ABSTRACT
The carbodiimide active ester method was employed to synthesise the antigen of 1-cyclopropyl-7-(4-ethylpiperazin-1-yl)-6-fluoro-4-oxo-1,4-dihydroquinoline-3-carboxylic acid [Enrofloxacin (ENFX)], and male Balb/c mice were used to produce anti-ENFX monoclonal antibody. Based on the checkerboard titration, an indirect competitive enzyme-linked immunosorbent assay standard curve was established. This assay was sensitive and highly specific to ENFX with the half maximal inhibitory concentration and limit of detection values of 0.15 ng mL−1 and 0.028ng mL−1, respectively. Similarly, the antibody had a high affinity as seen in Amax values. Spiked cow milk beef liver and fish samples analysed using this kit had recoveries in the range of 90.2–110.2%, 70.7–81.5% and 89.1–101%, respectively, showing satisfactory results. The results suggest that this ELISA kit could be applied as a screening method to detect and control the illegal content of ENFX in food products.
Introduction
Fluoroquinolones (FQs) are a group of potent antibiotics which are extensively used for both the prevention and treatment of various diseases in animal husbandry as well as in humans (Huet et al., Citation2006). FQs are derived from the quinolone 1-ethyl-7-methyl-4-oxo-[1,8]naphthyridine-3-carboxylic acid (nalidixic acid – the first of the synthetic quinolone antibiotics) which has a fluorine atom attached to the central ring system, a piperazine moiety at position 7 and a fluorine atom at position 6 (Peterson, Citation2001). An example is Enrofloxacin (ENFX), , which is typical of FQs that have a wide spectrum of action and high efficacy against infectious disease particularly those caused by gram-positive, gram-negative and Mycoplasma bacteria including Pseudomonas aeruginosa, Klebsiella, Escherichia coli, Enterobacter, Campylobacter, Shigella, Salmonella and Mycobacterium, among others (Hooper, Citation2001).
Figure 1. Chemical structure of FQs. (a) Typical generic structure of the quinolone molecule, using the accepted numbering scheme for positions on the molecule. R indicates possible sites for structural modification. Molecules at positions marked by a dashed box can also be changed. (b) The ENFX structure, the quinolone used as a hapten in this study.
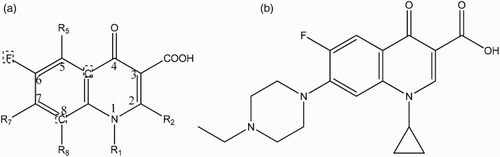
Veterinary antibiotics are used in agriculture to prevent disease and promote growth. ENFX in particular is used in the treatment of bovine respiratory disease associated with Mannheimia haemolytica, Pasteurella multocida and Histophilus somni in beef and non-lactating dairy cattle and control of swine respiratory disease associated with Actinobacillus pleuropneumoniae, P. multocida, Haemophilus parasuis, Streptococcus suis, Bordetella bronchiseptica and Mycoplasma hyopneumoniae. It used to be administered through drinking water for poultry treatment until September 2005 when it was banned by the US Food and Drug Administration (FDA) following reports of the evolution of FQ-resistant strains of the bacterium Campylobacter, a human pathogen (FDA, Citation2005). When used for treatment of lactating animals, sufficient withdrawal periods are required before milk can be consumed by humans. Misuse of these drugs, particularly in developing countries, can lead to their persistence in animal tissue and milk, where they can pose a health hazard due to the possibility of allergic reactions by hypersensitive individuals (Hooper, Citation2001; Paige, Tollefson, & Miller, Citation1999). Other dangers associated with these antibiotic residues involve the stimulation of drug-resistant pathogenic bacteria strains. Studies have reported an increased rate of pathogens resistant to FQs in the Middle East, corresponding to widespread misuse of the antibiotic in food-producing animals (Al-Mustafa and Al-Ghamdi, Citation2000). Evidence for the presence of multiple drug-resistant strains of Campylobacter, Staphylococcus and E. coli has also been reported (Randall et al., Citation2003). There is overwhelming evidence of the transfer of these drug-resistant strains of bacteria from animals to humans (Butaye, Devriese, & Haesebrouck, Citation2001; Drlica, Zhao, Malik, Salz, & Kerns, Citation2012; Rice, Citation2012) and this trend is expected to increase (Cabello et al., Citation2013). In addition, these antimicrobial residues in foods of animal origin may have adverse effects on the indigenous human intestinal micro-flora which are an essential component of human physiology. These flora act as a barrier against colonisation of the gastrointestinal tract by pathogenic bacteria (Cerniglia and Kotarski, Citation1999) and have an important role in the digestion of food. Furthermore, studies have shown that food-processing methods such as cooking and pasteurisation do not affect the level of FQs in food since residues remain relatively stable during heating (Al-Mustafa and Al-Ghamdi, Citation2000; Lolo et al., Citation2006; Roca, Castillo, Marti, Althaus, & Molina, Citation2010). As a result of these concerns, many countries and organisations including the European Union (EU), USA, Japan, People's Republic of China (PRC), and Food and Agriculture Organization of the United Nations (FAO) have set maximum residue limits (MRLs) for several quinolones and FQs (Huang et al., Citation2010). For example, among the EU members, an MRL of 100 µg kg−1 has been recommended for bovine muscle, fat and milk, 200 µg kg−1 and 300 µg kg−1 for bovine kidney and liver, respectively (European Commission, Citation2010) for ENFX. In PRC, the species of animal, usage, dosage and withdrawal period of FQs have been established by the Ministry of Agriculture of the PRC (No. 278, 22 May 2003). In view of all these circumstances, it is crucial that foods of animal origin be monitored for the presence of veterinary drug residues to safeguard public health, prevent their illicit use and facilitate regulation and surveillance (Chen and Jiang, Citation2013; Wang, Zhang, Ni, Zhang, & Shen, Citation2014). Currently, there is also a growing demand for easy, rapid and economical methods to monitor FQ residues in foodstuffs.
Instrumental techniques, such as high-performance liquid chromatography (HPLC) (Freitas, Barbosa, & Ramos, Citation2012; Jin-Lan, Chang, & Yue, Citation2012) and liquid chromatography-tandem mass spectrometry (LC-MS/MS), have been employed in the determination of ENFX residues in biological matrices (Cerniglia and Kotarski, Citation1999; Freitas et al., Citation2012; Jin-Lan et al., Citation2012; Lopes et al., Citation2012). While these methods provide sensitive and accurate techniques, they rely on a highly trained personnel to operate expensive and sophisticated instruments and interpret complicated chromatograms or spectral results. Moreover, these procedures are laborious and employ the use of harmful organic solvents that are hazardous to personnel and pollute the environment. Hence, they may be unsuitable for on-site screening for a large amount of samples where speed and simplicity may be required.
Enzyme-linked immunosorbent assays (ELISAs) are analytical methods based on specific antibody/antigen interaction. These methods have advantages of being less labour intensive, high sensitivity, portable with high throughput, simple sample preparation and low cost, and hence have become popular and are considered alternative or complementary to conventional instrumental techniques (Jiang et al., Citation2013; Jinqing et al., Citation2012; Mi, Wang, Eremin, Shen, & Zhang, Citation2013; Watanabe, Satake, Kido, & Tsuji, Citation2002).
Several authors have published work on ELISA methods for the detection of ENFX (Bucknall, Silverlight, Coldham, Thorne, & Jackman, Citation2003; Huet et al., Citation2006; Li et al., Citation2008; Ni et al., Citation2014; Suryoprabowo, Liu, Peng, Kuang, & Xu, Citation2014). However, most of this published work was based on polyclonal antibodies and where monoclonal antibodies (mAbs) were utilised, the level of detection was not sensitive enough to capture trace levels of the ENFX residues in food products. This is summarised in .
Table 1. A summary of cross-reactivity for ENFX and different parent haptens as reported by various authors.
In this study, our aim was to produce a mAb against ENFX and develop a highly sensitive, indirect competitive ELISA (icELISA) for the detection of ENFX residue in milk, fish and beef liver. The influence of several physicochemical factors such as ionic strength, pH value and casein concentration was carefully evaluated for their effects on the icELISA performance.
Materials and methods
Chemicals and materials
ENFX and other FQ standards were purchased from Shanghai HOPE Industry Co., Ltd (Shanghai, PRC). Freund's complete adjuvant (FCA), Freund's incomplete adjuvant (FIA) and enzyme immunoassay-grade horseradish peroxidase-labelled goat anti-mouse immunoglobulin (HRP-IgG) were obtained from Jackson Immuno-Research Laboratories, Inc. (West Baltimore Pike West Grove, PA, USA). 1-Ethyl-3-(3-dimethylaminopropyl) carbodiimide (EDC) and N-hydroxysuccinimide (NHS) were obtained from Pierce (USA). Gelatine was obtained from Beijing Biodee Biotechnology Co., Ltd (Beijing, PRC). 3,3′,5,5′-Tetramethylbenzidine (TMB) was purchased from Aladdin Chemistry Co., Ltd (Shanghai, PRC). All reagents for cell fusion were sourced from Sunshine Biotechnology Co., Ltd (Nanjing, PRC). Bovine serum albumin (BSA) and Chicken white ovalbumin (OVA) were obtained from Solarbio Science & Technology, Co., Ltd (Beijing, PRC). Other reagents and chemicals were obtained from the National Pharmaceutical Group Chemical Reagent Co., Ltd (Shanghai, PRC).
Solutions and buffers
Most buffers used were prepared based on 0.01 M phosphate-buffered saline (PBS, pH 7.4) containing per litre KCl (0.2 g), KH2PO4 (0.24 g), NaCl (8.0 g) and Na2HPO4·12H2O (3.62 g). The blocking buffer contained 0.2% w/v gelatine in coating buffer (described below), washing buffer contained 0.05% v/v Tween 20 in PBS (PBST) and the antibody dilution buffer was basically PBS containing 0.1% w/v gelatine and 0.05% v/v Tween 20. The coating buffer (pH 9.6) contained Na2CO3 (1.59 g) and NaHCO3 (2.93 g) per litre water. The ELISA substrate reagent freshly prepared was a mixer at a ratio of 1:5 (v/v) 0.6 mg mL−1TMB in glycol and 0.1 M citrate phosphate buffer (pH 5.0) containing 180 µL L−1 of 30% hydrogen peroxide. The ELISA stop reagent was 2 M sulphuric acid.
Standard stock solutions (100 µg mL−1) of antibiotics were prepared by dissolving an appropriate amount of each standard in 0.03 M sodium hydroxide. The individual stock solutions were stored at 4°C in amber glass bottles and were stable for at least three months. Working standards (0.01, 0.03, 0.09, 0.27, 0.9, 3, 9, 27, 81 and 243 ng mL−1) of each antibiotic were prepared and used immediately by diluting the stock solution in assay buffer.
Immunogen and antigen synthesis
The immunogen ENFX–BSA and the coating antigen ENFX–OVA were both prepared by the carbodiimide active ester method (Huang et al., Citation2010). A total of 9.12 mg (0.03 mmol) ENFX, 9.53 mg (0.06 mmol) of EDC and 3.21 mg (0.03 mmol) of NHS were added to 1.0 mL of dry dimethylformamide in order. The mixture solution (1) was incubated for 24 h at 37°C in the dark while being stirred using a magnetic stirrer.
This solution (1) was drop-wise added to 3.0 mL of PBS (0.01 mol/L, pH 7.4) containing 16.75 mg BSA (solution 2) at room temperature for 1 h, followed by 3 h incubation at 37°C in the dark, while stirring with a magnetic stirrer. This was followed by a resting period overnight at 4°C.
Finally, the reaction mixture was dialysed under stirring against milli-Q deionised water for 2 days followed by PBS (0.01 mol/L, pH 7.4) for a further 2 days with 6 h repeated changes of the dialysis solution to remove the unconjugated free hapten.
The UV absorbance method was used to determine whether or not the conjugate had success as shown in . The obtained ENFX–BSA conjugate was stored at −20°C. The ENFX–OVA conjugate was prepared in a similar manner.
Figure 2. Antigen characterisation with 1 and 2 showing the typical BSA and OVA peaks at a maximum of 278 nm, 3 shows the typical ENFX peaks with prominent absorbing at 271 nm, and the minor ones at 322 and 335 nm, 4 shows a compromise peak of ENFX–OVA taking its shape and absorption (278, 322 and 335 nm) after both ENFX and OVA, while 5 is a compromise of ENFX with BSA showing a clear shift in ENFX absorption.
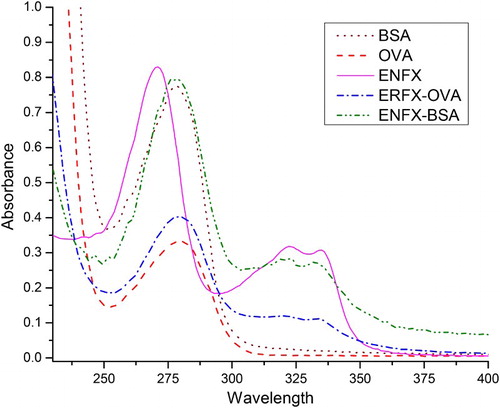
Antibody production and characterisation
Immunogens diluted by sterile 0.9% saline (NaCl injection solution) were emulsified with an equal volume of either FCA for the first immunisation or FIA for subsequent boost immunisations. This mixture was repeatedly made to pass through a 1 mm bored tunnel in a 2 cm long cylindrical Pyrex block, drilled at each end to accommodate two syringes with the aid of an emulsifier (Model No. SUNON DP201AT – Sunon Wealth Elec Mach Ind. Co., Ltd, PRC) at a rate of four strokes per second with intermitted rest periods after every seven minutes until it was ready to use. Once ready, the immunogens were administered at three-week intervals. Following this protocol, a 100 µg dose was subcutaneously given to male Balb/c mice (6–8 weeks old) in the initial immunisation and thereafter 50 µg for the subsequent boost immunisations all done at multiple sites on the animal. Seven days following the third boost, the mice were tail bled and blood samples collected and were subjected to indirect ELISA.
Cross-reactivity determination
Stock solutions of different FQs were prepared as described in the solutions and buffers section. Standard curves were prepared in the assay buffer, and each half maximal inhibitory concentration (IC50) value was determined in the competitive experiment. The cross-reactivity values were calculated according to the following equation:
ELISA development
Competitive ELISA and antibody titre determination
The microtitre plates were coated with 100 µL/well of a coating antigen (ENFX–OVA) at different concentrations (1.0, 0.3, 0.1 and 0.03 µg mL−1) in the carbonate coating buffer followed by overnight incubation at 4°C. The following day, the plates were washed three times with washing buffer, and sealed with blocking buffer (210 µL/well) followed by incubation at 37°C for 2 h. After washing twice, the antiserum at four different dilutions (1:300–1:81,000) were added to the wells (50 µL/well) at two levels; level one PBS with zero drug standards and level two chosen drug standard prepared in PBS, respectively, and incubated for 30 min at 37°C. Plates were washed thrice and a diluted (1:3000) solution of goat anti-mouse IgG-HRP was added (100 µL/well) and incubated for 30 min at 37°C. This was followed by washing the plates four times and 100 µL/well of freshly prepared TMB solution was added and incubated for a final 15 min at 37°C before the reaction was stopped by adding 2 M sulphuric acid (50 µL/well). We note here that all incubations were done in dark conditions. Finally, absorbance was read at 450 nm with the aid of a microplate reader (Model EON-Biotek Instruments Inc., Highland Park, USA).
Following the results, the mouse whose serum showed the highest titre was sacrificed and its splenocytes were fused with SP 2/0 murine myeloma cells (Peng et al., Citation2012); thereafter, hybridomas were screened using an indirect competitive ELISA. Two hybridoma cell lines (designated 2E9 and 2G4) selected were cloned and subcloned thrice by limiting dilution before being injected into Balb/c mice to produce the mAb to ENFX (Deng et al., Citation2011). Ascites were harvested and purified using the caprylic acid-ammonium sulphate precipitation method (Kuang et al., Citation2013; Perosa, Carbone, Ferrone, & Dammacco, Citation1990; Temponi et al., Citation1989). The purified mAb solutions (named mAb2E9 and mAb2G4 according to their respective hybridoma cell lines) were divided into small aliquots and stored at −20°C for further analysis in this study.
Standard calibration curve. A standard calibration curve with ENFX concentrations between 0.01 and 2.7 ng mL−1 was established under optimised conditions. The limit of detection (LOD) or the least detectable dose was evaluated as the concentration of ENFX giving a 10% inhibition (Sun, Liu, Kuang, & Xu, Citation2011).
Evaluation of ELISA
Sample preparation and detection
Milk, beef liver and fish were purchased from a local supermarket and used for recovery tests. The residue-free status of the samples was confirmed by HPLC–MS/MS (Standard, Citation2009) prior to use.
Milk analysis
Each milk aliquot (5 mL) was diluted with assay buffer to 250 times its original volume for ELISA analysis. Precision detection was determined by spiking a pool of negative milk samples with ENFX at three concentrations (50, 100 and 200 µg L−1). Intra-assay variability in this study is defined as evaluation of 15 repeat samples analysed on a single day, while inter-assay variability was determined from triplicate spiked samples analysed on six different days. Accuracy means the recovery data of the spiked concentrations and precision was expressed as the coefficient of variation (CV).
Fish and beef liver extract preparation
Beef liver samples were sliced into small pieces and blended before aliquot of 1.0 ± 0.05 g was weighed into a 50 mL disposable polypropylene centrifuge tube for each analysis. Centrifugal force at 3000 rpm at 4°C for 5 min was applied to move the sample from the inside tube wall to the bottom of the tubes. Fortification was done at 50, 100 and 200 µg kg−1 levels before the samples were subjected to a 30 min vortex at 3000 vibrations per minute in a Vibrax mixer (IKA MS 3 digital). This was followed by 30 min incubation at 25°C in the dark. Five millilitres of 0.01 M PB (pH 7.4) were added to each tube, the contents homogenised for 20 s at 5000 rpm (Fluko Model F6/10) and then centrifuged at 8500 rpm at 4°C for 10 min. Approximately 2500 μL of 0.01 M PB (pH 7.4) was added to the pellet after collecting the supernatant and re-extraction done like before. The pooled supernatants were vortexed for 20 s and filtered through a 0.2 μm nylon syringe filter into an autosampler vial and stored at 4°C for analysis the day after. Fish samples were similarly prepared.
Curve fitting and statistical analysis
A four-parameter logistic equation was used to fit the immunoassay data. Calculations were performed using OrginPro 8.5 software (OriginLab Corporation, Northampton, MA, USA).
Results and discussion
Antigen characterisation
The ENFX molecule in the UV absorption spectrum () showed typical absorbance peaks at 271, 322 and 335 nm. The peak at 271 nm was very prominent as compared to the peaks seen at 322 and 335 nm. The proteins BSA and OVA had a characteristic peak with a maximum absorbance observed at 278 nm. The conjugates of ENFX–BSA and ENFX–OVA were found to show a typical absorbance peak at 280, 323 and 332 nm, a representation of the peaks from ENFX–BSA and ENFX–OVA interaction, respectively. We noted that while the minor peaks of ENFX showed at 322 and 335 nm, in both conjugates, there was a shift in the right-most peak by 3 nm units towards the left which brought the two peaks closer to each other with a separation of 9 nm units. However, there was a significant shift in the major peak by 9 nm units from 271 to 280 nm. ENFX (MW 359.399) being non-immunogenic, conjugation with carrier protein BSA is a prerequisite to the production of an activated B-cell clone. The conjugates ENFX–BSA and ENFX–OVA were used as immunogen and coating antigen, respectively, in this study. After the fifth immunisation cycle, a mouse producing high titre antibody was selected for cell fusion. Antibody from selected hybridoma was characterised with high titre (1:8100).
Optimisation of ELISA
Using the checkerboard titration method, it was determined that the optimal concentration of coating antigen and the antibody was 0.05 and 1 μg mL−1, respectively. The IC50 value and Amax value (the maximum optical density value at 450 nm) were the key parameters to evaluate the performance of this antibody in ELISA. In consideration of IC50 and Amax values from preliminary results (not shown), elimination of antibody mAb2E9 was enabled from these studies, while allowing for further analysis of antibody mAb2G4.
The fact that the antibody is meant for real sample analysis, and because food matrices are different or their pH value is affected by various additives, we sought to evaluate the potential interferences that may be encountered by the effects of pH and ionic strength on its performance in ELISA. While evaluating the effects of pH on its performance, it was observed that there was a slight elevation in the Amax value (2.135–2.194) with an increase in the pH value from pH 5.0 to 7.4 beyond which there was a sharp drop. On the other hand, the IC50 value did show a decrease with an increase in pH up to pH 7.4 after which a sharp increase in the IC50 value was observed ((a)). Following this result, PBS at pH 7.4 was the choice for subsequent ELISA.
Figure 3. (a) Effects of pH on the performance of immunoassay (with each curve having a six-point (6) repeat), n = 3. (b) Effects of ion concentration on the performance of immunoassay (with different percentages of NaCl in PBS buffer (0, 0.5, 1, 2 and 4%), each curve has a six-point (6)repeat), n = 3.
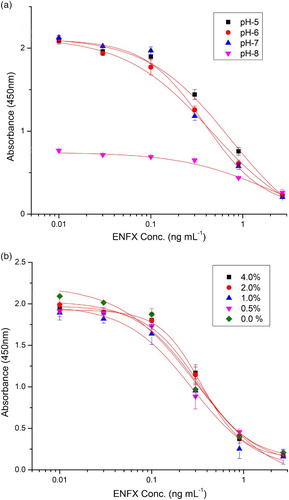
With regard to salt concentration, the IC50 value was found to be sensitive and the value increased from 0.243 ± 0.041 to 0.343 ± 0.02 ((b)) with increasing percentage salt strength (0, 0.5, 1, 2 and 4) in PBS. There was no observed trend on Amax value at the evaluated salt concentrations but the highest value was 2.264 at 0% salt concentration. Since these two values IC50 and Amax were a key to the evaluation of the performance of this antibody in the ELISA, we avoided salt concentration beyond that inherent in the experimental PBS as earlier outlined in the solutions and buffers section.
After optimising for salt and pH working values, we further followed to optimise using casein where concentrations of casein ranging from 0.01% to 0.5% in PBS were evaluated. In the subsequent results (), there was no significant difference observed in both Amax values with the highest being 2.208 and the lowest being 1.781 and the IC50 values in the range of 0.202–0.145 (highest to lowest). Based on this result, 0.05% casein in PBS was chosen as the optimal condition having given the best R2 (0.9902), a high ratio of Amax/IC50 and the spread was narrow among its values as compared to the other conditions used. All optimisation studies were carried out in triplicate.
Table 2. The effect of casein concentration on the antibody performance in ELISA.
Assay characteristics
A standard inhibition curve for ENFX generated using this ELISA system with optimised conditions is shown in . Six concentrations of ENFX were applied to the ELISA system. The lowest detectable level (LOD) of ENFX in this ELISA was 0.028 ng mL−1 and that of IC50 was 0.15 ng mL−1.
Antibody specificity
The specificity of the antibody was evaluated by performing competitive assays against 12 FQ analogues and the IC50 values (ng mL−1) obtained were used to calculate percentage cross-reactivity. The results are summarised in . The values represent the mean of three separate experiments each containing three replicates. The antibody demonstrated high affinity to its hapten (100%) but less affinity to the analogues’ hapten (<10%). These results are consistent with previous studies (Bucknall et al., Citation2003; Duan and Yuan, Citation2001; Huet et al., Citation2006; Watanabe et al., Citation2002), where the use of ENFX as a hapten reportedly produces primarily narrow, specific antibodies (Bucknall et al., Citation2003; Duan and Yuan, Citation2001; Wang et al., Citation2007).
Table 3. The IC50 and Cross-reactivities (CR) of the FQs in this ELISA study.
Recovery studies for ELISA
Three spiked levels (50, 100 and 200 µg kg−1) were tested for cow milk, beef liver and fish samples. Recoveries data were presented in . Intra- and inter-assay recoveries for milk ranged from 105.8% to 110.2% and from 90.2% to 104.7%, respectively. For liver, intra- and inter-assay recoveries ranged from 70.7% to 81.5% and from 70.7% to 80.7%, respectively, while fish samples gave a range between 89.1–101% and 90.4–99.6%, respectively. Matrix interference is common in recovery studies for ELISA. In this study, matrix effects were substantially narrowed by simple dilution (Chusri et al., Citation2013; Ebrahimzadeh, Mesgari, Abedimanesh, Ostadrahimi, & Gorbani, Citation2014; Watanabe et al., Citation2002) with water at a ratio of 1:250. The precision (% CV) between analyses ranged between 2.3% and 10.16%.
Table 4. The ENFX analysis of spiked cow milk, beef liver and fish samples with this ELISA kit.
Conclusions
In summary, we describe the synthesis of an immunogen that was successfully used to induce immune response in mice on immunisation. Consequently, the mouse whose serum showed the highest titre was sacrificed and its splenocytes were fused with SP 2/0 murine myeloma cells, and screened hybridoma cell lines were cloned and subcloned thrice by limiting dilution before they were used to produce anti-ENFX mAb.
The prepared anti-ENFX antibody was found to be very specific, sensitive and of high affinity during application. The mAb showed high sensitivity with an IC50 value of 0.15 ng mL−1. Spiked cow milk, beef liver and fish samples tested using this antibody had recoveries in the range of 90.2–110.6%, 70.7–81.5% and 89.1–101%, respectively, with intra-assay CV ranging from 3.4% to 9.43%, while inter-assay was between 2.3% and 10.16% showing satisfactory results. The indirect competitive ELISA method developed with this antibody had an LOD value of 0.028 ng mL−1 and was suitable for future use as a rapid screening method for detecting ENFX residues in samples.
Disclosure statement
No potential conflict of interest was reported by the authors.
Additional information
Funding
References
- Al-Mustafa, Z. H., & Al-Ghamdi, M. S. (2000). Use of norfloxacin in poultry production in the eastern province of Saudi Arabia and its possible impact on public health. International Journal of Environmental Research, 10, 291–299.
- Bucknall, S., Silverlight, J., Coldham, N., Thorne, L., & Jackman, R. (2003). Antibodies to the quinolones and fluoroquinolones for the development of generic and specific immunoassays for detection of these residues in animal products. Food Additives & Contaminants, 20, 221–228. doi: 10.1080/0265203021000055388
- Butaye, P., Devriese, L. A., & Haesebrouck, F. (2001). Differences in antibiotic resistance patterns of Enterococcus faecalis and Enterococcus faecium strains isolated from farm and pet animals. Antimicrobial Agents and Chemotherapy, 45, 1374–1378. doi: 10.1128/AAC.45.5.1374-1378.2001
- Cabello, F. C., Godfrey, H. P., Tomova, A., Ivanova, L., Dölz, H., Millanao, A., & Buschmann, A. H. (2013). Antimicrobial use in aquaculture re-examined: Its relevance to antimicrobial resistance and to animal and human health. Environmental Microbiology, 15, 1917–1942. doi: 10.1111/1462-2920.12134
- Cerniglia, C. E., & Kotarski, S. (1999). Evaluation of veterinary drug residues in food for their potential to affect human intestinal microflora. Regulatory Toxicology and Pharmacology, 29, 238–261. doi: 10.1006/rtph.1999.1300
- Chen, J. J., & Jiang, J. Q. (2013). Monoclonal antibody-based solvent tolerable indirect competitive ELISA for monitoring ciprofloxacin residue in poultry samples. Food and Agricultural Immunology, 24, 331–344. doi: 10.1080/09540105.2012.689817
- Chusri, M., Pitikarn, W., Tanapat, P., Songchan, P., Sarintip, S., & Kittinan, K. (2013). Production and characterization of a monoclonal antibody against Enrofloxacin. Journal of Microbiology and Biotechnology, 23, 69–75. doi: 10.4014/jmb.1201.01017
- Deng, X., Liu, L., Ma, W., Xu, C., Wang, L., & Kuang, H. (2011). Development and validation of a sandwich ELISA for quantification of peanut agglutinin (PNA) in foods. Food and Agricultural Immunology, 23, 265–272. doi: 10.1080/09540105.2011.617358
- Drlica, K., Zhao, X., Malik, M., Salz, T., & Kerns, R. (2012). Fluoroquinolone resistance: Mechanisms, restrictive dosing, and anti-mutant screening strategies for new compounds. In T. J. Dougherty, M. J. Pucci (Eds.), Antibiotic discovery and development, (pp. 485–514). New York, NY: Springer.
- Duan, J. H., & Yuan, Z. H. (2001). Development of an indirect competitive ELISA for ciprofloxacin residues in food animal edible tissues. Journal of Agricultural and Food Chemistry, 49, 1087–1089. doi: 10.1021/jf000091j
- Ebrahimzadeh, A. V., Mesgari, A. M., Abedimanesh, N., Ostadrahimi, A., & Gorbani, A. (2014). Investigation of Enrofloxacin and chloramphenicol residues in broiler chickens carcasses collected from local markets of Tabriz. Northwestern Iran Health Promotion Perspectives, 4, 151–157.
- European Commission. (2010). Commission regulation (EU) No 37/2010 of 22 December 2009 on pharmacologically active substances and their classification regarding maximum residue limits in foodstuffs of animal origin. Official Journal of the European Union – EUR-Lex, 20, L15.
- FDA. (2005). Withdrawal of Enrofloxacin for poultry effective on September, 12, 2005.
- Freitas, A., Barbosa, J., & Ramos, F. (2012). Determination of amoxicillin stability in chicken meat by liquid chromatography–Tandem mass spectrometry. Food Analytical Method, 5, 471–479. doi: 10.1007/s12161-011-9267-4
- Hooper, D. C. (2001). Emerging mechanisms of fluoroquinolone resistance. Emerging Infectious Diseases, 7, 337. doi: 10.3201/eid0702.010239
- Huang, B., Yin, Y., Lu, L., Ding, H., Wang, L., Yu, T., … Zhang, Y. (2010). Preparation of high-affinity rabbit monoclonal antibodies for ciprofloxacin and development of an indirect competitive ELISA for residues in milk. Journal of Zhejiang University Science B, 11, 812–818. doi: 10.1631/jzus.B1000055
- Huet, A. C., Charlier, C., Tittlemier, S. A., Singh, G., Benrejeb, S., & Delahaut, P. (2006). Simultaneous determination of (Fluoro) quinolone antibiotics in kidney, marine products, eggs, and muscle by enzyme-linked immunosorbent assay (ELISA). Journal of Agricultural and Food Chemistry, 54, 2822–2827. doi: 10.1021/jf052445i
- Jiang, W., Wang, Z., Beier, R. C., Jiang, H., Wu, Y., & Shen, J. (2013). Simultaneous determination of 13 fluoroquinolone and 22 sulfonamide residues in milk by a dual-colorimetric enzyme-linked immunosorbent assay. Analytical Chemistry, 85, 1995–1999. doi: 10.1021/ac303606h
- Jin-Lan, S., Chang, L., & Yue, S. (2012). Screening 36 veterinary drugs in animal origin food by LC-MS-MS combined with Modified QuEChERS Method Agilent Technologies Co, Ltd, Beijing, China (pp. 1–10).
- Jinqing, J., Haitang, Z., Zhixing, A., Zhiyong, X., Xuefeng, Y., Huaguo, H., & Ziliang, W. (2012). Development of an heterologous immunoassay for ciprofloxacin residue in milk. Physics Procedia, 25, 1829–1836. doi: 10.1016/j.phpro.2012.03.318
- Kuang, H., Xing, C., Hao, C., Liu, L., Wang, L., & Xu, C. (2013). Rapid and highly sensitive detection of lead ions in drinking water based on a strip immunosensor. Sensors, 13, 4214–4224. doi: 10.3390/s130404214
- Li, Y., Ji, B., Chen, W., Liu, L., Xu, C., Peng, C., & Wang, L. (2008). Production of new class-specific polyclonal antibody for determination of fluoroquinolones antibiotics by indirect competitive ELISA. Food and Agricultural Immunology, 19, 251–264. doi: 10.1080/09540100802471538
- Lolo, M., Pedreira, S., Miranda, J. M., Vázquez, B. I., Franco, C. M., Cepeda, A., & Fente, C. (2006). Effect of cooking on enrofloxacin residues in chicken tissue. Food Additives & Contaminants, 23, 988–993. doi: 10.1080/02652030600904894
- Lopes, R. P., Reyes, R. C., Romero-González, R., Frenich, A. G., & Vidal, J. L. M. (2012). Development and validation of a multiclass method for the determination of veterinary drug residues in chicken by ultra high performance liquid chromatography–Tandem mass spectrometry. Talanta, 89, 201–208. doi: 10.1016/j.talanta.2011.11.082
- Mi, T., Wang, Z., Eremin, S. A., Shen, J., & Zhang, S. (2013). Simultaneous determination of multiple (Fluoro)quinolone antibiotics in food samples by a one-step fluorescence polarization immunoassay. Journal of Agricultural and Food Chemistry, 61, 9347–9355. doi: 10.1021/jf403972r
- Ni, H., Zhang, S., Ding, X., Mi, T., Wang, Z., & Liu, M. (2014). Determination of Enrofloxacin in bovine milk by a novel single-stranded DNA Aptamer Chemiluminescent enzyme immunoassay. Analytical Letters, 47, 2844–2856. doi: 10.1080/00032719.2014.924009
- Paige, J. C., Tollefson, L., & Miller, M. A. (1999). Health implications of residues of veterinary drugs and chemicals in animal tissues. The Veterinary clinics of North America Food animal practice, 15, 31–43. doi: 10.1016/S0749-0720(15)30205-X
- Peng, J., Meng, X., Deng, X., Zhu, J., Kuang, H., & Xu, C. (2012). Development of a monoclonal antibody-based sandwich ELISA for the detection of ovalbumin in foods. Food and Agricultural Immunology, 25, 1–8. doi: 10.1080/09540105.2012.716398
- Perosa, F., Carbone, R., Ferrone, S., & Dammacco, F. (1990). Purification of human immunoglobulins by sequential precipitation with caprylic acid and ammonium sulphate. Journal of Immunological Methods, 128, 9–16. doi: 10.1016/0022-1759(90)90458-8
- Peterson, L. R. (2001). Quinolone molecular structure-activity relationships: What we have learned about improving antimicrobial activity. Clinical Infectious Diseases, 33, S180–S186. doi: 10.1086/321846
- Randall, L. P., Ridley, A. M., Cooles, S. W., Sharma, M., Sayers, A. R., Pumbwe, L., … Woodward, M. J. (2003). Prevalence of multiple antibiotic resistance in 443 Campylobacter spp. Isolated from humans and animals. Journal of Antimicrobial Chemotherapy, 52, 507–510. doi: 10.1093/jac/dkg379
- Rice, L. B. (2012). Mechanisms of resistance and clinical relevance of resistance to β-lactams, glycopeptides, and fluoroquinolones. Mayo Clinic Proceedings (issue 2, pp. 198–208).
- Roca, M., Castillo, M., Marti, P., Althaus, R. L., & Molina, M. P. (2010). Effect of heating on the stability of quinolones in milk. Journal of Agricultural and Food Chemistry, 58, 5427–5431. doi: 10.1021/jf9040518
- Standard (2009). Determination of enrofloxacin, danofloxacin, ciprofloxacin, sarafloxacin, orbifloxacin, difloxacin and marbofloxacin in milk and milk powder-LC-MS-MS method. Beijing.
- Sun, F., Liu, L., Kuang, H., & Xu, C. (2011). Development of ELISA for melamine detection in milk powder. Food and Agricultural Immunology, 24, 79–86. doi: 10.1080/09540105.2011.641170
- Suryoprabowo, S., Liu, L., Peng, J., Kuang, H., & Xu, C. (2014). Development of a broad specific monoclonal antibody for fluoroquinolone analysis. Food Analytical Method, 7, 2163–2168. doi: 10.1007/s12161-014-9863-1
- Temponi, M., Kageshita, T., Perosa, F., Ono, R., Okada, H., & Ferrone, S. (1989). Purification of murine IgG monoclonal antibodies by precipitation with caprylic acid: comparison with other methods of purification. Hybridoma, 8, 85–95. doi: 10.1089/hyb.1989.8.85
- Tittlemier, S. A., Gélinas, J., Dufresne, G., Haria, M., Querry, J., Cleroux, C., … Godefroy, S. B. (2008). Development of a direct competitive enzyme-linked immunosorbent assay for the detection of fluoroquinolone residues in shrimp. Food Analytical Method, 1, 28–35. doi: 10.1007/s12161-007-9004-1
- Wang, Z., Zhang, H., Ni, H., Zhang, S., & Shen, J. (2014). Development of a highly sensitive and specific immunoassay for enrofloxacin based on heterologous coating haptens. Analytica Chimica Acta, 820, 152–158. doi: 10.1016/j.aca.2014.02.043
- Wang, Z., Zhu, Y., Ding, S., He, F., Beier, R. C., Li, J., … Shen, J. (2007). Development of a monoclonal antibody-based broad-specificity ELISA for fluoroquinolone antibiotics in foods and molecular modeling studies of cross-reactive compounds. Analytical Chemistry, 79, 4471–4483. doi: 10.1021/ac070064t
- Watanabe, H., Satake, A., Kido, Y., & Tsuji, A. (2002). Monoclonal-based enzyme-linked immunosorbent assay and immunochromatographic assay for enrofloxacin in biological matrices. The Analyst, 127, 98–103. doi: 10.1039/b109427k