ABSTRACT
The present investigation aims to evaluate the prophylactic effects and mechanisms of Myr on experimental colitis model induced by acetic acid (AA) in mice. The obtained results showed that Myr treatment prior to induction of colitis significantly modulated the colonic oxidative stress by increasing the antioxidant enzymatic activities of catalse, superoxide dismutase, glutathione peroxidase and glutathione reductase, as well as by lowering the levels of lipid peroxidation. The treatment also significantly decreased the colonic tissue levels of inflammatory markers, including myeloperoxidase activity, tumour necrosis factor-alpha, interleukin-1 and interleukin-17. Besides, Myr significantly inhibited nuclear factor-kappa B (NF-κB) levels in AA-induced ulcerative colitis tissues. In the histological evaluation, the tissue damage scores in the mice colon were significantly suppressed with the administration of Myr + AA. Taken together, our findings suggested that Myr exerts marked protective effect against colonic inflammation through regulating antioxidative responses, NF-κB and subsequent pro-inflammatory cytokines production.
Introduction
Ulcerative colitis (UC) is a type of debilitating and autoimmune-mediated inflammatory bowel disease (IBD), which ultimately leads to extensive mucosal disruption and ulceration (Niu et al., Citation2015). Compelling evidences indicate that human subjects with untreated IBD may develop colorectal cancer (Sturm, de Souza, & Fiocchi, Citation2008) and life-threatening respiratory or circulatory complications (Chu, Moran, & Card, Citation2017). Although the full etiology of UC is not completely understood, the current literature suggests that dysregulation of the antioxidant defense system and pro-/anti-inflammatory systems are key events in the pathogenesis of UC (Amirshahrokhi, Citation2019; Kannan & Guruvayoorappan, Citation2013; Sener et al., Citation2007; Talero et al., Citation2008). The nuclear factor kappa B (NF-κB) promotes transcription of genes encoding proinflammatory cytokines, which are directly involved in the mucosal tissue damage occurring in UC (Gu et al., Citation2017). Consequently, the effective inhibition of NF-κB regulated cytokine expression may be beneficial in the treatment of inflammation. Currently, allopathic therapeutic strategies for UC include different lines of drugs like antibiotics, antimicrobials and immunosuppressive agents. However, the risk of causing adverse side effects upon long-term treatment limit their clinical use (Xu, Meng, & Pan, Citation2004). Therefore, there is an increasing need to explore alternative drug candidates (e.g. herbal products) with improved tolerability and safety for the treatment of this disease. Myristicin (Myr) belongs to the methylenedioxyphenyl or allyl-benzene family of compounds, which are found widely in various spices such as nutmeg and mace, and in plants of the Umbelliferae family, such as parsley and carrot (Yang et al., Citation2015). Myr was reported to have diverse biological effects, including antibacterial, antioxidant, and cancer chemopreventive properties (Dkhil et al., Citation2019; Lee & Park, Citation2011; Ozaki, Soedigdo, Wattimena, & Suganda, Citation1989; Zhao et al., Citation2017; Zheng, Kenney, Zhang, & Lam, Citation1992). Furthermore, it has been demonstrated that Myr exerts a significant anti-inflammatory effect through suppression of tumour necrosis factor-alpha production in a model of liver injury in rats (Morita et al., Citation2003). Despite the well-established therapeutic benefits of Myr in several experimental studies, its effect on drugs-induced colitis has not been studied yet. Herein, we investigated for the first time the anti-inflammatory potency of Myr on a murine model of acetic acid (AA)-induced colitis, which mimics acute human intestinal inflammation, and further explored whether its mechanism of action is associated with scavenging of oxygen free radicals.
Materials and methods
Reagents
Except where indicated, all reagents were obtained from Sigma Aldrich (St. Louis, USA).
Animals
Male albino mice weighing 30–35 g were obtained from an animal facility at King Faisal University, Saudi Arabia and were housed in well-ordered environment with standard rodent diet and water provided ad libitium. They were kept in stainless steel cages in an area at 23 ± 2 °C with a relative humidity of 55 ± 5% and a 12 h light/dark cycle. After 2 weeks of acclimatization, the animals were deprived of food 24 h prior to the beginning of the study but allowed access to water throughout. All experimental procedures were conducted according to the prescribed guidelines of a local ethical committee (Ref. No. KFU-REC/2020-02-19).
Induction of ulcerative colitis
Colitis was provoked in mice according to the method of Ghasemi-Pirbaluti, Motaghi, Najafi, and Hosseini (Citation2017) with slight modifications. Briefly, anesthesia was performed using light ether and a solution of 2 ml acid acetic (AA; 3% in normal saline) was instilled into the lumen of the colon using a medical-grade polyethylene tube (external diameter 2 mm) 4.5 cm proximal to the anus verge. The mice were maintained in the head-down position during instillation and for 30 s after instillation to avoid leakage of solution. Control animals received an equivalent volume of saline solution (0.9%) intracolonically.
Experimental design
Mice were randomly divided into four groups (6 animals per group). Group I (control): received normal saline rectecally; Group II (AA): received rectal AA instillation; Group III (Myr): received a single dose of Myr (200 mg/kg) by oral gavage 6 h before rectal saline instillation; Group IV (Myr + AA): received Myr (as group III) 6 h before rectal AA instillation. The dose of Myr was chosen based on a previous work (Morita et al., Citation2003). Eighteen hours after instillation, all animals were euthanized by ether overdose inhalation, and the colon was excised and processed for all analyses. The proximal colonic segments were immediately stored at −80 °C for further biochemical estimations. The remaining distal portion of colon was used for evaluation of histological lesions.
Biochemical assays
Catalase (CAT), superoxide dismutase (SOD), glutathione peroxidase (GPx) and glutathione reductase (GR) enzyme activities, and malondialdehyde (MDA) levels were measured according to the manufacturer’s protocol (CAT, CAT. No.: CA 2516; SOD, CAT. No.: SD 2520; GPx; CAT. No.: GP 2524; GR, CAT. No.: GR 2522; MDA, CAT No.: MD 2528; Biodiagnostic, Dokki, Egypt). CAT assay colorimetric method depends on the measurement of hydrogen peroxide (H2O2) substrate using a redox dye. The change in colour intensity at 570 nm is directly proportional to the CAT activity. SOD assay relies on the ability of the SOD to inhibit the phenazine methosulphate-mediated reduction of nitro-blue tetrazolium dye. GPx activity was determined by an indirect measure of the activity c-GPx. Oxidized glutathione (GSSG), produced upon reduction of an organic peroxide by c-GPx, is recycled to its reduced state by glutathione reductase (GR). The oxidation of NADPH to NADP+ was accompanied by a decrease in absorbance at 340 nm, thus providing a spectrophotometric means for monitoring GPx enzyme activity. GR was assayed using GSSG as a substrate. GR catalyzed the reduction of GSSG in the presence of NADPH and the decrease in absorbance due to the decreased concentration of NADPH was measured at 340 nm. The level of lipid peroxidation (LPO) was determined according to the concentration of thiobarbituric acid reactive substances (Ohkawa, Ohishi, & Yagi, Citation1979), and the amount of malondialdehyde (MDA) produced was used as an index of LPO. The level of MDA at 534 nm was expressed as nmol/g tissue. Myeloperoxidase (MPO), tumour necrosis factor-alpha (TNF-α), interleukin-1 (IL-1), interleukin-17 (IL-17) and nuclear factor-kappa B (NF-κB) were estimated by Enzyme Linked Immuno-Sorbent Assay (ELISA) kits (MPO, CAT. No.: MBS700747; TNF-α, CAT. No.: MBS2502004; IL-1, CAT. No.: MBS264984; IL-17, CAT. No.: MBS764076; NF-κB, CAT. No.: MBS2023542; MyBioSource, Inc., San Diego, USA). The concentration of the protein was determined using the method of Bradford (Bradford, Citation1976) and crystalline bovine serum albumin was used as a standard protein.
Histopathological study
Fresh colon tissues were fixed in 10% buffered formalin at room temperature for 24 h, dehydrated with increasing concentrations of ethanol, and embedded in paraffin. Thin tissue sections (6 μm) were mounted on slides, cleared, hydrated, stained with hematoxylin and eosin dye, and examined using a Nikon 80i light microscope (Nikon Corporation, Tokyo, Japan). The histological scoring was assessed according to previously described criteria (Banerjee, Ghosh, Sinha, Chowdhury, & Sil, Citation2019) as follows: 0, no evidence of inflammation; 1, low level of inflammation with scattered infiltrating mononuclear cells (1–2 foci); 2, moderate inflammation with multiple foci; 3, high level of inflammation with increased vascular density and marked thickening of colon wall; 4, maximal severity of inflammation with transmural leukocyte infiltration and loss of goblet cells.
Statistics
All data were expressed as means ± SE (standard error). Statistical calculations were performed using SPSS for Windows (version 17; SPSS Inc., Chicago, IL, USA). The results were analysed by one-way ANOVA followed by a LSD post hoc test for multiple comparisons. A P value < 0.05 was considered significant.
Results
Myr reduced cellular oxidative stress induced by AA
In the AA group, the colonic antioxidant enzyme activities of SOD (A), CAT (B), GPx (C) and GR (D) were significantly decreased, while MDA level, a pro-oxidative product (E) was significantly increased compared to the control values. These redox perturbations in the colonic tissue were obviously ameliorated by the pretreatment with Myr at the dose protocol of 200 mg/kg b.w.
Myr alleviated inflammation in the AA-induced colitis
The MPO activity (A) and the levels of TNF-α (B), IL-1 (C) and IL-17 (D) in the colon tissues were significantly increased in the AA group compared to the control. The mice pretreated with Myr at 200 mg/kg b.w. presented a significant decrease in the levels of these inflammatory mediators when compared to the AA group. As NF-κB plays a critical role in inflammation, we then examined the effect of Myr on its activation in the inflamed tissues. The NF-κB level was significantly elevated in the AA group, but reversed in the Myr + AA group (E).
Figure 2. Inflammatory markers and NF-κB protein levels in male mice after treatment of AA and/or Myr. (A) MPO, (B) TNF-α, (C) IL-1, (D) IL-17 and (E) NF-κB. Data are expressed as means ± SE of n = 6 animals/group. Bars with different letters show statistically significant differences between the groups (p < 0.05).
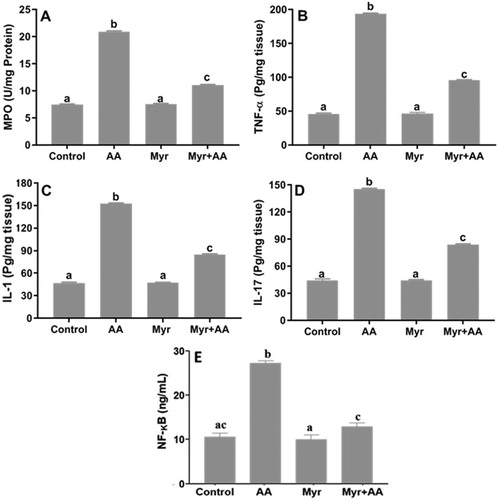
Myr relieved the histopathological colonic damage induced by AA
Microscopic examination of hematoxylin and eosin stained sections of colonic tissues of normal mice showed intact epithelium and mucosal layer (A). In contrast, AA-induced UC promoted necrotic destruction of surface epithelium, focal crypt distortions and abscesses, intense cellular infiltration, mucodepletion, and hyperemia of submucosal capillaries (B). Myr-alone (standard drug) group showed normal mucosal epithelial cells with no observable colonic lesions (C). Noticeably, treatment with Myr + AA showed remarkable recovery of the colonic tissue compared to the AA-induced colitis damage (D). In this group, we observed minimal ulcerous areas and a lesser degree of the inflammatory infiltrates. Also, goblets cells and epithelial lining were preserved to near normal morphology. The histopathological scores confirmed a significantly higher inflammation rate and tissue damage in the colon of AA treated mice relative to those of the healthy control and Mry groups, while pretreatment with Myr (200 mg/kg) decreased the microscopic injury score (by 40%) compared with the AA group (E).
Figure 3. (A-D) Histological findings of colons: (A) Control group showing occasional leucocytes and normal epithelium. (B) AA-induced colitis depicting widespread mucosal damage and mucodepletion. Note also crypt abscess (short arrow), marked inflammatory cell infiltration (long arrows) and massive submucosal congestion (arrowheads). (C) Mry-alone treated group with close appearance to control. (D) Myr pretreatment in AA-treated mice attenuated the extent of severity of colonic cell damage. Original Magnification: 200x (A, B, D) and 100x (C). (E) Scoring of histology sections. Six animals were taken in each group. Results are expressed as mean ± SE. Bars with different letters show statistically significant differences between the groups (p < 0.05).
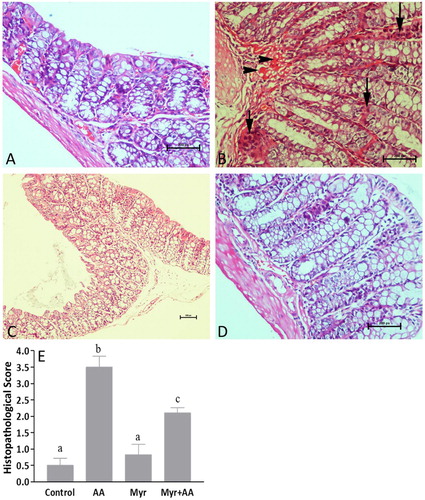
Discussion
Many in vivo inducible models of UC have been produced in order to investigate the pathogenesis and therapeutic opportunities for this disease. In this study, oxidative stress and antioxidant activity parameters, MPO, TNF- α, IL-1, IL-6, and IL-17 levels were analysed to demonstrate the protective effects of Myr in AA-induced experimental colitis, and the tissue histopathologies were also evaluated by light microscopy. According to the literature review, this is the first study demonstrating the protective effect of Myr on UC. We have shown that Myr significantly improved histological scores of colitis severity and diminished the elevated amounts of biochemical markers including MPO and MDA as well as those of inflammatory cytokines.
MPO is a peroxidase enzyme most abundantly found in the azurophilic granules of neutrophils (Klebanoff, Citation2005). High MPO activity is a hallmark of intense infiltration of neutrophils, which may contribute to significant tissue damage (Ozsoy et al., Citation2017). In our study, Myr + AA-treated mice showed a significant decrease of colonic MPO activity when compared to the AA-alone treatment, suggesting an anti-inflammatory effect of Myr. Based on accumulating evidence, the activated and infiltrated neutrophils represents an important source of free radicals in the inflamed colonic mucosa (Daneshmand et al., Citation2009; Isik et al., Citation2011; Niu et al., Citation2013; Suzuki et al., Citation2001; Wera, Lancellotti, & Oury, Citation2016; Williams & Parkos, Citation2007). In fact, the neutrophil-MPO enzyme uses hydrogen peroxide (H2O2) to catalyze the production of the potent oxidants such as hypochlorous acid (HOCl), hypobromous acid (HOBr) and hypothiocyanous acid (HOSCN) from halide and pseudohalide (SCN ̶) ions (Koelsch et al., Citation2010). Excess MPO-derived oxidants react with intestinal macromolecules, including membrane phospholipids to elicit harmful events like LPO (oxidative insult) and finally cause cell death (Bitiren et al., Citation2010; Isik et al., Citation2011; Wong, Yu, & Cho, Citation2008). During experimental colitis, the oxidants play a critical role in the inflammatory reaction by increasing the number of neutrophils and macrophages that induce a self-sustaining loop (Kurutas, Cetinkaya, Bulbuloglu, & Kantarceken, Citation2005; van der Veen, de Winther, & Heeringa, Citation2009). Endogenous antioxidants degrade highly reactive oxygen species (ROS), thus become important targets for IBD treatment (Guan & Lan, Citation2018). Herein, we detected lipid peroxide (i.e. MDA), CAT, SOD, GPx and GR levels in tissues to evaluate the effect of Myr on oxidative stress in mice with acute colitis. Interestingly, we observed a significant decrease in the colon MDA level, but the activities of the antioxidant enzymes of CAT, SOD, GPx and GR were increased by pretreatment with Myr. These results nicely agreed with the previously reported in vitro free radical scavenging activity of Myr-rich extract from nutmeg (Kareem, Gadhamsetty, Shaik, Prasad, & Kodidhela, Citation2013), and the same has been proven with pure Myr isolate in in vivo animal model of spinal cord injury (Zhao et al., Citation2017).
According to published reports, the increased production of proinflammatory mediators such as TNF-α and interleukins (e.g. IL-1 and IL-17) led to inflammation cascade effects and tissue damage in the pathologic progression of colitis (Fujino et al., Citation2003; Gu et al., Citation2017; Impellizzeri et al., Citation2018; Palla, Iqbal, Minhas, & Gilani, Citation2016; Zhu, Gu, & Shen, Citation2019). Particularly, TNF-α is thought to play an essential role in inflammatory diseases such as IBD (UC and CD) and it can activate monocytes, macrophages and intestinal immune cells and then evoke immune responses by triggering secretion of other cytokines, leading to a stronger inflammation (Xiao, Yan, Cao, Yan, & Cai, Citation2016). As expected, TNF-α, IL-1 and IL-17 were significantly raised in colon tissues of AA-treated mice. Myr alone had no effect on those levels, but Myr pretreatment of AA-induced UC drastically attenuated them, which suggested that Myr could protect against AA-induced acute UC at least partly due to its inhibition on the inflammatory factors. There are quite convincing findings that demonstrate the anti-inflammatory effects of Myr. Lee and Park (Citation2011) revealed that Myr suppressed various participants of the immune response, including the production of intracellular calcium, NO, IL-6, IL-10, interferon inducible protein-10, monocyte chemotactic protein (MCP)-1, MCP-3, granulocyte-macrophage colony-stimulating factor (GM-CSF), macrophage inflammatory protein (MIP)-1α, MIP-1β, and leukemia inhibitory factor (LIF, a member of IL-6 family) in double-stranded RNA-stimulated RAW 264.7 mouse macrophages. In addition, Lim, Woo, Lee, Lee, and Kim (Citation2014) showed that Myr could protect against lung inflammation via a concentration-dependent inhibition of IL-1β and IL-6 production from alveolar epithelial cells (A549).
It is known that NF-κB is a central mediator of pro-inflammatory gene induction (Liu, Zhang, Joo, & Sun, Citation2017). Furthermore, it has been reported that NF-κB pathway play a critical role in AA-induced UC mice model (Niu et al., Citation2013, Citation2015). Indeed, many agents exhibit their anti-inflammatory action through suppressing the NF-κB signalling pathways (Kundu & Surh, Citation2004). Our results showed that the NF-κB level was significantly enhanced in response to AA stimulation, while administration of Myr markedly downregulated the increase induced by AA. NF-κB is usually found in the cytoplasm conjugated with inhibitory protein IκB. Under stress-induced inflammatory conditions, the phosphorylation of IκB by IκB kinase leads to degradation of IκB via proteasomes and results in activation and transfer of NF-κB to the nucleus. Thus, we assumed that the pretreatment of Myr could prevent degradation of IκB, thereby inhibiting NF-κB-dependent inflammatory gene expression, which resulted in amelioration of AA-induced colitis. Otherwise, the anti-inflammatory ability of Mry may be achieved indirectly via combating ROS-induced cellular injury. Overproduction of ROS overwhelms antioxidant capacity and activates redox-sensitive NF-κB, resulting in increased level of circulating proinflammatory cytokines (Janssen-Heininger, Poynter, & Baeuerle, Citation2000). Conversely, the pro-inflammatory cytokines cause oxidative stress by promoting the release of ROS from immune and non-immune cells (Reifen, Nissenkorn, Matas, & Bujanover, Citation2004). Thus, uncontrolled inflammation and oxidative stress are involved in the spiralling vicious cycle that contributes to the severity of intestinal inflammation (Toblli, Cao, & Angerosa, Citation2015). Therefore, it is not surprising that natural antioxidant drugs (like Myr) inhibited NF-κB activation.
In conclusion, our data suggest that Myr is a valuable medicinal food for combating colonic inflammation in AA-induced acute colitis, and its effects may be mediated at least partly by the inhibition of NF-κB through scavenging reactions of free radicals.
Acknowledgements
The authors acknowledge the Deanship of Scientific Research at King Faisal University, Saudi Arabia for the financial support under Nasher Track (grant number 186199).
Disclosure statement
No potential conflict of interest was reported by the author(s).
Correction Statement
This article has been republished with minor changes. These changes do not impact the academic content of the article.
Additional information
Funding
References
- Amirshahrokhi, K. (2019). Febuxostat attenuates ulcerative colitis by the inhibition of NF-κB, proinflammatory cytokines, and oxidative stress in mice. International Immunopharmacology, 76, 105884. doi: 10.1016/j.intimp.2019.105884
- Banerjee, S., Ghosh, S., Sinha, K., Chowdhury, S., & Sil, P. C. (2019). Sulphur dioxide ameliorates colitis related pathophysiology and inflammation. Toxicology, 412, 63–78. doi: 10.1016/j.tox.2018.11.010
- Bitiren, M., Karakilcik, A. Z., Zerin, M., Ozardali, I., Selek, S., Nazligul, Y., … Uzunkoy, A. (2010). Protective effects of selenium and vitamin E combination on experimental colitis in blood plasma and colon of rats. Biological Trace Element Research, 136(1), 87–95. doi: 10.1007/s12011-009-8518-3
- Bradford, M. M. (1976). A rapid and sensitive method for the quantitation of microgram quantities of protein utilizing the principle of protein-dye binding. Analytical Biochemistry, 72, 248–254. doi: 10.1016/0003-2697(76)90527-3
- Chu, T. P. C., Moran, G. W., & Card, T. R. (2017). The Pattern of Underlying cause of death in Patients with inflammatory bowel disease in England: A Record Linkage study. Journal of Crohn's & Colitis, 11(5), 578–585.
- Daneshmand, A., Rahimian, R., Mohammadi, H., Ejtemaee-Mehr, S., Tavangar, S. M., Babaei Kelishomi, R., & Dehpour, A. R. (2009). Protective effects of lithium on acetic acid-induced colitis in rats. Digestive Diseases and Sciences, 54(9), 1901–1907. doi: 10.1007/s10620-008-0569-3
- Dkhil, M. A., Abdel Moneim, A. E., Hafez, T. A., Mubaraki, M. A., Mohamed, W. F., Thagfan, F. A., & Al-Quraishy, S. (2019). Myristica fragrans Kernels prevent Paracetamol-induced Hepatotoxicity by Inducing anti-Apoptotic genes and Nrf2/HO-1 pathway. International Journal of Molecular Sciences, 20(4). doi: 10.3390/ijms20040993
- Fujino, S., Andoh, A., Bamba, S., Ogawa, A., Hata, K., Araki, Y., … Fujiyama, Y. (2003). Increased expression of interleukin 17 in inflammatory bowel disease. Gut, 52(1), 65–70. doi: 10.1136/gut.52.1.65
- Ghasemi-Pirbaluti, M., Motaghi, E., Najafi, A., & Hosseini, M. J. (2017). The effect of theophylline on acetic acid induced ulcerative colitis in rats. Biomedicine & Pharmacotherapy, 90, 153–159. doi: 10.1016/j.biopha.2017.03.038
- Gu, P., Zhu, L., Liu, Y., Zhang, L., Liu, J., & Shen, H. (2017). Protective effects of paeoniflorin on TNBS-induced ulcerative colitis through inhibiting NF-kappaB pathway and apoptosis in mice. International Immunopharmacology, 50, 152–160. doi: 10.1016/j.intimp.2017.06.022
- Guan, G., & Lan, S. (2018). Implications of antioxidant systems in inflammatory bowel disease. Biomedicine Research International, 2018, 1. doi: 10.1155/2018/1290179
- Impellizzeri, D., Siracusa, R., Cordaro, M., Peritore, A. F., Gugliandolo, E., Mancuso, G., … Cuzzocrea, S. (2018). Therapeutic potential of dinitrobenzene sulfonic acid (DNBS)-induced colitis in mice by targeting IL-1beta and IL-18. Biochemical Pharmacology, 155, 150–161. doi: 10.1016/j.bcp.2018.06.029
- Isik, F., Tunali Akbay, T., Yarat, A., Genc, Z., Pisiriciler, R., Caliskan-Ak, E., … Sener, G. (2011). Protective effects of black cumin (Nigella sativa) oil on TNBS-induced experimental colitis in rats. Digestive Diseases and Sciences, 56(3), 721–730. doi: 10.1007/s10620-010-1333-z
- Janssen-Heininger, Y. M., Poynter, M. E., & Baeuerle, P. A. (2000). Recent advances towards understanding redox mechanisms in the activation of nuclear factor kappaB. Free Radical Biology and Medicine, 28(9), 1317–1327. doi: 10.1016/S0891-5849(00)00218-5
- Kannan, N., & Guruvayoorappan, C. (2013). Protective effect of Bauhinia tomentosa on acetic acid induced ulcerative colitis by regulating antioxidant and inflammatory mediators. International Immunopharmacology, 16(1), 57–66. doi: 10.1016/j.intimp.2013.03.008
- Kareem, M. A., Gadhamsetty, S. K., Shaik, A. H., Prasad, E. M., & Kodidhela, L. D. (2013). Protective effect of nutmeg aqueous extract against experimentally-induced hepatotoxicity and oxidative stress in rats. Journal of Ayurveda and Integrative Medicine, 4(4), 216–223. doi: 10.4103/0975-9476.123704
- Klebanoff, S. J. (2005). Myeloperoxidase: Friend and foe. Journal of Leukocyte Biology, 77(5), 598–625. doi: 10.1189/jlb.1204697
- Koelsch, M., Mallak, R., Graham, G. G., Kajer, T., Milligan, M. K., Nguyen, L. Q., … Davies, M. J. (2010). Acetaminophen (paracetamol) inhibits myeloperoxidase-catalyzed oxidant production and biological damage at therapeutically achievable concentrations. Biochemical Pharmacology, 79(8), 1156–1164. doi: 10.1016/j.bcp.2009.11.024
- Kundu, J. K., & Surh, Y. J. (2004). Molecular basis of chemoprevention by resveratrol: NF-κB and AP-1 as potential targets. Mutation Research/Fundamental and Molecular Mechanisms of Mutagenesis, 555(1–2), 65–80. doi: 10.1016/j.mrfmmm.2004.05.019
- Kurutas, E. B., Cetinkaya, A., Bulbuloglu, E., & Kantarceken, B. (2005). Effects of antioxidant therapy on leukocyte myeloperoxidase and Cu/Zn-superoxide dismutase and plasma malondialdehyde levels in experimental colitis. Mediators of Inflammation, 2005(6), 390–394. doi: 10.1155/MI.2005.390
- Lee, J. Y., & Park, W. (2011). Anti-inflammatory effect of myristicin on RAW 264.7 macrophages stimulated with polyinosinic-polycytidylic acid. Molecules, 16(8), 7132–7142. doi: 10.3390/molecules16087132
- Lim, H. J., Woo, K. W., Lee, K. R., Lee, S. K., & Kim, H. P. (2014). Inhibition of proinflammatory cytokine Generation in lung inflammation by the Leaves of Perilla frutescens and Its Constituents. Biomolecules & Therapeutics, 22(1), 62–67. doi: 10.4062/biomolther.2013.088
- Liu, T., Zhang, L., Joo, D., & Sun, S.-C. (2017). NF-κB signaling in inflammation. Signal Transduct Target Ther, 2(17023).
- Morita, T., Jinno, K., Kawagishi, H., Arimoto, Y., Suganuma, H., Inakuma, T., & Sugiyama, K. (2003). Hepatoprotective effect of myristicin from nutmeg (Myristica fragrans) on lipopolysaccharide/d-galactosamine-induced liver injury. Journal of Agricultural and Food Chemistry, 51(6), 1560–1565. doi: 10.1021/jf020946n
- Niu, X., Fan, T., Li, W., Huang, H., Zhang, Y., & Xing, W. (2013). Protective effect of sanguinarine against acetic acid-induced ulcerative colitis in mice. Toxicology and Applied Pharmacology, 267(3), 256–265. doi: 10.1016/j.taap.2013.01.009
- Niu, X., Zhang, H., Li, W., Wang, Y., Mu, Q., Wang, X., … Yao, H. (2015). Protective effect of cavidine on acetic acid-induced murine colitis via regulating antioxidant, cytokine profile and NF-κB signal transduction pathways. Chemico-Biological Interactions, 239, 34–45. doi: 10.1016/j.cbi.2015.06.026
- Ohkawa, H., Ohishi, N., & Yagi, K. (1979). Assay for lipid peroxides in animal tissues by thiobarbituric acid reaction. Analytical Biochemistry, 95(2), 351–358. doi: 10.1016/0003-2697(79)90738-3
- Ozaki, Y., Soedigdo, S., Wattimena, Y. R., & Suganda, A. G. (1989). Antiinflammatory effect of mace, aril of Myristica fragrans Houtt., and its active principles. The Japanese Journal of Pharmacology, 49(2), 155–163.
- Ozsoy, Z., Ozsoy, S., Gevrek, F., Demir, E., Benli, I., Daldal, E., … Yenidogan, E. (2017). Effect of bevacizumab on acetic acid-induced ulcerative colitis in rats. Journal of Surgical Research, 216, 191–200. doi: 10.1016/j.jss.2017.05.011
- Palla, A. H., Iqbal, N. T., Minhas, K., & Gilani, A. H. (2016). Flaxseed extract exhibits mucosal protective effect in acetic acid induced colitis in mice by modulating cytokines, antioxidant and antiinflammatory mechanisms. International Immunopharmacology, 38, 153–166. doi: 10.1016/j.intimp.2016.04.043
- Reifen, R., Nissenkorn, A., Matas, Z., & Bujanover, Y. (2004). 5-ASA and lycopene decrease the oxidative stress and inflammation induced by iron in rats with colitis. Journal of Gastroenterology, 39(6), 514–519. doi: 10.1007/s00535-003-1336-z
- Sener, G., Aksoy, H., Sehirli, O., Yuksel, M., Aral, C., Gedik, N., … Yeğen, B. Ç. (2007). Erdosteine prevents colonic inflammation through its antioxidant and free radical scavenging activities. Digestive Diseases and Sciences, 52(9), 2122–2132. doi: 10.1007/s10620-007-9801-9
- Sturm, A., de Souza, H. S., & Fiocchi, C. (2008). Mucosal T cell proliferation and apoptosis in inflammatory bowel disease. Current Drug Targets, 9(5), 381–387. doi: 10.2174/138945008784221198
- Suzuki, K., Sugimura, K., Hasegawa, K., Yoshida, K., Suzuki, A., Ishizuka, K., … Asakura, K. (2001). Activated platelets in ulcerative colitis enhance the production of reactive oxygen species by polymorphonuclear leukocytes. Scandinavian Journal of Gastroenterology, 36(12), 1301–1306. doi: 10.1080/003655201317097164
- Talero, E., Sanchez-Fidalgo, S., de la Lastra, C. A., Illanes, M., Calvo, J. R., & Motilva, V. (2008). Acute and chronic responses associated with adrenomedullin administration in experimental colitis. Peptides, 29(11), 2001–2012. doi: 10.1016/j.peptides.2008.07.013
- Toblli, J. E., Cao, G., & Angerosa, M. (2015). Ferrous sulfate, but not iron polymaltose complex, aggravates local and systemic inflammation and oxidative stress in dextran sodium sulfate-induced colitis in rats. Drug Design, Development and Therapy, 9, 2585–2597. doi: 10.2147/DDDT.S81863
- van der Veen, B. S., de Winther, M. P., & Heeringa, P. (2009). Myeloperoxidase: Molecular mechanisms of action and their relevance to human health and disease. Antioxidants & Redox Signaling, 11(11), 2899–2937. doi: 10.1089/ars.2009.2538
- Wera, O., Lancellotti, P., & Oury, C. (2016). The dual role of neutrophils in inflammatory bowel diseases. Journal of Clinical Medicine, 5(12). doi: 10.3390/jcm5120118
- Williams, I. R., & Parkos, C. A. (2007). Colonic neutrophils in inflammatory bowel disease: Double-edged swords of the innate immune system with protective and destructive capacity. Gastroenterology, 133(6), 2049–2052. doi: 10.1053/j.gastro.2007.10.031
- Wong, V. K., Yu, L., & Cho, C. H. (2008). Protective effect of polysaccharides from Angelica sinensis on ulcerative colitis in rats. Inflammopharmacology, 16(4), 162–167. doi: 10.1007/s10787-007-0026-5
- Xiao, Y. T., Yan, W. H., Cao, Y., Yan, J. K., & Cai, W. (2016). Neutralization of IL-6 and TNF-alpha ameliorates intestinal permeability in DSS-induced colitis. Cytokine, 83, 189–192. doi: 10.1016/j.cyto.2016.04.012
- Xu, C. T., Meng, S. Y., & Pan, B. R. (2004). Drug therapy for ulcerative colitis. World Journal of Gastroenterology, 10(16), 2311–2317. doi: 10.3748/wjg.v10.i16.2311
- Yang, A. H., He, X., Chen, J. X., He, L. N., Jin, C. H., Wang, L. L., … An, L.-J. (2015). Identification and characterization of reactive metabolites in myristicin-mediated mechanism-based inhibition of CYP1A2. Chemico-biological Interactions, 237, 133–140. doi: 10.1016/j.cbi.2015.06.018
- Zhao, Q., Liu, C., Shen, X., Xiao, L., Wang, H., Liu, P., … Xu, H. (2017). Cytoprotective effects of myristicin against hypoxia induced apoptosis and endoplasmic reticulum stress in rat dorsal root ganglion neurons. Molecular Medicine Reports, 15(4), 2280–2288. doi: 10.3892/mmr.2017.6258
- Zheng, G. Q., Kenney, P. M., Zhang, J., & Lam, L. K. (1992). Inhibition of benzo[a]pyrene-induced tumorigenesis by myristicin, a volatile aroma constituent of parsley leaf oil. Carcinogenesis, 13(10), 1921–1923. doi: 10.1093/carcin/13.10.1921
- Zhu, L., Gu, P., & Shen, H. (2019). Protective effects of berberine hydrochloride on DSS-induced ulcerative colitis in rats. International Immunopharmacology, 68, 242–251. doi: 10.1016/j.intimp.2018.12.036