ABSTRACT
The study aimed to develop chitosan–alginate microcapsule as an oral delivery carrier for IgG. Bovine serum albumin (BSA) was used as the protein to be encapsulated while determining the microcapsule formula by evaluating encapsulation efficiency (EE), loading capacity (LC) and release profile. Results suggested that the optimal formula was composed of 0.1% chitosan, 0.5% CaCl2, 2% sodium alginate and the loading rate of BSA reached 25%. IgG was substituted for BSA and an IgG microcapsule was prepared following the above formula. The EE and LC of the resulted IgG microcapsule reached 76.83% and 18.53%. After 2 h incubation in simulated gastric fluid, the activity of IgG in the microcapsule remained at 79.79% and the total release rate of IgG in a simulated intestinal fluid reached 82.52%. This encapsulation formula was proved to be an effective oral delivery system which would protect the IgG from severe gastric conditions and guarantee an efficient release in the intestinal tract.
GRAPHICAL ABSTRACT
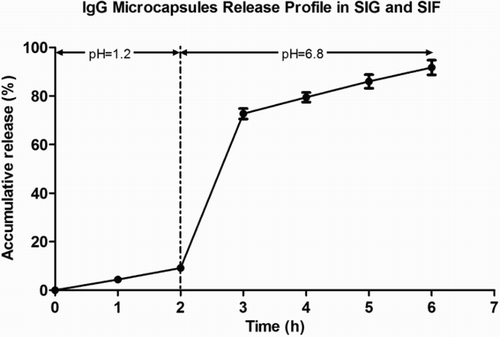
1. Introduction
IgG, a small-sized and Y-shaped monomer, is the main antibody isotype in humoral immunity which can easily reach the inflammatory site and has been developed for therapeutic applications (Erdemli, Keskin, & Tezcaner, Citation2015; Marquette et al., Citation2014). IgG also plays important roles in intestinal immunity, especially against enteric viral and bacterial infections (Asmuth et al., Citation2013; Besser, Gay, McGuire, & Evermann, Citation1988; Losonsky, Johnson, Winkelstein, & Yolken, Citation1985). Trans-membrane transportation of IgG from the blood into the intestinal cavity and oral consumption of IgG are two major sources of gut IgG. It is known that the orally administrated form of IgG can efficiently protect mucosal surfaces from infestation of rotavirus (Sarker et al., Citation1998), Escherichia coli (Quigley & Drew, Citation2000), cryptosporidium (Hunt et al., Citation2002) and other pathogens.
IgG, as a protein, is sensitive to the severe gastrointestinal ecosystem and is prone to lose its immuno-activity during delivery to the target site. To maximize the retention of its bioactivity, orally taken IgG requires effective protection against severe acidic gastric condition. Microencapsulation was proven as one of the most promising and efficient protective strategies for the delivery of bioactive proteins through harsh environment conditions and for the controlled release at the target site (Anal, Stevens, & Remuñán-López, Citation2006). More recently, although chitosan–alginate microcapsules have been extensively investigated as a controlled-release carrier and an oral delivery vehicle for proteins, polypeptides and cells (Jiang et al., Citation2014; Kamalian, Mirhosseini, Mustafa, & Manap, Citation2014; Li et al., Citation2007; Zhang, Wei, Lv, Wang, & Ma, Citation2011), its protective role for IgG is rarely reported.
Alginate, a kind of natural polysaccharide, has gel-forming ability in the presence of multivalent cations under mild conditions that allows preservation of the bioactive molecules and the viability of cells (Jen, Wake, & Mikos, Citation1996; Trabelsi et al., Citation2013). However, leakage of the core material due to the loose network limits its applications (Zhang et al., Citation2011). This can be improved by coating the alginate-Ca beads with a layer of polycations such as another natural polysaccharide – chitosan. The electrostatic interaction of carboxylic groups of alginate with the amine groups of chitosan results in the formation of a membrane surrounding the surface of the alginate-Ca beads and reduces their porosity (Huguet & Dellacherie, Citation1996; Sezer & Akbuğa, Citation1999). Thus the resulting microcapsules exhibit less release of inclusions at low pH (Hari, Chandy, & Sharma, Citation1996). Correspondingly, the payload, bio-adhesive property and permeability of the resulting microcapsules were also effectively enhanced (Shu & Zhu, Citation2002). Alginate and chitosan are nontoxic, biodegradable, muco-adhesive food grade materials (Murano, Citation1998).
Taking account of intestine immune roles of IgG, and active protein protection roles and low leakage of the core material at low pH of the chitosan–alginate microcapsule, encapsulation of IgG into alginate–chitosan beads was carried out in this study to determine the proper formula for oral delivery by the overall consideration of its encapsulation efficiency (EE), loading capacity (LC) and release profile.
2. Materials and methods
2.1. Materials
Chitosan (>98%), sodium alginate (50–800 cps), pepsin (1:3000) and trypsin (1:3000) were purchased from Sangon Biotech (Shanghai, China). Chicken immunoglobulin G (IgG), rabbit anti-chicken IgG, horseradish peroxidase (HRP)-conjugated rabbit anti-chicken IgG and 96-well plates were obtained from Beijing Boya Hongxing technology development Co., Ltd. (Beijing, China). Bovine serum albumin (BSA), tetramethylbenzidine (TMB) and Tween-20 were products of Sigma-Aldrich (USA).
2.2. Optimization of microcapsule formulation
Instead of IgG, BSA was used as protein being encapsulated in determination of the optimal composition of the chitosan–alginate microcapsule. Briefly, BSA was dissolved into aqueous sodium alginate and extruded into the encapsulation medium at a rate of 3 mL/min through a 0.8 mm (outer diameter) needle by a constant-flow pump. To make the encapsulation medium, chitosan was firstly dissolved into 10% glacial acetic acid, then diluted by deionized water to the required concentration, and CaCl2 was added and dissolved into the chitosan solution. The encapsulation medium was adjusted to pH 4.0 using glacial acetic acid before vacuum degassing. The needle tip was kept 10 cm away from the surface of the encapsulation medium. After 30 min of stirring, the resulted microcapsules were rinsed by deionized water and lyophilized under 30–50 pa at 15°C.
The concentrations of chitosan (0, 0.05%, 0.1%, 0.2%, 0.4%, 0.8%; w/v), CaCl2 (0.05%, 0.1%, 0.5%, 1%, 1.5%; w/v), sodium alginate (1%, 1.5%, 2%, 2.5%, 3%; w/v) and BSA loading rate (12.5%, 25%, 50%, 75%, 100%; BSA/sodium alginate; w/w) were selected by overall consideration of EE, LC and release properties of the prepared microcapsule.
2.2.1. Evaluation of EE and LC
Ten milligram freeze-dried BSA microcapsules were dissolved into 5 mL sterilized release solution (0.2M NaHCO3, 0.6M Na3C6H5O7·2H2O, pH 9.6) and shaken at 150 r/min for 2 h at 37°C. EE was calculated by:(1)
where CP is the mass of the released protein, and WTP is the total mass of the protein used for preparation of the microcapsule. Protein concentration was determined by Bradford assay. The LC was expressed as the percentage of CP compared to the total dried mass of microcapsule.
2.2.2. Analysis of release properties in vitro
Ten milligram freeze-dried microcapsules were placed in 10 mL simulated gastric fluid (SGF, containing 3.2 mg/mL pepsin and 0.03 mol/L NaCl, pH 1.2) with shaking at 150 r/min for 2 h at 37°C, then transferred into 10 mL simulated intestinal fluid (SIF, containing 0.05 mol/L KH2PO4 and 10 mg/mL trypsin, pH 6.8), incubated at 37°C with shaking at 150 r/min for 4 h. Each hour, 200 μL fluid was removed for protein concentration assay. After each sampling, the system was replenished with 200 μL fresh SGF or SIF. The accumulative release percentage (AR%) was obtained by:(2)
where Cn is the sample concentration at time Tn, V is the total volume of release fluid, WDM is the total mass of dried microcapsules and Vi is the sampling volume at Ti (both V0 and C0 were equal to zero).
2.3. Preparation and in vitro performance of IgG microcapsules
2.3.1. Preparation
IgG microcapsules were prepared according to the concentrations of chitosan, CaCl2 and sodium alginate listed in Section 2.2. The loading rates of IgG were equal to those listed for BSA. EE and LC were measured as described in Section 2.2.1.
2.3.2. Protection of IgG against SGF
The activity of non-encapsulated IgG was directly analyzed every 5 min during 30 min incubation in physiological saline, pepsin-free SGF or SGF. IgG microcapsules were immediately transferred from SGF into the release solution for another 2 h at 37°C with shaking at 150 r/min. After being centrifuged at 4500 r/min for 10 min at 4°C, the supernatant was checked for the activity of IgG. To demonstrate the protective effect of microencapsulation, another sample of IgG microcapsule was directly incubated in the release solution without exposure to SGF as control.
2.3.3. IgG release profile in vitro
The release capacity of IgG microcapsules was evaluated according to the method described in Section 2.2.2.
2.3.4. Assay of IgG activity
The immuno-activity of IgG was determined by enzyme linked immunosorbent assay (ELISA). Briefly, wells were coated with 100 μL rabbit anti-chicken IgG and incubated overnight at 4°C. After blocking with 3% BSA, IgG or sample was added and the plate was incubated at 37°C for 2 h, then HRP-conjugated rabbit anti-chicken IgG was added allowing the antigen–antibody binding. Finally, after thoroughly washing, 100 μL TMB substrate were added into the wells for enzymatic reaction. Fifty microliters 2 mol/L H2SO4 was used to stop the reaction. Finally the immuno-activity of IgG was determined at 450/630 nm by a plate reader (DNM-9606, Prolong New Technology Co., Ltd., China).
2.4. Statistical analysis
The mean of results and standard deviations (SD) were calculated based on at least three independent evaluations (n = 3). One-way ANOVA followed by Tukey’s post-hoc test were performed using SAS 8.1 software (SAS Institute Inc.) to assess differences between the samples at the level of p < .05.
3. Results
3.1. The formula of chitosan–alginate microcapsule
3.1.1. Chitosan concentration
Under the condition of 1% Ca2+, 2% alginate and 25% loading rate of BSA, the EE and the LC of the microcapsule showed a similar tendency in response to the changes of chitosan concentration. Both EE and LC increased dramatically (p < .05) when the chitosan concentration (w/v) increased from 0% to 0.1% ((a)). At higher concentrations over 0.1%, they changed only slightly and showed no significant increase (p > .05).
Figure 1. The effect of chitosan concentration on the microcapsule property. (a) LC and EE, (b) accumulative release. Other components include 1% (w/v) CaCl2, 2% (w/v) sodium alginate and 25% (w/w) BSA.
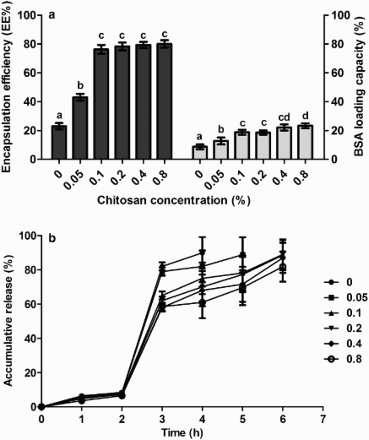
Release profiles of BSA were not greatly affected by chitosan concentration ((b)). During 2 h exposure to SGF the total release was no more than 10%. When transferred to SIF, a burst release of BSA was observed in the first hour. Therefore, 0.1% chitosan was chosen for the microcapsule formula.
3.1.2. CaCl2 concentration
Different levels of CaCl2 were used to prepare microcapsules together with 0.1% chitosan, 2% alginate and 25% BSA loading rate. Results showed that both EE and LC were adversely affected by CaCl2 concentration ((a)). At 0.05% CaCl2, they both reached peak values of 80.44% (EE) and 22.41% (LC). However at 0.05% and 0.1%, the prepared microcapsules were fragile and were easily broken. 0.5% CaCl2 endowed the prepared capsule a tough structure, and a higher EE and LC than the level of 1% and 1.5%. In regard to the release percentage ((b)), at different levels of CaCl2, less than 10% BSA was released in SGF and 69–72% BSA were released in the first hour in SIF. In the subsequent 3 h, the release rate was very slow and reached approximately 90%. Therefore, 0.5% CaCl2 was selected for the formula of the chitosan–alginate microcapsule.
3.1.3. Sodium alginate concentration
Microcapsules were prepared using 0.1% chitosan, 0.5% CaCl2, 25% BSA loading rate and different levels of sodium alginate. EE and LC were significantly influenced by the level of sodium alginate ((a)). Both increased to peak values of 74.07% and 16.44%, respectively, when the level of alginate increased from 1.0% to 2.0%, and then decreased at higher levels. Meanwhile lower levels of alginate were associated with larger release of BSA ((b)) in SGF. There continued to be a burst release of BSA in the first hour in SIF. The best, and the most, economic level of sodium alginate was selected to be 2.0%.
3.1.4. BSA loading rate
In a combination of the above optimized levels of chitosan, CaCl2 and alginate, different loading rates of BSA were used to prepare the microcapsule. The results showed ((a)) that a higher loading rate of BSA caused a lower EE and a higher LC. In the release experiments, at loading rates (BSA to alginate, w/w) of 12.5% and 25%, less than 10% BSA was released from the microcapsule in SGF and over 77.96% BSA was released in SIF. Higher rates cannot meet the requirements for gastrointestinal release and cannot protect the core material through the stomach. Hence, 25% was selected as the loading rate of BSA.
3.2. Preparation and characteristics of IgG microcapsules
3.2.1. Preparation of IgG microcapsules
Following the optimal formulation comprising 0.2% chitosan, 0.5% CaCl2, 2% alginate and 25% BSA loading rate, the resulted microcapsules were smooth, spherical, tough and homogeneous. High EE (76.83%) and high LC (18.53%) were achieved. The results were similar to those of Li et al. (Citation2008) and Zhang et al. (Citation2011).
3.2.2. Protection of IgG activity against SGF
As the oral delivery carrier of IgG, microcapsules have to travel through the gastric tract before reaching the destination site. The retention of IgG activity after passing through the stomach is a key factor in assessing an oral delivery system. The remaining IgG activity was evaluated by ELISA after incubating the microcapsules in SGF (pH 1.2) in vitro. Results demonstrated that non-encapsulated IgG retained only 53.84% relative activity after 30 min in pepsin-free SGF, and was almost completely degraded (8.02% relative activity) in SGF. For the microencapsulated IgG, the relative activity after 2 h incubation in SGF still remained at 79.79%. Compared to the result of Li et al. (Citation2008) (70%), the residual activity was higher. Most of the IgG was effectively protected by chitosan–alginate encapsulation against SGF.
3.2.3. IgG release profile in vitro
The release amount of encapsulated IgG in SGF was 9.15% (). After transferring the microcapsules from SGF to SIF, a burst release of IgG (63.49%) was detected in the first hour in SIF and reached 82.52% after 4 h. In total, 91.67% of IgG was released from the microcapsule after 2 h in SGF and 4 h in SIF. The results demonstrated that the microcapsule effectively controlled the release of orally administered encapsulated IgG. It inhibited the release in SGF and exhibited good release ability in SIF where IgG plays specific roles in binding or neutralizing pathogens or toxins.
4. Discussion
The target destination of orally administered IgG is usually the intestinal tract, while the results of this study showed that without protection IgG was destroyed or lost its activity within 30 min by the combined action of low pH and pepsin, and thus it needs protection against the severe gastric environment. Microcapsulation is an effective strategy to protect probiotics and other bioactive substances. Chitosan and alginate are widely developed as microencapsulating wall material due to their appropriate biocompatibility, non-toxicity, low cost and abundance in nature, so this kind of IgG microcapsule was prepared and its protective role was investigated in the present study.
In addition to EE and LC, the ability to control release should also be considered during preparation of the microcapsule. The tested concentrations of chitosan, sodium alginate and CaCl2 had little effect on release of the BSA in SGF; while only the loading rate of 12.5% and 25% showed minimal release, over 60% of BSA was released in SGF when the loading rate surpassed 25%. pH sensitivity is an attribute of chitosan–alginate microcapsules which have little water-binding ability and swelling ability at low pH such as in SGF (pH 1.2) and would significantly swell and release the core material at high pH such as in SIF (pH 6.8). This attribute was found to be broken if more core material is embedded, so that loading rates in excess of 25% would adversely affect the protective role of the microcapsule. This is in accordance with the results of Li et al. (Citation2008).
Both EE and LC increased with the increased concentration of chitosan from 0% to 0.1%. This is because more chitosan coated on the surface of capsule reduced the porosity of the capsule (Shu & Zhu, Citation2002). It is also reported that the bio-adhesive ability of microcapsules to the gastrointestinal tract, such as stomach (GåserØd, Jolliffe, Hampson, Dettmar, & Skjåk-Baek, Citation1998), small intestine, ileum, colon (Ping, Stanley, & Lisbeth, Citation1998; Shimoda, Onishi, & Machida, Citation2001) and buccal mucosa (Miyazaki, Nakayama, Oda, Takada, & Attwood, Citation1995; Remuñán-López, Portero, Vila-Jato, & Alonso, Citation1998) could also be improved by coating with chitosan.
For a one-stage procedure, it was observed that external gelation reduced the loss of BSA during the 24 h acid incubation period (Vandenberg & De La Noüe, Citation2001). The heterogeneity of the alginate gel can be affected by the external Ca2+ concentration: a decrease of Ca2+ level will enlarge the concentration gradient between the core and the microcapsule surface, thus providing a relatively non-porous external shell around the core, which will restrict the diffusion of the core material outside of microcapsules to the water phase (Li et al., Citation2007). This is well illustrated by increased EE and LC with the decreased Ca2+ level in this study.
The amount of negative charges on the surface of the capsule increased with the increased alginate concentration, and thus increased the amount of chitosan coated around the capsule surface (Gåserød, Smidsrød, & Skjåk-Baek, Citation1998; Shu & Zhu, Citation2002). Therefore it is likely that the release of BSA decreased with the increase of alginate concentration because a tight low-porosity outer layer was formed by the increased amount of chitosan. This also explains why the controlled-release ability of the microcapsule improved with the increased level of the alginate. Moreover, the increase of alginate concentration led to the decrease of capsule porosity (Gåserød et al. Citation1998), and hence reduced the loss of BSA and IgG during preparation, and improved the EE and load capacity of capsules. Shu and Zhu (Citation2002) and Li et al. (Citation2008) obtained similar results.
IgG microcapsules were prepared using the optimized formula for BSA. The molecular weight, degree of ionization and specific conformation of the protein being encapsulated have great influence on the release profile (Huguet & Dellacherie, Citation1996). The capacity of BSA to deform can also enhance its ability to diffuse out of the chitosan–alginate microcapsules. Moreover, the ionization of BSA in the initial sodium alginate–BSA solution (pH 6–7.2) affects BSA retention in microcapsules. For these reasons, we checked EE, LC, release profile and remaining IgG activity after SGF treatment of the prepared IgG microcapsules. Results demonstrated that although EE and LC were slightly lower than for BSA microcapsules, EE of IgG microcapsules was higher than that of Marquette et al. (Citation2014), Li et al. (Citation2008) and Zhang et al. (Citation2011), and LC was similar to the results of Li et al. (Citation2008).
The study confirmed that chitosan–alginate microcapsules are pH sensitive (Anal, Bhopatkar, Tokura, Tamura, & Stevens, Citation2003; Anal & Stevens, Citation2005). In acidic solution, due to the ionic bond between the chitosan and alginate (Knorr & Daly, Citation1988), as well as the physical barrier provided by the interphasic membrane itself (Zhang et al., Citation2011), IgG microcapsules maintained integrity well, and effectively prevented the direct exposure of IgG to the gastric fluid. As a result only less than 10% of IgG was released in SGF and 80% IgG activity was preserved. When transferring into SIF, during the first hour, a burst release of IgG was observed. Li et al. thought this is because in the neutral or alkaline solution, the network of the Ca2+–chitosan–alginate complex disintegrated, during which hydroxyl ions displaced the anionic alginate and the positive charges possessed by chitosan disappeared (Li et al., Citation2007). Silva, Ribeiro, Ferreira, and Veiga (Citation2006) thought the burst release of the encapsulated material after transferring from SGF into SIF was mainly because of the reduced gel strength after acidic treatment.
In conclusion, the optimized formula for BSA microcapsules was composed of 0.1% chitosan, 0.5% CaCl2, 2% sodium alginate and 20% loading rate. The EE and LC of the IgG microcapsule prepared following the above formula reached 76.83% and 18.53%, respectively. Encapsulation of IgG into chitosan–alginate microcapsules was an efficient oral delivery system to protect IgG through severe gastric conditions and ensure a large release of IgG in the intestinal tract.
Acknowledgements
Special thanks go to Professor Decong Zheng (College of Animal Science and Veterinary Medicine, Shanxi Agricultural University) and Associate Professor Guofeng Duan (college of horticulture, Shanxi Agricultural University) for their help with data analysis.
Disclosure statement
No potential conflict of interest was reported by the authors.
Notes on contributors
Zhiyuan Ren, Ms Ag. Lecturer, College of Food Sciences and Engineering, Shanxi Agricultural University. Ren's research field interests are bioengineering, food nutrition and immunology, and aromatic compounds in vine.
Xinrong Zhang, Ms Ag. Zhang's field of research is food bioengineering.
Yu Guo, Ms Ag. Lecturer, College of Food Sciences and Engineering, Shanxi Agricultural University. Guo's research fields include food nutrition, isolation and analysis of active phytochemicals.
Keguang Han, Ms Ag. Associate professor, College of Animal Science and Veterinary Medicine, Shanxi Agricultural University. Han's research fields include molecular mechanism of inflammation and immune pharmacology.
Nairui Huo, Ph.D., Professor, College of Animal Science and Veterinary Medicine, Shanxi Agricultural University. Huo's research fields include bioengineering and food safety.
Additional information
Funding
References
- Anal, A. K., Bhopatkar, D., Tokura, S., Tamura, H., & Stevens, W. F. (2003). Chitosan-alginate multilayer beads for gastric passage and controlled intestinal release of protein. Drug Development and Industrial Pharmacy, 29(6), 713–724. doi: 10.1081/DDC-120021320
- Anal, A. K., & Stevens, W. F. (2005). Chitosan-alginate multilayer beads for controlled release of ampicillin. International Journal of Pharmaceutics, 290(1–2), 45–54. doi: 10.1016/j.ijpharm.2004.11.015
- Anal, A. K., Stevens, W. F., & Remuñán-López, C. (2006). Ionotropic cross-linked chitosan microspheres for controlled release of ampicillin. International Journal of Pharmaceutics, 312(1–2), 166–173. doi: 10.1016/j.ijpharm.2006.01.043
- Asmuth, D. M., Ma, Z. M., Albanese, A., Sandler, N. G., Devaraj, S., Knight, T. H., … Miller, C. J. (2013). Oral serum-derived bovine immunoglobulin improves duodenal immune reconstitution and absorption function in patients with HIV enteropathy. AIDS, 27(14), 2207–2217. doi: 10.1097/QAD.0b013e328362e54c
- Besser, T. E., Gay, C. C., McGuire, T. C., & Evermann, J. F. (1988). Passive immunity to bovine rotavirus infection associated with transfer of serum antibody into the intestinal lumen. Journal of Virology, 62(7), 2238–2242.
- Erdemli, Ö, Keskin, D., & Tezcaner, A. (2015). Influence of excipients on characteristics and release profiles of poly(ε-caprolactone) microspheres containing immunoglobulin G. Materials Science and Engineering: C, 48, 391–399. doi: 10.1016/j.msec.2014.12.044
- GåserØd, O., Jolliffe, I. G., Hampson, F. C., Dettmar, P. W., & Skjåk-Baek, G. (1998). The enhancement of the bioadhesive properties of calcium alginate gel beads by coating with chitosan. International Journal of Pharmaceutics, 175(2), 237–246. doi: 10.1016/S0378-5173(98)00277-4
- Gåserød, O., Smidsrød, O., & Skjåk-Baek, G. (1998). Microcapsules of alginate-chitosan--I. A quantitative study of the interaction between alginate and chitosan. Biomaterials, 19(20), 1815–1825. doi: 10.1016/S0142-9612(98)00073-8
- Hari, P. R., Chandy, T., & Sharma, C. P. (1996). Chitosan/calcium—alginate beads for oral delivery of insulin. Journal of Applied Polymer Science, 59(11), 1795–1801. doi: 10.1002/(SICI)1097-4628(19960314)59:11<1795::AID-APP16>3.0.CO;2-T
- Huguet, M. L., & Dellacherie, E. (1996). Calcium alginate beads coated with chitosan: Effect of the structure of encapsulated materials on their release. Process Biochemistry, 31(8), 745–751. doi: 10.1016/S0032-9592(96)00032-5
- Hunt, E., Fu, Q., Armstrong, M. U., Rennix, D. K., Webster, D. W., Galanko, J. A., … Rhoads, J. M. (2002). Oral bovine serum concentrate improves cryptosporidial enteritis in calves. Pediatric Research, 51(3), 370–376. doi: 10.1203/00006450-200203000-00017
- Jen, A. C., Wake, M. C., & Mikos, A. G. (1996). Review: Hydrogels for cell immobilization. Biotechnology and Bioengineering, 50(4), 357–364. doi: 10.1002/(SICI)1097-0290(19960520)50:4<357::AID-BIT2>3.3.CO;2-F
- Jiang, T., Singh, B., Maharjan, S., Li, H. S., Kang, S. K., Bok, J. D., … Choi, Y.-J. (2014). Oral delivery of probiotic expressing M cell homing peptide conjugated BmpB vaccine encapsulated into alginate/chitosan/alginate microcapsules. European Journal of Pharmaceutics and Biopharmaceutics, 88(3), 768–777. doi: 10.1016/j.ejpb.2014.07.003
- Kamalian, N., Mirhosseini, H., Mustafa, S., & Manap, M. Y. (2014). Effect of alginate and chitosan on viability and release behavior of Bifidobacterium pseudocatenulatum G4 in simulated gastrointestinal fluid. Carbohydrate Polymers, 111, 700–706. doi: 10.1016/j.carbpol.2014.05.014
- Knorr, D., & Daly, M. (1988). Mechanics and diffusional changes observed in multilayer chitosan/alginate coacervate capsules. Process Biochem, 23, 48–50.
- Li, X. Y., Jin, L. J., MacAllister, T. A., Stanford, K., Xu, J. Y., Lu, Y. N., … Xu, Y.-P. (2007). Chitosan-alginate microcapsules for oral delivery of egg yolk immunoglobulin (IgY). Journal of Agricultural and Food Chemistry, 55(8), 2911–2917. doi: 10.1021/jf062900q
- Li, X. Y., Jin, L. J., Zhen, Y. H., Lu, Y. N., Wang, L. H., & Xu, Y. P. (2008). Optimization and characterization of chitosan-alginate microcapsules containing egg yolk immunoglobulin (IgY). Journal of Biotechnology, 136, s258.
- Losonsky, G. A., Johnson, J. P., Winkelstein, J. A., & Yolken, R. H. (1985). Oral administration of human serum immunoglobulin in immunodeficient patients with viral gastroenteritis. A pharmacokinetic and functional analysis. Journal of Clinical Investigation, 76(6), 2362–2367. doi: 10.1172/JCI112248
- Marquette, S., Peerboom, C., Yates, A., Denis, L., Goole, J., & Amiqui, K. (2014). Encapsulation of immunoglobulin G by solid-in-oil-in-water: Effect of process parameters on microsphere properties. European Journal of Pharmaceutics and Biopharmaceutics, 86(3), 393–403. doi: 10.1016/j.ejpb.2013.10.013
- Miyazaki, S., Nakayama, A., Oda, M., Takada, M., & Attwood, D. (1995). Drug release from oral mucosal adhesive tablets of chitosan and sodium alginate. International Journal of Pharmaceutics, 118(2), 257–263. doi: 10.1016/0378-5173(94)00396-M
- Murano, E. (1998). Use of natural polysaccharides in the microencapsulation techniques. Journal of Applied Ichthyology, 14(3–4), 245–249. doi: 10.1111/j.1439-0426.1998.tb00650.x
- Ping, H., Stanley, S. D., & Lisbeth, I. (1998). In vitro evaluation of the mucoadhesive properties of chitosan microspheres. International Journal of Pharmaceutics, 166(1), 75–88. doi: 10.1016/S0378-5173(98)00027-1
- Quigley, J. D., & Drew, M. D. (2000). Effects of oral antibiotics or bovine plasma on survival, health and growth in dairy calves challenged with Escherichia coli. Food and Agricultural Immunology, 12(4), 311–318. doi: 10.1080/09540100020008173
- Remuñán-López, C., Portero, A., Vila-Jato, J. L., & Alonso, M. J. (1998). Design and evaluation of chitosan/ethylcellulose mucoadhesive bilayered devices for buccal drug delivery. Journal of Controlled Release, 55(2–3), 143–152. doi: 10.1016/S0168-3659(98)00044-3
- Sarker, S. A., Casswall, T. H., Mahalanabis, D., Alam, N. H., Albert, M. J., Brüssow, H., … Hammerström, L. (1998). Successful treatment of rotavirus diarrhea in children with immunoglobulin from immunized bovine colostrum. The Pediatric Infectious Disease Journal, 17(12), 1149–1154. doi: 10.1097/00006454-199812000-00010
- Sezer, A. D., & Akbuğa, J. (1999). Release characteristics of chitosan treated alginate beads: II. Sustained release of a low molecular drug from chitosan treated alginate beads. Journal of Microencapsulation, 16(6), 687–696. doi: 10.1080/026520499288636
- Shimoda, J., Onishi, H., & Machida, Y. (2001). Bioadhesive characteristics of chitosan microspheres to the mucosa of rat small intestine. Drug Development and Industrial Pharmacy, 27(6), 567–576. doi: 10.1081/DDC-100105182
- Shu, X. Z., & Zhu, K. J. (2002). The release behavior of brilliant blue from calcium-alginate gel beads coated by chitosan: The preparation method effect. European Journal of Pharmaceutics and Biopharmaceutics, 53(2), 193–201. doi: 10.1016/S0939-6411(01)00247-8
- Silva, C. M., Ribeiro, A. J., Ferreira, D., & Veiga, F. (2006). Insulin encapsulation in reinforced alginate microsphere prepared by internal gelation. European Journal of Pharmaceutical Sciences, 29(2), 148–159. doi: 10.1016/j.ejps.2006.06.008
- Trabelsi, I., Bejar, W., Ayadi, D., Chouayekh, H., Kammoun, R., Bejar, S., & Ben Salah, R. (2013). Encapsulation in alginate and alginate coated-chitosan improved the survival of newly probiotic in oxgall and gastric juice. International Journal of Biological Macromolecules, 61, 36–42. doi: 10.1016/j.ijbiomac.2013.06.035
- Vandenberg, G. W., & De La Noüe, J. (2001). Evaluation of protein release from chitosan-alginate microcapsules produced using external or internal gelation. Journal of Microencapsulation, 18(4), 433–441. doi: 10.1080/02652040010019578
- Zhang, Y. L., Wei, W., Lv, P., Wang, L., & Ma, G. (2011). Preparation and evaluation of alginate-chitosan microspheres for oral delivery of insulin. European Journal of Pharmaceutics and Biopharmaceutics, 77(1), 11–19. doi: 10.1016/j.ejpb.2010.09.016