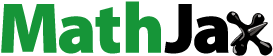
ABSTRACT
Ovotransferrin (OVT) and ovomucoid (OVM) were treated, respectively by different food processing conditions. The protein recovery and immunoreactivity of OVT significantly decreased during heat treatment (P < .05), and aggregates appeared after dry heating at 150°C or 200°C or boiling at 100°C. OVM appeared more stable than OVT under the same treatment. Greatly reduced solubility occurred only after dry heating at 200°C. The immunoreactivity increased after dry heating at 100°C or 150°C or moist heating (60°C, boiling). Autoclave sterilization had a strong effect on the characteristics of both OVT and OVM. Ultra-high pressure had very little effect on OVT and OVM, except at 600 MPa in the moist treatment. Results showed that temperature synergistic processing with water and high pressure had the greatest impact on the characteristics, which could be a potential strategy for mitigating the negative effects of allergens in egg.
Introduction
Eggs compose one of the eight main categories of food allergens and are widely used in the food industry (Acero-Lopez, Ullah, Offengenden, Jung, & Wu, Citation2012). Approximately 0.5–2.5% of children worldwide are allergic to eggs, and two-thirds of children suffer from severe atopic dermatitis caused by egg hypersensitivity (Anet et al., Citation1985). Therefore, eggs are considered the second most common food allergen for infants, with milk being the most common (Bush & Hefle, Citation1996).
Most egg allergens are mainly contained in egg whites (Dubois et al., Citation2015). Ovotransferrin (OVT), which accounts for 12% of the total egg white protein (Fu, Maks, & Banaszewski, Citation2010; Herman, Helm, Jung, & Kinney, Citation2003), is an iron-binding glycoprotein that contains 2.63% carbohydrate (Hirose, Citation2000). Ovomucoid (OVM) is present at the highest concentration and exhibits the strongest allergenicity in egg whites; OVM contains 20–25% sugar, which makes it is fairly stable under treatment with trypsin, and is therefore also known to be a trypsin inhibitor (Kume & Matsuda, Citation1995).
Because of the threat that allergens pose to human health, many scientists have been working on strategies to reduce allergenicity, including physical methods, such as heat processing (Lasekan & Nayak, Citation2016), irritation (Lemon-Mulé et al., Citation2008; Liu et al., Citation2017), and ultra-high pressure (Liang, Xu, Pan, Ge, & Zong, Citation2015); chemical methods, such as the Maillard reaction (Li, Jiang, You, Luo, & Feng, Citation2014; Li, Offengenden, Fentabil, Gänzle, & Wu, Citation2013), and enzymatic hydrolysis (Mine, Citation1995), and biological methods, such as traditional fermentation (Moon & Song, Citation2001), or gene silencing (Kovacs-Nolan, Phillips, & Mine, Citation2005; Nicolai & Durand, Citation2013). These methods have diverse effects on allergens based on different principles, even leading to the formation of new allergenic compounds via the Maillard reaction (Rahaman, Vasiljevic, & Ramchandran, Citation2016; Yu, Goktepe, & Ahmedna, Citation2013). Among all of these strategies, thermal processing has been verified to be a promising tool that can lead to the destruction of the secondary and tertiary structures of proteins, along with disulfide bond cleavage and new disulfide bond formation, thereby masking protein epitomes and reducing the allergenicity of the allergen (Rao et al., Citation2016). Therefore, many studies have investigated the thermal effects on common allergens, primarily the allergens in peanut (Rao et al., Citation2016), soybean protein (Li, Zhu, Zhou, Peng, & Guo, Citation2016), milk (Sampson, Citation2004; Xu, Shi, Yao, Jiang, & Luo, Citation2015), wheat, and almond (Taheri-Kafrani et al., Citation2009), as well as egg OVT (Fu et al., Citation2010; Tong, Gao, Chen, & Li, Citation2012), at different temperatures. Due to their complex conformational structure, different allergens exhibit different changes in their characteristics. Regarding the allergens in egg, Tong et al. investigated the effect of the mild temperature on hen OVT. The OVT structure unfolded when heated to 55–60°C, and the secondary structure disappeared when heating to 70–80°C. Conversely, the allergenicity of OVT was enhanced under these conditions as the protein structure expanded and disulfide bonds fractured (Williams, Elleman, Kingston, Wilkins, & Kuhn, Citation1982).
Ultra-high-pressure treatment, which can reduce the molecule spacing and change the electronic and crystal structures, while maintaining the original nutritional value, colour, and flavour of the food (Rastogi, Raghavarao, Balasubramaniam, Niranjan, & Knorr, Citation2007), has also been used to treat allergens. Liang found a positive effect on pineapple juice, with a reduction in allergenicity that correlated with increasing pressure and a maximum reduction of approximately 20% under 500 MPa (Liang et al., Citation2015). A greater reduction of the major peanut allergens and IgE-binding capacity was induced when using a high-pressure treatment at 500 MPa combined with polyphenol oxidase (Chung, Houska, & Reed, Citation2013). Acero-Lopez et al. (Citation2012) reported that OVT treated with high pressure and different pH levels resulted in changes in the sulfhydryl groups, surface hydrophobicity, enthalpy, and intrinsic fluorescence, along with partial aggregation. Our previous study also showed that high-pressure treatment at 500 MPa with water significantly reduced the solubility and immunoreactivity of almond proteins (Zhang, Zhang, Sheng, Wang, & Fu, Citation2016).
The present study aimed to systematically investigate the effects of general food processing, including heat, autoclave sterilization, and ultra-high pressure, on the solubility, primary structure, and immunoreactivity of OVT and OVM proteins, specifically concerning the combined effect of temperature, water and high pressure.
Materials and methods
Dry heat treatment
OVT (20 mg) or OVM (20 mg) powder (both from Sigma-Aldrich, St. Louis, MO, USA) was weighed into glass tubes or crucibles and heated in an oil bath (Yuhua, He’nan, China) at 100°C or 150°C or in a muffle furnace (Zhonghuan, Tianjin, China) at 200°C for 10 min.
Moist heat treatment
OVT (20 mg) or OVM (20 mg) powder was weighed into tubes, dissolved in 2 mL of PBS, and then heated in an oil bath at 60°C or boiled for 10 min, respectively.
Autoclave sterilization
OVT (20 mg) or OVM (20 mg) powder was weighed into 15-mL centrifuge tubes and autoclaved in an HVA-110 autoclave (Hirayama, Kasukabe, Saitama-ken, Japan) at 121°C and 0.15 MPa for 10 min. The same quantity of each protein was also dissolved in 2 mL of PBS as a moist sample and subjected to autoclave treatment as described above.
High-pressure treatment
OVT (20 mg) or OVM (20 mg) powder was weighed into plastic pouches. Half of the samples were dissolved in 2 mL of PBS and then hot-sealed. The samples were then subjected to high-pressure treatment in an HPP.L2-600/0.6 high-pressure system (Huatai, Tianjin, China) at 400, 500, or 600 MPa of hydrostatic pressure and 20°C for 10 min.
Protein extraction of the treated samples
After treatment, 2 mL of PBS was added to the solid sample, which was then mixed on a shaker (30 rpm/min) for 5 min. Then, the sample was centrifuged at 10,000g for 10 min, and the supernatant was removed for further use.
Determination of the protein recovery
The protein concentration in the supernatant was determined using a bicinchoninic acid (BCA) Protein Assay Kit (Solarbio Scientific Co., Beijing, China), according to the manufacturer’s instructions. The protein recovery was calculated as the ratio of the protein concentration in the treated samples to those in the control sample.
Determination of the immunoreactivity of OVT and OVM by indirect competitive enzyme-linked immunosorbent assay (IC-ELISA)
IC-ELISA was used to evaluate the immunoreactivity changes in the target proteins. The detailed procedure of IC-ELISA for OVT or OVM is as follows.
OVT (0.03 µg) or OVM (0.05 µg) in 100 µL of coating buffer (50 mM carbonate buffer, pH 9.6) was added to each well of a 96-well plate (Thermo Scientific, Rochester, MN, USA), which was then incubated at 4°C for 12–16 h. The plate was then washed with washing buffer (0.05% Tween 20 in PBS solution, pH 7.4, 200 µL/well) for three times. Two hundred microlitres of blocking buffer (0.5% skimmed milk powder in PBS, pH 7.4) was added to each well and incubated at 37°C for 1 h to block the unbound sites. After three washes with washing buffer, 50 µL of PBS was added into the control wells, and 100 µL of PBS was added into the blank wells. Fifty microlitres of serially diluted OVT or OVM samples (quantified by BCA assay kit after treatment) was added into each well (triplicate wells for each diluted sample) except the blank well, followed by 50 µL of diluted rabbit anti-OVT (1:10,000 dilution) or rabbit anti-OVM (1:40,000 dilution) antibodies (generated in-house) in PBS, and then incubated for 1 h at 37°C. After four washes, 100 µL of HRP-labelled goat anti-rabbit IgG (Promega, Madison, WI, USA) diluted at 1:10,000 with PBS was added, and the mixture was incubated at 37°C for 30 min. After three washes, 100 µL of a TMB substrate solution (1.25 mM 3,3′,5,5′-tetramethylbenzidine-1.6 mM hydrogen peroxide in acetate buffer, pH 5.0) was added to each well, and the mixture was incubated for 15–30 min. Finally, 50 µL of a stop solution (1.25 mol L−1 H2SO4) was added into each well.
A microplate reader (Thermo Scientific) recorded the absorbance at 450 nm (test wavelength) and 650 nm (reference wavelength). Inhibition curves were established by graphing the inhibition values versus the concentrations of the tested samples. The inhibition ratio was calculated using the following equation:
where A0 is the absorbance of the control sample, A2 is the absorbance of the tested sample, and A1 is the absorbance of the blank well. The protein concentration that gives 50% inhibition (IC50) was obtained from the OVT or OVM inhibition curve.
Electrophoresis
The protein profiles were analysed by sodium dodecyl sulfate polyacrylamide gel electrophoresis (SDS-PAGE). The supernatants of the treated proteins were diluted to 1 mg/mL and boiled in gel loading buffer (Bio-Rad Laboratories, Richmond, CA, USA) for 5 min. Ten microlitres of the boiled samples were then loaded onto the pre-prepared gel (stacking gel was 5% acrylamide, separating gel was 12% acrylamide; Bio-Rad Laboratories, Richmond, CA, USA) with an electrophoresis buffer (0.025 M Tris/HCl buffer containing 0.1% SDS and 0.192 M glycine). The electrophoresis voltage was constant at 80 V. The gel was stained and destained according to Zhang (Citation2016).
Experimental design and statistical analysis
All the treatments were carried out in three independent experiments. For each trial, the protein extracts were analysed using the BCA assay and immunoassay in triplicate wells. Analysis of variance between the normalized mean values was carried out using Duncan’s test in IBM SPSS (version rel. 19.0, 2010) with a significance level of P < .05.
Statement of human and animal rights
No experiments were related to human or animal rights.
Results and discussion
OVT and OVM were subjected to various conditions that usually occur during home cooking or industrial food manufacturing, including thermal and non-thermal processing. Water was also considered under the same conditions to investigate whether it had any effect on the proteins in question. Water and pressure treatment were also combined with thermal treatment to investigate any potential synergistic effects. The solubility, immunoreactivity, and protein structure were evaluated by determining the protein recovery, immunoassay IC50 and SDS-PAGE profile.
Effect of processing on the solubility of OVT protein
The solubility of a protein is an important indicator of whether the protein is denatured or coagulated. Changes in protein quantity were used to assess changes in the solubility of the treated OVT and OVM proteins. The results were normalized and calculated as the ratio of the values of treated samples to those of untreated samples to avoid data fluctuations between different experiments.
The changes in the solubility of the processed OVT proteins are shown in . After dry heating at 100°C, the relative recovery of OVT significantly decreased (P < .05) compared with the control. The differences between the protein samples processed at 150°C and 200°C were also significant (P < .05); when heated at 200°C, almost all protein was precipitated, and the protein recovery was very low, indicating that heat has a strong effect on OVT.
Table 1. Effect of processing on the solubility of OVT and OVM.
The protein recovery of OVT under moist heat at 60°C was similar to that observed after the dry heat treatment at 100°C. After the boiling water treatment, the relative protein recovery significantly decreased (P < .05), similar to the result obtained after dry heat treatment at 200°C, showing that moist heat has a greater effect on OVT than dry heat.
Autoclaving also exerted a strong effect on the solubility of OVT proteins (). The treated proteins were mostly precipitated and difficult to re-dissolve in PBS. To investigate the precipitated protein, an 8 M urea solution was added, but all the precipitates did not re-dissolve and likely formed higher molecular aggregates via inter-molecular crosslinking. Compared with the moist heat treatment, autoclaving caused a dramatic change in the protein properties, which may have been due to the combined effect of pressure and temperature.
The effect of high pressure on the solubility of OVT protein is also shown in . The protein recovery of OVT from the different pressure treatments was not significantly different from that in the control group (P > .05), except in the treatment with 600 MPa and moist heat, where a small amount of protein coagulated and could not be re-dissolved in PBS. Therefore, ultra-high pressure had a limited effect on the solubility of OVT.
Effect of processing on the solubility of OVM protein
Under dry heat treatment, the trend of the OVM protein recovery was similar to that of OVT; under dry heat at 200°C, the protein precipitated, and very low recovery was obtained (). However, in the moist heat and autoclave treatments, the protein recovery of OVM decreased significantly compared with that of the unprocessed sample (P < .05; ). However, there was no significant difference within the results of these treatments, and higher recoveries were obtained compared with the recoveries of OVT, indicating that OVM is more stable than OVT in moist heat and autoclave treatments.
In the high-pressure treatment, neither dry nor moist pressure could significantly change the solubility of OVM (P > .05; ), indicating that OVM is stable under high pressure.
The relative protein recovery of OVM was always higher than that of OVT; therefore, OVM is more stable than OVT during heat treatment, regardless of the application of dry or moist heat or concurrent autoclaving or ultra-high-pressure treatments.
Effect of processing on the immunoreactivity of OVT
IC-ELISA was used to evaluate the immunoreactivity of the allergens. The change in immunoreactivity after various treatments can be estimated from the IC50 determined from ELISA based on the specific reaction between the OVT or OVM allergen and their cognate antibodies. Structural alteration of the target proteins can change the immunoreactivity, with an increased IC50 representing reduced immunoreactivity. To avoid data fluctuations between different experiments, relative IC50 values were calculated as the ratio of the IC50 of the processed protein to those of unprocessed samples.
The relative IC50 () of OVT was not significantly affected by dry heating at 100°C (P > .05) but obviously increased when heated to 150°C (P < .05), indicating that the immunoreactivity of OVT was significantly reduced. Spatial structural changes in OVT under these conditions may result in some epitopes being destroyed. When heated to 200°C, the IC50 was undetectable, indicating no identified epitopes, which may due to the protein aggregation besides the destroyed epitopes.
Table 2. Effect of food processing on the immunoreactivity of OVT and OVM.
After exposure to moist heat at 60°C or 100°C, the IC50 of OVT significantly increased (P < .05) to an even greater extent than that in the dry heat treatment, indicating that moist heat has a greater effect on immunoreactivity than dry heat. In the autoclaved (121°C, 0.15 MPa) samples, whether treated as a solid or in PBS solution, the protein completely precipitated and could not be re-dissolved in PBS; therefore, no IC50 values could be determined. These results also suggest a synergistic influence of the temperature and water on immunoreactivity, resulting in greater attenuation of immunoreactivity than that observed with heat alone.
In the high-pressure treatment, no obvious differences in the IC50 values of the processed and unprocessed samples were observed, regardless of whether moist or dry pressure was applied (P > .05). These results are inconsistent with those obtained for OVT solubility, which decreased with increasing pressure, even at 600 MPa of moist pressure. High pressure likely did not damage the epitopes of the OVT that remained in the aqueous solution, further verifying that ultra-high pressure has a limited effect on the characteristics of OVT (without considering the undissolved OVT).
Effect of processing on the immunoreactivity of OVM
Processed OVM produced unexpected results with regards to immunoreactivity (). The IC50 values of OVM obviously decreased after exposure to dry heat at 100°C and 150°C and moist heat at 100°C (P < .05), indicating that the immunoreactivity of OVM had significantly increased. Because OVM contain linear epitopes that, when heated within a certain temperature range, become exposed as the spatial structure of OVM decomposes, thus increasing the binding sites for specific antibodies and leading to the observed enhancement in immunoreactivity. Upon heating at 200°C, the immunoreactivity significantly decreased (P < .05) possibly due to the destruction of the primary structure of OVM, including the linear epitopes.
After autoclaving (121°C, 0.15 MPa), different samples showed opposing immunoreactivities (). The IC50 values of solid OVM significantly decreased (P < .05), whereas the IC50 of OVM in PBS solution significantly increased (P < .05), indicating that water plays an important role in the denaturation of OVM protein. Serving as an energy transfer agent, water increases the effect of heat and pressure on the protein, leading to significant changes in the protein structure and thus immunoreactivity.
The immunoreactivity of OVM under high-pressure processing was similar to that observed for OVT, except in the treatment consisting of 600 MPa of moist pressure. In this treatment, the IC50 values of OVT remained unchanged, indicating unchanged epitopes, whereas those of OVM decreased (), indicating increased immunoreactivity. The inconsistent changes in protein solubility under the same processing conditions further verified that OVM possesses linear epitopes. With the synergistic treatment of extremely high pressure and water, the spatial structure of OVM was destroyed, the linear epitopes were exposed, and the immunoreactivity was enhanced.
SDS-PAGE analysis of OVT after the different treatments
The structures of OVT and OVM after the different treatments were evaluated by SDS-PAGE. SDS and β-ME destroy the secondary and tertiary structures of the protein, respectively; therefore, the SDS-PAGE profile reveals changes in the primary structure of proteins.
shows the protein/peptide profiles of OVT following different treatments. Most bands remained unchanged (lanes 1, 2, 4, and 9–14) compared with the untreated sample (lane 15), indicating that, the structure of OVT was not destroyed by treatment with dry heat at 100°C, moist heat at 60°C, or high pressure at 400–600 MPa. Higher molecular weight bands appeared after dry heat treatment at 150°C and 200°C and boiling (lanes 2, 3, and 5); in particular, more protein was observed with dry heating at 200°C, and aggregation was observed with boiling, verifying the hypothesis that protein tends to form aggregates under high temperature. The solubility of the autoclaved proteins was very low, so the extraction had to be concentrated to achieve the same content as in the other samples. However, no band was observed. It was supposed due to the small molecular weight protein or the soluble aggregated proteins, which cannot be invisible in the normal SAS-PAGE.
Figure 1. SDS-PAGE patterns of OVT after different treatments. Lane 1, Dry heat at 100°C; 2, dry heat at 150°C; 3, dry heat at 200°C; 4, moist heat at 60°C; 5, moist heat at 100°C; 6, dry autoclave sterilization; 7, moist autoclave sterilization; 8, marker; 9, dry high-pressure processing at 400 MPa; 10, moist high-pressure processing at 400 MPa; 11, dry high-pressure processing at 500 MPa; 12, moist high-pressure processing at 500 MPa; 13, dry high-pressure processing at 600 MPa; 14, moist high-pressure processing at 600 MPa; 15, untreated sample.
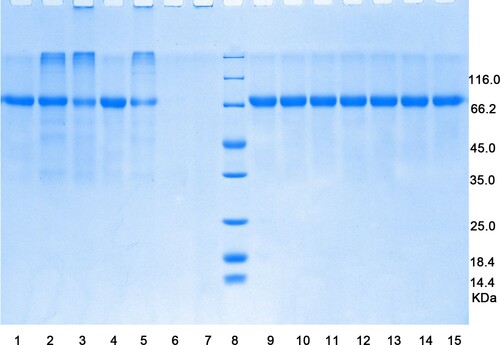
Based on the unchanged primary structure, in combination with the solubility and decreased immunoreactivity after treatment at 100°C, 150°C, and moist 60°C, it may be concluded that the conformational epitopes of OVT had been destroyed, making it impossible to bind with specific antibodies.
SDS-PAGE analysis of OVM after different treatments
shows the SDS-PAGE profiles of OVM after the different treatments. The profiles of OVM determined after dry heating at 200°C (lane 3) and under dry or moist autoclave sterilization (lanes 6 and 7) were obviously different from that of the unprocessed sample (lane 15), which indicates that these treatments can partially or totally destroy the primary structure of OVM protein.
Figure 2. SDS-PAGE patterns of OVM after different treatments. Lane 1, Dry heat at 100°C; 2, dry heat at 150°C; 3, dry heat at 200°C; 4, moist heat at 60°C; 5, moist heat at 100°C; 6, dry autoclave sterilization; 7, moist autoclave sterilization; 8, marker; 9, dry high-pressure processing at 400 MPa; 10, moist high-pressure processing at 400 MPa; 11, dry high-pressure processing at 500 MPa; 12, moist high-pressure processing at 500 MPa; 13, dry high-pressure processing at 600 MPa; 14, moist high-pressure processing at 600 MPa; 15, untreated sample.
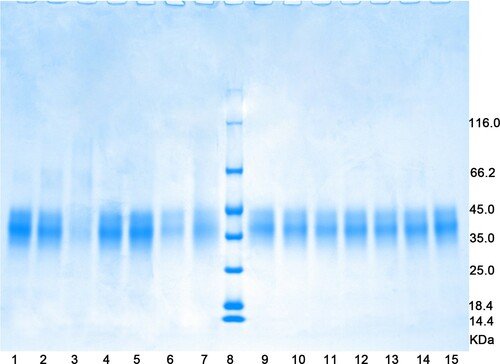
As observed with OVT in the ultra-high-pressure treatments, no significant changes in the SDS-PAGE profiles of OVM were observed after the high-pressure treatment (lanes 9–14), showing that the primary structure of OVM was stable under ultra-high pressure.
Conclusion
Heating, autoclaving, and ultra-high-pressure treatments had different effects on the characteristics of OVT and OVM allergens in egg. Temperature was the key factor that significantly contributed to the changes in immunoreactivity of OVT and OVM proteins, which were induced by the structural alteration. Autoclave sterilization had a substantial effect on the solubility, immunoreactivity, and structure of both OVT and OVM, whereas high pressure exerted a moderate effect that did not alter the primary structures of OVT or OVM, nor the solubility or immunoreactivity significantly. Based on all the determined characteristics of OVT and OVM after these various treatments, OVM was more stable than OVT under the same treatment.
The responses of the solubility, immunoreactivity, and structure of the tested allergens were consistent under the same processing conditions. The protein structure determined the solubility and immunoreactivity of these allergens. Any processing that alters the structure of the protein could alter the immunoreactivity and solubility. Therefore, synergistic processing with temperature, water, and high pressure had the greatest effect on the characteristics of OVT and OVM. This information could be used to mitigate the negative effects of allergens in egg.
Disclosure statement
No potential conflict of interest was reported by the authors.
Additional information
Funding
References
- Acero-Lopez, A., Ullah, A., Offengenden, M., Jung, S., & Wu, J. P. (2012). Effect of high pressure treatment on ovotransferrin. Food Chemistry, 135, 2245–2252. doi: https://doi.org/10.1016/j.foodchem.2012.07.071
- Anet, J., Back, J. F., Baker, R. S., Barnett, D., Burley, R. W., & Howden, M. E. (1985). Allergens in the white and yolk of hen’s egg a study of IgE binding by egg proteins. International Archives of Allergy and Immunology, 77, 364–371. doi: https://doi.org/10.1159/000233846
- Bush, R. K., & Hefle, S. L. (1996). Food allergens. Critical Reviews in Food Science & Nutrition, 36, 119–163. doi: https://doi.org/10.1080/10408399609527762
- Chung, S. Y., Houska, M., & Reed, S. (2013). Reducing peanut allergens by high pressure combined with polyphenol oxidase. High Pressure Research, 33, 813–821. doi: https://doi.org/10.1080/08957959.2013.828716
- Dubois, A. E. J., Pagliarani, G., Brouwer, R. M., Kollen, B. J., Dragsted, L. O., Eriksen, F. D., & Callesen, O. (2015). First successful reduction of clinical allergenicity of food by genetic modification: Mal d 1-silenced apples cause fewer allergy symptoms than the wild-type cultivar. Allergy, 70, 1406–1412. doi: https://doi.org/10.1111/all.12684
- Fu, T. J., Maks, N., & Banaszewski, K. (2010). Effect of heat treatment on the quantitative detection of egg protein residues by commercial enzyme-linked immunosorbent assay test kits. Journal of Agricultural and Food Chemistry, 58, 4831–4838. doi: https://doi.org/10.1021/jf903536z
- Herman, E. M., Helm, R. M., Jung, R., & Kinney, A. J. (2003). Genetic modification removes an immunodominant allergen from soybean. Plant Physiology, 132, 36–43. doi: https://doi.org/10.1104/pp.103.021865
- Hirose, M. (2000). The structural mechanism for iron uptake and release by transferrins. Bioscience, Biotechnology, and Biochemistry, 64, 1328–1336. doi: https://doi.org/10.1271/bbb.64.1328
- Kovacs-Nolan, J., Phillips, A. M., & Mine, Y. (2005). Advances in the value of eggs and egg components for human health. Journal of Agricultural and Food Chemistry, 53, 8421–8431. doi: https://doi.org/10.1021/jf050964f
- Kume, T., & Matsuda, T. (1995). Changes in structural and antigenic properties of proteins by radiation. Radiation Physics and Chemistry, 46, 225–231. doi: https://doi.org/10.1016/0969-806X(95)00017-R
- Lasekan, A. O., & Nayak, B. (2016). Effects of buffer additives and thermal processing methods on the solubility of shrimp (penaeus monodon) proteins and the immunoreactivity of its major allergen. Food Chemistry, 200, 146–153. doi: https://doi.org/10.1016/j.foodchem.2016.01.015
- Lemon-Mulé, H., Sampson, H. A., Sicherer, S. H., Shreffler, W. G., Noone, S., & Wegrzyn, A. N. (2008). Immunologic changes in children with egg allergy ingesting extensively heated egg. Journal of Allergy and Clinical Immunology, 122, 977–983. doi: https://doi.org/10.1016/j.jaci.2008.09.007
- Li, Z., Jiang, M., You, J., Luo, Y., & Feng, L. (2014). Impact of maillard reaction conditions on the antigenicity of parvalbumin, the major allergen in grass carp. Food and Agricultural Immunology, 25(4), 486–497. doi: https://doi.org/10.1080/09540105.2013.838943
- Li, S., Offengenden, M., Fentabil, M., Gänzle, M. G., & Wu, J. (2013). Effect of egg white fermentation with lactobacilli on IgE binding ability of egg white proteins. Food Research International, 52, 359–366. doi: https://doi.org/10.1016/j.foodres.2013.03.018
- Li, H., Zhu, K. X., Zhou, H. M., Peng, W., & Guo, X. N. (2016). Comparative study of four physical approaches about allergenicity of soybean protein isolate for infant formula. Food and Agricultural Immunology, 27(5), 1–20. doi:10.1080/37209540105.2015.1129602 doi: https://doi.org/10.1080/09540105.2015.1129602
- Liang, J., Xu, J. F., Pan, J., Ge, M., & Zong, K. (2015). Identification of the main allergenic proteins in high hydrostatic pressure pineapple juice and assessing the influence of pressure on their allergenicity. International Journal of Food Properties, 18, 2134–2144. doi: https://doi.org/10.1080/10942912.2014.966386
- Liu, Y. X., Li, Z. J., Pavase, T., Li, Z. X., Liu, Y. M., & Wang, N. (2017). Evaluation of electron beam irradiation to reduce the IgE binding capacity of frozen shrimp tropomyosin. Food and Agricultural Immunology, 28, 189–201. doi: https://doi.org/10.1080/09540105.2016.1251394
- Mine, Y. (1995). Recent advances in the understanding of egg-white protein functionality. Trends in Food Science and Technology, 6, 225–232. doi: https://doi.org/10.1016/S0924-2244(00)89083-4
- Moon, S., & Song, K. B. (2001). Effect of γ-irradiation on the molecular properties of ovalbumin and ovomucoid and protection by ascorbic acid. Food Chemistry, 74, 479–483. doi: https://doi.org/10.1016/S0308-8146(01)00166-2
- Nicolai, T., & Durand, D. (2013). Controlled food protein aggregation for new functionality. Current Opinion in Colloid and Interface Science, 18, 249–256. doi: https://doi.org/10.1016/j.cocis.2013.03.001
- Rahaman, T., Vasiljevic, T., & Ramchandran, L. (2016). Effect of processing on conformational changes of food proteins related to allergenicity. Trends in Food Science and Technology, 49, 24–34. doi: https://doi.org/10.1016/j.tifs.2016.01.001
- Rao, H., Tian, Y., Tao, S., Tang, J., Li, X., & Xue, W. T. (2016). Key factors affecting the immunoreactivity of roasted and boiled peanuts: Temperature and water. LWT-food Science and Technology, 72, 492–500. doi: https://doi.org/10.1016/j.lwt.2016.05.014
- Rastogi, N. K., Raghavarao, K. S. M. S., Balasubramaniam, V. M., Niranjan, K., & Knorr, D. (2007). Opportunities and challenges in high pressure processing of foods. Critical Reviews in Food Science and Nutrition, 47, 69–112. doi: https://doi.org/10.1080/10408390600626420
- Sampson, H. A. (2004). Update on food allergy. Journal of Allergy and Clinical Immunology, 113, 805–819. doi: https://doi.org/10.1016/j.jaci.2004.03.014
- Taheri-Kafrani, A., Gaudin, J. C., Rabesona, H., Nioi, C., Agarwal, D., Drouet, M., … Haertle, T. (2009). Effects of heating and glycation of β-lactoglobulin on its recognition by IgE of sera from cow milk allergy patients. Journal of Agricultural and Food Chemistry, 57, 4974–4982. doi: https://doi.org/10.1021/jf804038t
- Tong, P., Gao, J. Y., Chen, H. B., & Li, F. H. (2012). Effect of heat treatment on the potential allergenicity and conformational structure of egg allergen ovotransferrin. Food Chemistry, 131, 603–610. doi: https://doi.org/10.1016/j.foodchem.2011.08.084
- Williams, J., Elleman, T. C., Kingston, I. B., Wilkins, A. G., & Kuhn, K. A. (1982). The primary structure of hen ovotransferrin. European Journal of Biochemistry, 122, 297–303. doi: https://doi.org/10.1111/j.1432-1033.1982.tb05880.x
- Xu, Q., Shi, J., Yao, M., Jiang, M., & Luo, Y. (2015). Effects of heat treatment on the antigenicity of four milk proteins in milk protein concentrates. Food and Agricultural Immunology, 27(3), 1–13. doi: https://doi.org/10.1080/09540105.2015.1117059
- Yu, J. M., Goktepe, I., & Ahmedna, M. (2013). Enzymatic treatment of peanut butter to reduce the concentration of major peanut allergens. International Journal of Food Science and Technology, 48, 1224–1234. doi: https://doi.org/10.1111/ijfs.12081
- Zhang, Y., Zhang, J. Q., Sheng, W., Wang, S., & Fu, T. J. (2016). Effects of heat and high-pressure treatments on the solubility and immunoreactivity of almond proteins. Food Chemistry, 199, 856–861. doi: https://doi.org/10.1016/j.foodchem.2015.12.063