ABSTRACT
Inflammation is a pathological process that is known to be involved in numerous diseases. Microbial infection or tissue injury activates inflammatory responses, resulting in the induction of proinflammatory proteins including cyclooxygenase-2 (COX-2) and inducible nitric oxide synthase (iNOS). Aster yomena is used in traditional Korean remedies to treat cough, asthma, and insect bites. Here, we investigated the effects of A. yomena extract (EAY) on the expression of COX-2 and iNOS induced by LPS. EAY inhibited NF-κB activation and IκBα degradation induced by LPS. EAY suppressed LPS-induced COX-2 and iNOS expression which are the target genes regulated through NF-κB activation in macrophages. EAY also suppressed LPS-induced nitrite production. These results suggest that EAY has the potential to be developed as a potent anti-inflammatory drug.
Introduction
When various pathogens invade the body, the immune system contributes to the biological defenses, thus, inflammatory reactions occur for host defense to microbial infection or tissue injury, facilitating the restoration of tissue structure (Murakami & Ohigashi, Citation2007). However, prolonged inflammation is not beneficial, as it contributes to the pathology of numerous diseases including cancer. Microbial infection or tissue injury activates inflammatory responses, resulting in the induction of proinflammatory proteins. Inducible nitric oxide synthase (iNOS) and cyclooxygenase-2 (COX-2) are major enzymes associated with various inflammatory diseases (Gu et al., Citation2014; Moncada, Citation1999).
NOS catalyzes the oxidative deamination of L-arginine to produce NO (Moncada, Citation1999). NOS is classified into three isoforms according to the cell type, the location, and the manner of expression: neuronal NOS (nNOS), endothelial NOS (eNOS), and macrophage or inducible NOS (iNOS). nNOS and eNOS are constitutively expressed in vascular endothelial cells and neuronal tissues, respectively, whereas iNOS is induced by infectious and proinflammatory stimuli in a variety of cell types (Murakami, Takahashi, Koshimizu, & Ohigashi, Citation2003). iNOS is most readily observed in monocytes and macrophages from patients with inflammation-related diseases (Moncada, Citation1999), therefore, iNOS is a very important therapeutic target for the treatment of chronic inflammatory diseases.
Two isoforms of COX, designated COX-1 and COX-2, catalyze the conversion of arachidonic acid to prostanoids, which are important inflammatory response mediators (Rouzer & Marnett, Citation2009). The COX-1 enzyme is widely distributed and constitutively expressed in many normal tissues at relatively stable levels, whereas COX-2 is undetectable but is an inducible enzyme expressed in most normal mammalian tissue in response to physical, chemical, and biological stimuli (Murakami & Ohigashi, Citation2007). It has been reported that COX is the target of the nonsteroidal anti-inflammatory drugs (NSAIDs), which play a therapeutic role in the treatment of pain, fever, and inflammation (Vane, Citation1971).
The regulation of iNOS and COX-2 expression is a very important therapeutic avenue in the treatment of certain inflammatory diseases (Moncada, Citation1999; Turini & Dubois, Citation2002). Traditionally, many compounds of plant origin have been used to develop anti-inflammatory drugs. As part of our search for iNOS and COX-2 inhibitors from natural products, Aster yomena extract (EAY) was identified as a novel potent inhibitor of iNOS and COX-2 expression induced by LPS. In the present study, we found that EAY inhibited the LPS-induced expression of iNOS and COX-2 in RAW264.7 murine macrophage cells.
Materials and methods
Preparation of EAY
The A. yomena leaves were purchased from the Agricultural Technology Center, Gurye-eup, Gurye-gun, Jeollanam-do 57660, Republic of Korea. The plant was authenticated and a voucher specimen (Voucher SCH0722) was deposited at the Department of Botany, Soonchunhyang University, Republic of Korea. The dried leaves of A. yomena (1 kg) were extracted with 50% ethanol (9 L) three times at 87°C for 4 h, and then filtered (Whatman No. 2, 30-cm diameter). The 50% ethanol extract of A. yomena leaves was concentrated in vacuo at 45°C. The dried extract (12.1 g) was suspended in water (100 ml) and partitioned with n-hexane, methylene chloride, ethyl acetate, and water. The methylene chloride fraction of A. yomena was used in the present study.
Reagents
LPS was obtained from List Biological Laboratories (San Jose, CA, USA). All other reagents were purchased from Sigma-Aldrich unless otherwise indicated.
Cell culture
RAW264.7 cells (a murine monocytic cell line; ATCC TIB-71) were cultured in Dulbecco’s modified Eagle’s medium containing 10% (v/v) fetal bovine serum, 100 units/ml penicillin, and 100 μg/ml streptomycin. Cells were maintained at 37°C in a 5% CO2/95% air environment.
Cell viability test
Cell viability was assessed using a 3-(4,5-dimethylthiazol-2-yl)-5(3-carboxymethoxyphenyl)-2-(4-sulfophenyl)-2H-tetrazolium (MTS)-based colorimetric assay. Viability tests were performed by adding a small amount of the CellTiter 96 AQueous One Solution reagent (Promega, Madison, WI, USA) directly to the culture wells, incubating for 4 h, and then recording the absorbance at 490 nm with a 96-well plate reader.
Transfection and luciferase reporter gene assay
The assay was performed as previously described (Park & Youn, Citation2013; Shin, Shon, & Youn, Citation2013). Cells were cotransfected with a luciferase plasmid and a plasmid containing heat shock protein (HSP)70-β-galactosidase as an internal control, using SuperFect transfection reagent (Qiagen, Valencia, CA, USA) according to the manufacturer’s instructions. Luciferase enzyme activity was determined using a commercial luciferase assay system (Promega) according to the manufacturer’s instructions. Luciferase activity was normalized to β-galactosidase activity.
Western blotting analysis
Western blotting was performed as previously described (Ahn et al., Citation2015; Park, Citation2015). Equal amounts of cell extracts were subjected to 8% sodium dodecyl sulfate-polyacrylamide gel electrophoresis, and the separated proteins were electrotransferred to a polyvinylidene difluoride membrane. The membrane was blocked in phosphate-buffered saline containing 0.1% Tween-20 and 3% nonfat dried milk to prevent nonspecific binding of the antibodies. Immunoblotting was performed with the indicated primary antibodies and secondary antibodies conjugated to horseradish peroxidase (Amersham Biosciences, Arlington Heights, IL, USA). The reactive bands were visualized with enhanced chemiluminescence detection reagents (Intron, Seongnam, Gyeonggi-do, South Korea) and a Fusion Solo chemiluminescence system (Fisher Biotec, Wembley, WA, Australia).
Nitrite assay
Nitric oxide (NO) released from the RAW264.7 cells was assessed by determining the nitrite concentration in the culture supernatant (Lee et al., Citation2014). Samples (100 μl) of the culture medium were incubated with 150 μl Griess reagent (1% sulfanilamide and 0.1% naphthylethylene diamine in a 2.5% phosphoric acid solution) at room temperature for 10 min in a 96-well microplate. The absorbance at 540 nm was read using a plate reader, and the concentration of NO was then determined by the preparation of a standard calibration curve, using sodium nitrite as the standard.
Data analysis
Data were obtained from triplicate experiments. Values are expressed as the mean ± standard error of the mean (SEM). Differences in the data were evaluated using a Student’s t test. A p value of <.05 was taken to indicate a statistically significant difference.
Results and discussion
EAY inhibits LPS-induced NF-κB activation
To evaluate the cytotoxic nature of EAY in RAW 264.7 cells, cytotoxicity was assessed using a well-established viability assay based on the conversion of 3-(4,5-dimethylthiazol-2-yl)-5(3-carboxymethoxyphenyl)-2-(4-sulfophenyl)-2H-tetrazolium (MTS) to formazan. Treatment with 200 μg/ml EAY reduced population viability by 82% ((A)). Toxicity of EAY would not be a cause of any observed changes in experiments under a concentration of 200 μg/ml, therefore, all subsequent experiments utilized 100 μg/ml EAY.
Figure 1. EAY inhibits NF-κB activation induced by LPS. (A) Cell viability assay. (B) NF-κB luciferase reporter assay in RAW264.7 cells. Cells were pretreated with 50 or 100 μg/ml EAY for 1 h and then treated with LPS (10 ng/ml) for an additional 8 h. Cell lysates were prepared and luciferase enzyme activities were determined. Values are expressed as the mean ± SEM (n = 3). *, Significantly different from LPS alone, p < .05 (*). (C) Western blotting for IκBα and β-actin protein. Veh, vehicle; EAY, methylenechloride extracts of Aster yomena.
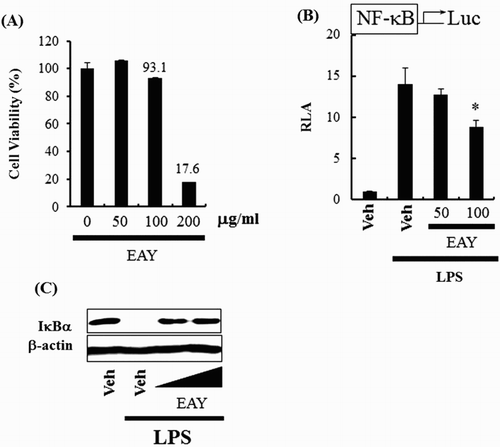
NF-κB is one of the most crucial transcription factors, regulating over 150 genes, many of which are associated with inflammation and cancer (Baldwin, Citation1996). NF-κB activation by proinflammatory stimuli from various pathogens upregulates the expression of numerous inflammatory gene products including cytokines, COX-2, and iNOS (Surh et al., Citation2001). The effects of EAY on NF-κB activation were determined using a NF-κB luciferase reporter assay. EAY inhibited NF-κB activation induced by LPS ((B)), and also inhibited LPS-induced IκBα degradation ((C)).
EAY suppresses LPS-induced COX-2 and iNOS expression
The next experiment assessed the capability of EAY to regulate COX-2 expression. COX-2 is one of the target genes regulated through NF-κB activation in macrophages. EAY suppressed the expression of COX-2 in RAW264.7 cells as determined by COX-2 luciferase reporter ((A)) and immunoblotting assays ((B)). Subsequently, we determined whether EAY could regulate iNOS expression, which is another target gene regulated through NF-κB activation. EAY suppressed the expression of iNOS in RAW264.7 cells as determined by iNOS luciferase reporter ((A)) and immunoblotting assays ((B)). EAY also suppressed LPS-induced nitrite production ((C)). These results support the suggestion that EAY inhibits the activation of NF-κB, resulting in the suppression of the expression of target genes such as COX-2 and iNOS.
Figure 2. EAY inhibits COX-2 expression induced by LPS. (A) COX-2 luciferase reporter assay in RAW264.7 cells. Cells were pretreated with 50 or 100 μg/ml EAY for 1 h and then treated with LPS (10 ng/ml) for an additional 8 h. Cell lysates were prepared and luciferase enzyme activities were determined. Values represent the mean ± SEM (n = 3). *, Significantly different from LPS alone, p < 0.05 (*). (B) Western blotting for COX-2 and β-actin protein. Note: Veh, vehicle; EAY, methylenechloride extracts of Aster yomena.
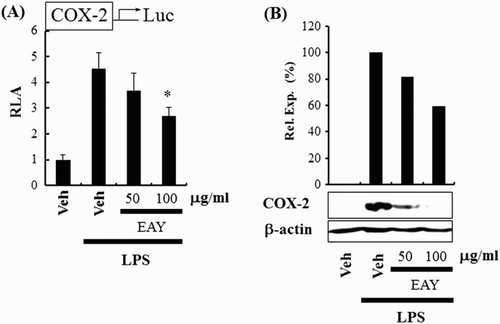
Figure 3. EAY inhibits iNOS expression induced by LPS. (A) iNOS luciferase reporter assay in RAW264.7 cells. Cells were pretreated with 50 or 100 μg/ml EAY for 1 h and then treated with LPS (10 ng/ml) for an additional 8 h. Cell lysates were prepared and luciferase enzyme activities were determined. Values represent the mean ± SEM (n = 3). *, Significantly different from LPS alone, p < .05 (*). (B) Western blotting for iNOS and β-actin protein. (C) NO assay. Values represent the mean ± SEM (n = 3). +, Significantly different from LPS alone, p < .01 (++). Note: Veh, vehicle; EAY, methylenechloride extracts of Aster yomena.
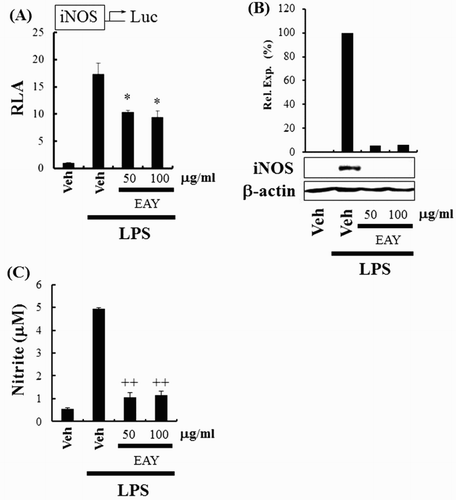
Inflammation can be initiated by microbial infection or tissue injury, facilitating the restoration of tissue structure (Murakami & Ohigashi, Citation2007). Toll-like receptors (TLRs), one of the pathogen recognition receptors, detect structural components derived from invading microorganisms or damaged cells/tissues. The activation of TLRs triggers immune and inflammatory responses through the activation of cellular signal transduction and the expression of immune and inflammatory mediators (Ahn et al., Citation2015). TLR4 is the first characterized TLR and recognizes LPS, a cell wall component of Gram negative bacteria that causes systemic inflammatory sepsis. Recognition of LPS by TLR4 results in oligomerization of TLR4 and subsequent recruitment of the adaptor molecules, myeloid differentiation primary response gene 88 (MyD88) and Toll/Interleukin-1 receptor domain-containing adaptor-inducing interferon beta (TRIF) (Ahn et al., Citation2015). MyD88 recruits IL-1R-associated kinase (IRAK)-4 and IRAK-1, which further associate with TNFR-associated factor 6 (TRAF6), leading to the activation of NF-κB. TRIF is another adaptor molecule responsible for the activation of MyD88-independent signaling pathways. TRIF activates receptor interacting protein 1, TANK-binding kinase 1 (TBK1), and IKKε, leading to the activation of various transcription factors including NF-κB and IRF3/7 (Gu et al., Citation2015). The activation of TLR4 culminates in the production of proinflammatory proteins including iNOS and COX-2. Deregulated TLR activity is known to be closely linked to the development of chronic inflammatory diseases, as well as immunological disorders. Therefore, TLRs and their downstream signaling components can be good therapeutic targets for many inflammatory diseases.
In previous studies, six phenolic compounds were isolated from the aerial parts of A. yomena (Kim, Jin, Jin, Ko, & Woo, Citation2014); esculetin, 4-O-β-D-glucopyranoside-3-hydroxy methyl benzoate, caffeic acid, isoquercitrin, isorhamnetin-3-O-glucoside, and apigenin. These phenolic compounds inhibit IL-6 induced by TNFα (Kim et al., Citation2014). It has also been reported that A. yomena inhibits asthma by suppressing Th2-type cytokines and eosinophils in the bronchoalveolar lavage fluid and ovalbumin-specific IgE in serum (Sim et al., Citation2014).
Conclusions
In the present study, we investigated whether EAY could regulate the activation of NF-κB and the expression of COX-2 and iNOS, which are important components of the inflammatory response. They are activated in response to stimuli of various pathogens, following rapid activation of target genes involved in innate and adaptive immune responses and inflammation (Hacker & Karin, Citation2006). EAY suppressed NF-κB activation and COX-2 and iNOS expression induced by LPS. These results suggest that EAY can regulate the TLR4 signaling pathway, leading to decreased inflammatory gene expression.
supplemental_files.zip
Download Zip (102.4 KB)Disclosure statement
No potential conflict of interest was reported by the authors.
Notes on contributors
Ji-Soo Kim, Graduate Student, Department of Medical Science, College of Medical Sciences, Soonchunhyang University, Asan, Republic of Korea.
Ah-Yeon Kim, Undergraduate Student, Department of Biomedical Laboratory Science, College of Medical Sciences, Soonchunhyang University, Asan, Republic of Korea.
Hyeon-Myeong Shin, Undergraduate Student, Department of Biomedical Laboratory Science, College of Medical Sciences, Soonchunhyang University, Asan, Republic of Korea.
Sang-Il Ahn, Graduate Student, Department of Medical Science, College of Medical Sciences, Soonchunhyang University, Asan, Republic of Korea.
Hyun-Jin Shim, Undergraduate Student, Department of Biomedical Laboratory Science, College of Medical Sciences, Soonchunhyang University, Asan, Republic of Korea.
Kung-Woo Nam, Professor, Department of Life Science and Biotechnology, College of Natural Science, Soonchunhyang University, Asan, Republic of Korea.
Kyung-A Hwang, Researcher, Department of Agrofood Resources, National Academy of Agricultural Science, RDA, Wanju, Republic of Korea.
Hyung-Sun Youn, Professor, Department of Biomedical Laboratory Science, College of Medical Sciences, Soonchunhyang University, Asan, Republic of Korea.
Additional information
Funding
References
- Ahn, S. I., Lim, S. J., Gu, G. J., Hong, C. Y., Kim, J. S., Jeong, H. J., … Youn, H. S. (2015). Suppressive effects of 1-[4-fluoro-2-(2-nitrovinyl)phenyl]pyrrolidine on the Toll-like receptor signaling pathways. International Immunopharmacology, 24(1), 36–41. doi: 10.1016/j.intimp.2014.10.033
- Baldwin, A. S., Jr. (1996). The NF-kappa B and I kappa B proteins: New discoveries and insights. Annual Review of Immunology, 14, 649–683. doi: 10.1146/annurev.immunol.14.1.649
- Gu, G. J., Ahn, S. I., Kim, J. S., Hong, C. Y., Lee, S. C., Chang, Y. T., … Youn, H. S. (2015). Suppression of the TRIF-dependent signaling pathway of Toll-like receptor by CDr10b in RAW264.7 macrophages. International Immunopharmacology, 28(1), 29–33. doi: 10.1016/j.intimp.2015.05.017
- Gu, G. J., Lim, S. J., Ahn, S. I., Lee, S. C., Chang, Y. T., Choi, T. H., … Youn, H. S. (2014). CDr10b inhibits the expression of cyclooxygenase-2 and inducible nitric oxide synthase induced by lipopolysaccharide. European Journal of Pharmacology, 742, 42–46. doi: 10.1016/j.ejphar.2014.08.036
- Hacker, H., & Karin, M. (2006). Regulation and function of IKK and IKK-related kinases. Science's STKE, 2006(357), re13. doi: 10.1126/stke.3572006re13
- Kim, A. R., Jin, Q., Jin, H. G., Ko, H. J., & Woo, E. R. (2014). Phenolic compounds with IL-6 inhibitory activity from Aster yomena. Archives of Pharmacal Research, 37(7), 845–851. doi: 10.1007/s12272-013-0236-x
- Lee, E. K., Jeong, D. W., Lim, S. J., Gu, G. J., Ahn, S. I., Kim, J. S., … Youn, H. S. (2014). Carpesium abrotanoides extract inhibits inducible nitric oxide synthase expression induced by Toll-like receptor agonists. Food Science and Biotechnology, 23(5), 1637–1641. doi: 10.1007/s10068-014-0223-0
- Moncada, S. (1999). Nitric oxide: Discovery and impact on clinical medicine. Journal of the Royal Society of Medicine, 92(4), 164–169.
- Murakami, A., & Ohigashi, H. (2007). Targeting NOX, INOS and COX-2 in inflammatory cells: Chemoprevention using food phytochemicals. International Journal of Cancer, 121(11), 2357–2363. doi: 10.1002/ijc.23161
- Murakami, A., Takahashi, D., Koshimizu, K., & Ohigashi, H. (2003). Synergistic suppression of superoxide and nitric oxide generation from inflammatory cells by combined food factors. Mutation Research/Fundamental and Molecular Mechanisms of Mutagenesis, 523–524, 151–161. doi: 10.1016/S0027-5107(02)00331-7
- Park, H. J., & Youn, H. S. (2013). Mercury induces the expression of cyclooxygenase-2 and inducible nitric oxide synthase. Toxicology and Industrial Health, 29(2), 169–174. doi: 10.1177/0748233711427048
- Park, M. Y. (2015). Carnosic acid disrupts Toll-like receptor 2 signaling pathway in Pam3CSK4-stimulated macrophages. Toxicology and Environmental Health Sciences, 7(3), 224–230. doi: 10.1007/s13530-015-0242-0
- Rouzer, C. A., & Marnett, L. J. (2009). Cyclooxygenases: Structural and functional insights. The Journal of Lipid Research, 50, S29–S34. doi: 10.1194/jlr.R800042-JLR200
- Shin, H. J., Shon, D. H., & Youn, H. S. (2013). Isobavachalcone suppresses expression of inducible nitric oxide synthase induced by Toll-like receptor agonists. International Immunopharmacology, 15(1), 38–41. doi: 10.1016/j.intimp.2012.11.005
- Sim, J. H., Lee, H. S., Lee, S., Park, D. E., Oh, K., Hwang, K. A., … Kim, H. R. (2014). Anti-asthmatic activities of an ethanol extract of Aster yomena in an ovalbumin-induced murine asthma model. Journal of Medicinal Food, 17(5), 606–611. doi: 10.1089/jmf.2013.2939
- Surh, Y. J., Chun, K. S., Cha, H. H., Han, S. S., Keum, Y. S., Park, K. K., & Lee, S. S. (2001). Molecular mechanisms underlying chemopreventive activities of anti-inflammatory phytochemicals: Down-regulation of COX-2 and iNOS through suppression of NF-kappa B activation. Mutation Research/Fundamental and Molecular Mechanisms of Mutagenesis, 480–481, 243–268. doi: 10.1016/S0027-5107(01)00183-X
- Turini, M. E., & Dubois, R. N. (2002). Cyclooxygenase-2: A therapeutic target. Annual Review of Medicine, 53, 35–57. doi: 10.1146/annurev.med.53.082901.103952
- Vane, J. R. (1971). Inhibition of prostaglandin synthesis as a mechanism of action for aspirin-like drugs. Nature New Biology, 231(25), 232–235. doi: 10.1038/newbio231232a0