kuang, H., Wang, L., & Xu, C. (2013). Development of a monoclonal antibody-based ELISA to detect Escherichia coli O157:h77. Food and Agricultural Immunology, 24, 481–487. doi:10.1080/09540105.2012.716026
- Golovliiov, I., Sjostedt, A., Mokrievich, A., & Pavlov, V. (2003). A method for allelic replacement in Francisella tularensis. FEMS Microbiology Letters, 222, 273–280. doi:10.1016/S0378-1097(03)00313-6
- Himelright, I., Harris, E., Lorch, V., Anderson, M., Jones, T., Craig, A., … Jernigan, D. (2002). Cronobacter sakazakii infections associated with the use of powdered infant formula—Tennessee, 2001. Morbidity and Mortality Weekly Report, 14, 298–300.
- Kotloff, K. L., Winickoff, J. P., Ivanoff, B., Clemens, J. D., Swerdlow, D. L., Sansonetti, P. J., … Levine, M. M. (1999). Global burden of Shigella infections: Implications for vaccine development and implementation of control strategies. Bull World Health Organ, 8, 651–656.
- Lin, X. L., Lai, W. H., & Zhang, L. L. (2009). Recent advances in detection of Shigella Species in food. Food Science, 15, 271–275.
- Liu, X. L., Zhang, Y., Yue, J. C., Jiang, P., & Zhang, Z. (2006). F0F1-ATPase as biosensor to detect single virus. Biochemical and Biophysical Research Communications, 342, 1319–1322. doi:10.1016/j.bbrc.2006.02.103
- Nalbantsoy, A. (2013). Prevention and identification of Salmonella Enteritidis infection via novel diagnostic stained antigens and polyclonal antibodies. Food and Agricultural Immunology, 24(2), 139–149. doi:10.1080/09540105.2012.662629
- Pei, X. Y., & Liu, X. M. (2004). Biological characteristics and health hazards of Enterobacter sakazakii: A review. Chinese Journal of Food Hygiene, 6, 550–555.
- Punyokun, K., Hongprayoon, R., Srisapoome, P., & Sirinarumitr, T. (2013). The production of anti-Vibrio harveyi egg yolk immunoglobulin and evaluation of its stability and neutralisation efficacy. Food and Agricultural Immunology, 24, 279–294. doi:10.1080/09540105.2012.684203
- US Food and Drug Administration (FDA). (2002). Isolation and enumeration of Enterobacter sakazakii from dehydrated powdered infant formula. http://www.fda.gov/Food/ScienceResearch/LaboratoryMethods/ucm114665.htm.
- Van Acker, J., de Smet, F., Muyldermans, G., Bougatef, A., Naessens, A., & Lauwers, S. (2001). Outbreak of necrotizing enterocolitis associated with Enterobacter sakazakii in powdered milk formula. Journal of Clinical Microbiology, 39, 293–297. doi:10.1128/JCM.39.1.293-297.2001
- Wei, L., Wu, H. J., Lun, Y. Z., Li, B. M., Gao, L. J., Zhang, X. L., & Kang, Z. J. (2010). An immunobiosensor for rapid detection of Staphylococcus aureusenes. Chinese Journal of Food Hygiene, 6, 498–501.
- Zhang, J., Gu, D. Z., Zhang, H. Y., Yang, Z. H., Wang, P. R., Zhang, L., … Yue, J. (2012). Preliminary study of a rapid detecting technology for Salmonella based on molecular motor biosensor. Science and Technology of Food Industry, 12, 93–96.
- Zhang, Y. H., Wang, J., Cui, Y. B., Yue, J. C., & Fang, X. H. (2005). Rotary torque produced by proton motive force in F0F1 motor. Biochemical and Biophysical Research Communications, 1, 370–374.