ABSTRACT
Flammulina velutipes contains bioactive polysaccharides that can activate the immune system. Here we report the functional characterization in an immunomodulatory F. velutipes polysaccharide (FVP) both in vitro and in vivo. In cell experiment, FVP was discovered to be an activator to induce the M1-polarization of the RAW 264.7 cells. In animal experiment, FVP was found to raise the gene expression of IL-1β, iNOS and ZO-1 in mice colon tissue. Moreover, it seemed to achieve the immune regulative function by raising the relative abundance of Allobaculum, Lactobacillus, Alloprevotella, Akkermansia and Bifidobacterium. Gut microbiota also caused the body’s functional changes, enriched the harmful chemicals’ degradation ability and weakened the metabolism of carbohydrates. These findings suggest that FVP is an immunomodulatory polysaccharide that can boost cellular immune responses and a potential prebiotic agent in regulating gut microbiota.
1. Introduction
The body’s immune function, primarily initiated by the innate and acquired immune systems, such a body defence mechanism is achieved by immune organs or cells and concatenated by blood or lymph circulation. Against the invasion of exogenous pathogen and harmful chemicals, to monitor and clear cells with the pathological change, the immune system produces a series of protection measures, which is collectively known as immune response (Fan et al., Citation2016). As essential immune cells of the innate immune system in mammals, the mouse leukaemia cells of monocyte macrophage (RAW264.7) participate in the host immune response, including non-specific phagocytize of pathogenic microorganisms, with an antigen presentation function to initiate immune response (Tran Van & Suzuki, Citation2016). The RAW264.7 cell model is commonly used to assess the immune regulatory function of natural products in vitro (Abarikwu, Citation2014). Phenotypic variability is an important characteristic of monocyte macrophages, a different antigen stimulation causes RAW264.7 cells polarized into different subtypes, classically activated macrophages (M1 subtypes) or alternatively activated macrophages (M2 subtypes) (Liu et al., Citation2016). M1 subtypes activated by interferon or lipopolysaccharide, with the strong function of sterilization, produce pro-inflammatory cytokines including tumour necrosis factors-α, interleukins-1β, interleukins-6 and nitric oxide (NO), the pro-inflammatory cytokines form an inflammatory microenvironment, increase the iNOS expression and promote the progress of the inflammatory response (Qin et al., Citation2012). M2 subtypes, activated by interleukins-13, produce arginase, chitinase, interleukins-8 and interleukins-10, with the function of anti-infection, promote the tissue repair process (van Tits et al., Citation2011). The mutual conversion of M1 and M2 subtypes, which regulate the changes of the microenvironment precisely play a key role in the prognosis and outcome of the disease.
Intestinal microbiota is an extreme complex system colonized in the host’s colon, known as the body’s largest “immune organ”. The colonic immune regulatory cells suppress the colonic immune response, making the colonic tolerance to intestinal microbes; hence, the intestinal microbes could make a coexistence with the host (James et al., Citation2020). Intestinal microbiota affecting the host goes beyond the gut tract and impacts the entire immune system, mainly manifested into two aspects: promotion and regulation. Goblet cells stimulated by intestinal microbes in secreting mucus ensure the integrity of the mucosal layer, induce the development of mucosa-associated lymphoid tissues, activate signalling pathways, generate different cytokines, regulate T-cell differentiation to make a balance of bacterial tolerance and immunity (Ohnmacht et al., Citation2011; Wang et al., Citation2012). In addition to this, the bacterial metabolites also translocate into the intestinal lamina propria and produce different immune regulatory effects (Blacher et al., Citation2017; Gao et al., Citation2017).
Nature product polysaccharides from edible mushrooms have the potentiality in activating and regulating the immune system, commonly used as an immunomodulator in clinical therapy (Li et al., Citation2009). The immunomodulatory properties of edible mushrooms, polysaccharides, commonly involved in both innate immunity and acquired immunity (Navegantes et al., Citation2013). The activation and regulation effect on the immune system arise from various mushroom polysaccharides received extensive attentions and research studies (Meng et al., Citation2016). Like most nature products, mushroom polysaccharides have a two-way regulatory effect on the host immune system, either enhance the immunity of normal organisms or improve the inflammation symptoms of inflammatory organisms (Chen et al., Citation2019; Taofiq et al., Citation2016). The immune boosting activity of mushroom polysaccharides appeared to be mediated through the macrophage stimulation (Zong et al., Citation2012). Flammulina velutipes (F. velutipes), a widely consumed edible mushroom in East Asia, has a long history of cultivation and high rate of industrialized production in China. Studies have shown that F. velutipes polysaccharide has a significant immune-enhancing activity in increasing the secretion of nitric oxide (NO), tumour necrosis factor-α, interleukin-6 and interleukin-12 in macrophages (Zhang et al., Citation2018). Meanwhile, it also has a capacity in promoting lymphocyte proliferation (Wu et al., Citation2014). Another research reported in our laboratory demonstrated that after chelated with zinc, F. velutipes polysaccharide inhibited macrophage inflammatory response induced by lipopolysaccharide (Zhao et al., Citation2017). We recently reported a crude F. velutipes polysaccharide (named FVP), which represented a capacity in intestinal microbiota regulation, and speculated that it has a certain immune regulating function by measuring the immune globulin and cellular immune factors in mouse serum (Zhao et al., Citation2019). However, the stimulation effect of this bioactive polysaccharide on the immune system and the relationship between gut microbiota and immune functional changes remains to be fully understood. Hence, we report here a coupling of in vitro and in vivo methodologies, cell model and animal experiment were utilized to investigate the immune functional characterization of this bioactive polysaccharide, based on gut microbiota regulation.
2. Materials and methods
2.1. Materials and chemicals
FVP was obtained from fresh F. velutipes, as described previously (Zhao et al., Citation2019). To be specific, FVP consisted of 81.01% polysaccharide, 7.07% monosaccharide, 3.52% uronic acids and 1.80% total phenols composed of mannose, glucose, xylose, arabinose and fucose. Moreover, this polysaccharide with furanose ring linked by β-glycosidic bonds, composed of two components, and its molecular weight is 7473.14 KDa (48.09%) and 15.077 KDa (51.91%) (Table S1).
Dulbecco’s modified Eagle’s medium (DMEM), Fetal Bovine Serum (FBS), penicillin and streptomycin combination, trypsin and LPS were obtained from Sigma-Aldrich Chemical Reagent Co., Ltd. (America). Lactate dehydrogenase (LDH) cytotoxicity assay kit, Neutral red cell proliferation assay kit, Nitric oxide detection assay kit were obtained from Beyotime Biotechnology Co., Ltd. (Shanghai, China). Mouse Interleukin 1β and Interleukin 10 ELISA Kit were obtained from Jiancheng Bioengineering Institute (Nanjing, China).
2.2. Cell culture
The RAW 264.7 mononuclear macrophage (China Center For Type Culture Collection, Wuhan, China) was cultured in DMEM including 1% penicillin and streptomycin combination and 10% FBS in a cell incubator at 37°C, 5% CO2.
Macrophage cells were seeded in a 96-well plate (200 μL/well) at a density of 1 × 104 cells/mL and pre-incubated for 24 h. After pre-incubation, the medium was discarded and replaced with 100 μL of fresh medium containing various concentrations (0, 5, 10, 50, 100, 200, 400 and 800 μg/mL) of FVP or LPS (2.5 μg/mL), incubated for another 24 h before further tests.
2.3. Cell viability and cytotoxicity assay
Cell viability was measured using the Thiazolyl Blue Tetrazolium Bromide (MTT) assay. Specifically, 20 μL of MTT was added to each well and incubation was continued for 4 h. After that, the culture medium was removed, 200 μL of dimethyl sulfoxide (DMSO) was added into each well. The absorbance was measured at 570 nm with a plate reader (MQX200, BioTek Instruments, Inc., Vermont, USA).
LDH cytotoxicity assay was measured by LDH cytotoxicity assay kit (Beyotime Biotechnology Co., Ltd), according to the protocol. In brief, the medium was discarded and replaced with 150 μL of LDH release agent and incubated for 1 h. After incubation, culture supernatants (120 μL) in each well were removed in a new 96-well plate. After the addition of LDH detection solution (60 μL) into each well, the absorbance was measured at 490 nm.
Neutral red cell proliferation assay was measured by neutral red cell proliferation assay kit (Beyotime Biotechnology Co., Ltd), according to the protocol. In short, 20 μL of neutral red stain was added into each well and continuously incubated for 2 h. After that, the culture medium was removed, 200 μL neutral red detection lysate was added and reacted for 10 min. The absorbance was measured at 540 nm.
2.4. NO production and cytokine concentrations assay
NO content in cell culture supernatants was quantified using the Nitric Oxide assay kit (Beyotime Biotechnology, Shanghai, China) according to the protocol. In brief, 50 μL cell culture supernatants (contained 50, 100, 200 and 400 μg/mL FVP or 2.5 μg/mL LPS or 5 μg/mL polymyxin B) were transferred into 96-well plate, 50 μL of Griess reagent I and Griess reagent II was added. After incubation for 3 min, the absorbance was measured at 540 nm.
The concentrations of IL-1β and IL-10 in cell culture supernatants were determined using ELISA kits (Jiancheng Bioengineering Institute, Nanjing, China) according to the protocol.
2.5. Animal feeding trials
Four-week-old C57BL/6J male mice (SPF grade, 20 ± 2 g, n = 24) were purchased from Nanjing Medical University (Nanjing, China, SCXK2016-0002), the experimental procedures were carried out in the Animal Center at Nanjing Agricultural University (SYXK--2017-0007). Each mouse was acclimated to the living condition of 25 ± 2°C and a 12/12 h light and dark cycle for 7 days. All mice were fed with a semi-purified diet, the diet compositions are shown in Table S2. After 7 days of acclimation, the mice were randomly divided into two groups (SD and SD-FVP). In the SD-FVP group, the mice were fed with FVP (400 mg/kg b.w.) by gavage every day during the experimental period for 16 weeks. In the SD group, the mice were given distilled water as blank control by gavage. The administration volume for gavage feeding was 0.1 mL/10 g body weight. All the mice were raised with free access to diet and water, and the feed consumptions were recorded weekly. At the end of the experimental period, all mice were sacrificed by cervical dislocation after carbon-dioxide absorption anaesthesia, serum and faeces were harvested and stored at −80°C prior to the analysis.
2.6. Extraction of colon tissue RNA and relative gene expression
The relative gene expression in colon tissue was analyzed by qRT-PCR. Total RNA of colon was extracted by using Trizol (Takara Bio. Inc., Japan), quantified by an SMA2000 Ultra-micro UV spectrophotometer (Thermo Fisher Scientific Inc., USA). cDNA was synthesized by Hifair® II 1st Strand cDNA Synthesis SuperMix (YEASEN Bio. Inc., Shanghai, China). Fluorescence quantitative PCR was performed with Hieff® qPCR SYBR Green Master Mix (YEASEN Bio. Inc., Shanghai, China) and qPCR fluorescence quantification system (BIOER Inc., Hangzhou, China). Relative gene expression level was calculated by 2−ΔΔCt, glyceraldehyde-3-phosphate dehydrogenase (GAPDH) as the housekeeping gene. The Primer Sequence is shown in Table S3.
2.7. Extraction of faecal DNA and high-throughput sequencing
The DNA of total bacteria in mice faeces was extracted with E.Z.N.A.™ Bacterial DNA Kit (Omega Bio-tek, Inc., USA), stored at −20°C prior to the analysis. For 16S rDNA absolute quantitative sequencing, the DNA samples were sent to Genesky Biological Technology Co., Ltd. (Shanghai, China). Nanodrop 2000 and Qubit quantitative were used for DNA quantified. In short, a specific tag sequence was introduced into the library end by high-fidelity PCR using Primer F: CCTACGGGNGGCWGCAG and Primer R: GACTACHVGGGTATCTAATCC. High-fidelity PCR was used to introduce specific sequence tags with Index sequences. For each faecal sample, sequencing and bioinformatic analysis were carried out on the Illumina MiSeq platform to generate 2 × 250 bp paired-end reads. Alpha-diversity analyses were determined utilizing the MOTHUR software. Community structure comparisons were conducted using the MOTHUR program and R software.
2.8. Ethics statement
Animals were maintained in accordance with the guidelines of the NIH (National Institutes of Health U.S.A) (2011) Guide for the Care and Use of Laboratory Animals. Washington, D.C.
2.9. Statistical analysis
The data were presented as mean ± standard deviation. One-way analysis of variance (ANOVA) procedure followed by Tukey test was used to evaluate the statistical significance by SPSS software (SPSS Inc., Chicago, IL, USA).
3. Result and discussion
3.1. Cytotoxicity effects of FVP on macrophages
The cell proliferation, cytotoxicity and neutral red phagocytosis of FVP on macrophages are shown in . Under the stimulation of LPS (2.5 μg/mL), the cell proliferation was significantly higher than that of the control group (P < .05). When the stimulating concentration of FVP was between 10 and 400 μg/mL, the cell proliferation was increased, compared with the control (P < .05). In contrast, the cell proliferation showed a decreased trend with the FVP concentration increasing to 800 μg/mL, compared with other groups ((a)). The same trend was found in the LDH-released amounts ((b)). The apoptosis or necrosis of macrophages would cause the destruction of cell membrane structure, leading to the release of enzymes from the cytoplasm into the culture medium, as a kind of stable enzyme, LDH in the cell culture supernatant is commonly used for cytotoxicity testing (Reddy & Reddanna, Citation2009). (c) shows the macrophage phagocytosis index tested by the neutral red cell proliferation assay kit. The phagocytosis index increased with the treatment of FVP and LPS, reached maximum under 100 μg/mL FVP treated, when the FVP dose was up to 800 μg/mL, the cell proliferation showed a downtrend. Meanwhile, the amount of LDH release showed a destruction of the cell membrane structure. Thus, FVP showed no-cytotoxicity effects at a concentration of 0–400 μg/mL and showed best activation ability at a concentration of 100 μg/mL.
3.2. FVP induced the secretion of NO and selective cytokines in macrophage
The NO production in cell culture supernatant is shown in (a,b). The control group generated 4.21 ± 0.37 μmol/L of NO, whereas cells were stimulated with LPS (2.5 μg/mL) as a positive control, the NO production raised to 7.42 ± 0.77 μmol / L in cell culture supernatant. The NO production reached highest (7.15 ± 0.18 μmol/L) when treated under 100 μg/mL FVP ((a)), showed no significant difference with positive control (LPS group) and represented a decreasing trend along with the increasing dosage of FVP. Endogenic LPS in the edible mushroom polysaccharides could lead to misleading results. Thus, to verify if the increased NO production in cell culture supernatant was responsive to FVP stimulation, rather than the endogenic LPS existed in FVP, cultures contained polymyxin B (Poly B) were tested ((b)). As a LPS inhibitor, Poly B neutralized the biological activity of LPS by blinding the lipid A-ketone deoxyoctonate region (Cheong et al., Citation2016). It was shown that LPS and Poly B were added jointly, the NO production was inhibited significantly compared with the cells stimulated by LPS without Poly B. Meanwhile, with the joint addition of FVP and Poly B, NO production showed a decreasing trend compared with the cell stimulated only by FVP, but was still higher than the blank control group. Combining the result from (a,b), the stimulation effect of FVP on macrophages mainly attributed to its unique chain structure, but the effect of the endogenous LPS still exists. The other studies also pointed out that the endogenous LPS, from bioactive compounds, was not attributed to the immunomodulatory function (Castro-Alves et al., Citation2017).
Figure 2. (a,b) NO production; (c) IL-1β concentration; (d) IL-10 concentration. Values are means ± SDs. Different lowercase letters (a > b > c > d) mean a significant difference in a different treatment group (P < .05).
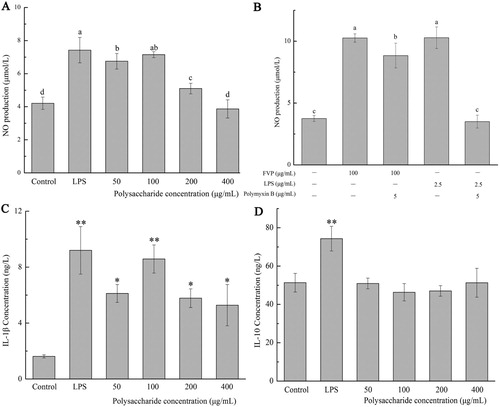
The concentrations of cytokines IL-1β and IL-10 in cell culture supernatant are shown in (c,d). The concentrations of IL-1β were increased with the treatment of FVP and LPS (8.58 ± 1.01 ng/L and 9.20 ± 1.70 ng/L, respectively), compared with the control (P < .05) ((c)). Conversely, no significant changes showed in IL-10 concentrations under the FVP stimulation ((d)). Having served as a complex and important physiological system, immune system will cause allergic, inflammation or immunosuppression under the circumstance of imbalance. Macrophages that fight together with other immune cells represent the first line of defence after the epithelial barrier (Tran Van & Suzuki, Citation2016). Without significant side effects, edible or medical mushroom polysaccharides will be regarded as an ideal candidates for immunoactivator in activating macrophage, restoring macrophage activity and reducing cell damage caused by oxidation (Hou et al., Citation2019; Ma et al., Citation2017). Activated macrophages promoted the immune regulation effects by secreting interleukins and tumour necrosis factor, eliminating endogenous abnormal cells and exogenous pathogens. In non-inflammatory models, the levels of pro-inflammatory cytokines generated by macrophages can be used as an index to evaluate the immune activation capacity of natural products (Cheong et al., Citation2016). IL-1β and IL-10 are typical productions generated by M1 and M2 subtype macrophages, respectively (Mantovani et al., Citation2004; Qin et al., Citation2012). The immune modulatory activity of polysaccharides is closely related to their molecular weight, it is reported that lycium barbarum polysaccharides with lower molecular weights are the main active fractions that enhance macrophage function (Deng et al., Citation2018). Our results indicated that FVP, as an immune activator, makes the macrophages polarized into M1 subtype to exert immune regulatory functions, and the fraction with the molecular weight of 15 KDa (accounting for 51.91% of the total) may play the main active role.
3.3. FVP regulated the relative mRNA expressions in mice colon tissue
Based on the results of in vitro study, we also investigated the in vivo effects of FVP in mice. All mice showed a healthy physiological state during the experiment, and the changes of mice body weight, food consumption and energy intake are shown in . In addition, the effects of FVP on the expression of mRNA levels involved in IL-1β, IL-10, inducible nitric oxide synthases (iNOS) and tight junction protein (ZO-1) in mice colon tissue are shown in . It can be found in SD-FVP that the relative mRNA expression of IL-1β, iNOS and ZO-1 was increased by the FVP treatment, compared with the SD group.
Figure 4. The Effects of FVP on the relative expression of mRNA in the colon. (a) IL-1β; (b) IL-10; (c) iNOS; (d) ZO-1. Values are means ± SDs. n = 12. Different lowercase letters (a > b) mean a significant difference in a different treatment group (P < .05).
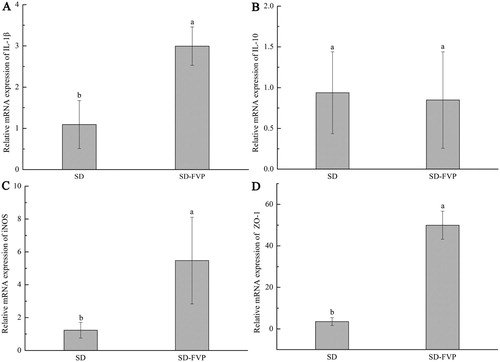
The expression of iNOS was examined to verify the consistency of in vivo and in vitro experiments. As a secondary messenger molecule between host and gut microbes, NO plays an important role in gastrointestinal protection and defence mechanisms of the host, intestinal bacteria secrete endogenous NO and proceed protein modification due to S-nitrosylation, thus, the gut microbiota can shape the post-translational landscape of the host proteome to regulate microRNA activity, gene expression and host development (Seth et al., Citation2019). Intestinal epithelial tight junction protein, ZO-1, which is the key to the intestinal mucosal barrier, plays an important role in the immune response. It is involved in mediating cellular material transport, and as the sign of the gut mechanical barrier and epithelial integrity, it also plays a role in the process of cell proliferation, tumour cell metastasis and gene transcription (Liu et al., Citation2017; Stevenson et al., Citation1986). Due to the large molecular weight, FVP cannot be absorbed by mouse intestinal epithelium, but it can interact with mouse spleen cells to initiate the mucosal immune system and maintain the integrity of intestinal mucosal barrier (Nie et al., Citation2019). The immune regulation of FVP in our result was similar to those reported for other mushroom polysaccharides (Ike et al., Citation2012; Wang et al., Citation2014).
3.4. FVP changed mice gut microbial diversity and relative abundance
Mice gut microbial diversity analysis was conducted, as shown in . The Principal Component Analysis (PCA) ((a)), followed by the provision of 90% ellipses, showed that the SD-FVP group was completely separated from the SD group in X-axis (16.766%). Sample cluster tree ((b)) and heat map also showed that FVP significantly altered intestinal microbial composition. Meanwhile, it can be seen from the Venn diagram ((d)) that 101 OTUs which differ from the SD group were detected in the SD-FVP group.
Figure 5. Mouse faecal microbiota diversity based on 16S rRNA gene sequences. (a) Principal Component Analysis (PCA); (b) sample cluster tree; (c) heat map; (d) Venn diagram.
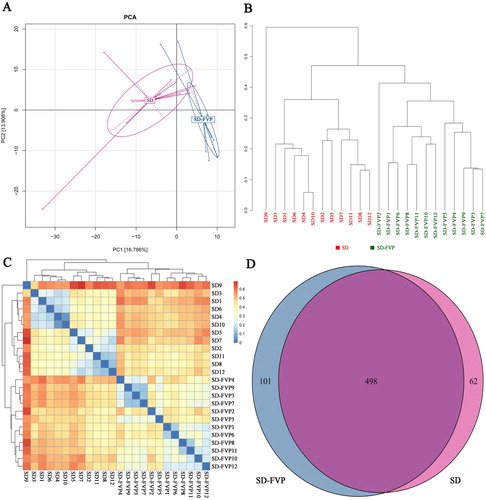
The changes of mice gut microbes are shown in , the stack column showed that each sample was composed mainly of Bacteroidetes, Firmicutes, Verrucomicrobia, Actinobacteria and Proteobacteria. The relative abundance of Bacteroidetes was increased by FVP treatment (P < .05) ((b)). Conversely, Firmicutes was significantly decreased under the FVP treatment ((c)). The relative abundances of Verrucomicrobia, Actinobacteria and Proteobacteria are variable at (a). The family level showed the discrepancy in relative abundance of Porphyromonadaceae, Lachnospiraceae, Prevotellaceae, Sutterellaceae, Bacteroidaceae and Desulfovibrionaceae ((a)). The relative abundance of Porphyromonadaceae showed an increased trend in the SD-FVP group, and the same trend occurred in Prevotellaceae and Sutterellaceae. The opposite trend was found in Lachnospiraceae, Bacteroidaceae and Desulfovibrionaceae ((b–g)).
Figure 6. Gut microbial compositions of mice at phylum level. (a) Relative abundance of major phyla across 24 faecal microbiomes; (b) Relative abundance of Bacteroidetes across each grouped microbiome; (c) Relative abundance of Firmicutes across each grouped microbiome. Values are means ± SDs. n = 12. Different lowercase letters (a > b) mean a significant difference in a different treatment group (P < .05).
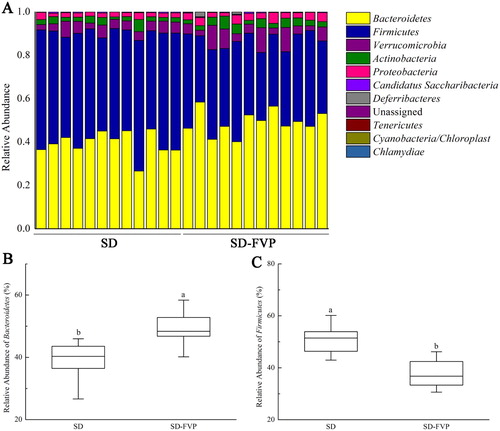
Figure 7. Gut microbial compositions of mice at the family level. (a) Relative abundance of major species across 24 faecal microbiomes; (b–g) Relative abundance of different species across each grouped microbiome. Values are means ± SDs. n = 12. Different lowercase letters (a > b) mean a significant difference in a different treatment group (P < .05).
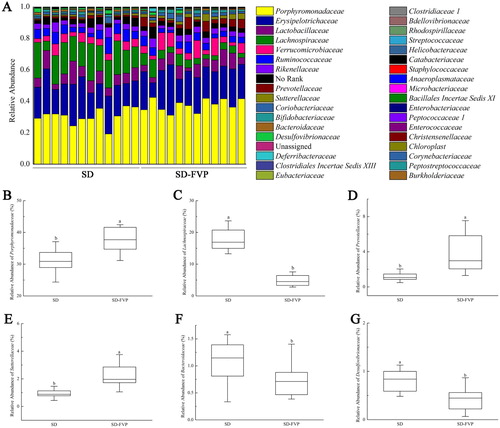
As the key players in mammalian physiology, the intestinal microbiota composition is influenced by genetics, environment and diet, and any changes in intestinal microbes could feedback the host (Lamas et al., Citation2016). At the phylum level, the ratio of Firmicutes to Bacteroidetes was decreased under the FVP treatment, which was close to other research on the effect of dietary fibres (Mou et al., Citation2020). At the family level, Porphyromonadaceae is an important mediator of gut health in the murine gut, one mechanism is that it could degrade complex carbohydrates into short chain fatty acids (SCFAs), such as butyrate (Sakamoto et al., Citation2009). Loss of butyrate-producing microbes could increase both inflammation and tumorigenesis (Segain et al., Citation2000). Prevotellaceae and Porphyromonadaceae are related to the host’s immune response, the absence of these two kinds of gut microbes may lead to a downtrend of the host’s immune response, it is reported that a significant loss of this two kinds of microbes was found in the gut of tumour mice (Zackular et al., Citation2013). Desulfovibrionaceae is the most important sulphate-reducing bacteria (SRB) in the gut, it decomposes short chain fatty acids, amino acids and organic acids in the intestine, reduces sulphate to produce H2S. The accumulation of H2S will block the butyrate oxidation pathway, break disulphide bonds and cause chronic inflammation (Jorgensen & Mortensen, Citation2001). Therefore, the enrichment of SRB in the gut may cause toxic effects on intestinal epithelial cells and destroy the intestinal stable ecosystem (Scanlan et al., Citation2009). The existence of Lachnospiraceae in the gut may trigger an excessive immune response of the host and induce inflammatory bowel diseases (Peng et al., Citation2019).
3.5. Correlation analysis between gut microbes and mRNA expression
shows the further investigation of the correlation analysis between mice colon tissue-related gene expression and gut microbes and 17 species gut microbes for correlation analysis. The intestinal microbe of these 17 species showed significant or extremely significant correlation with the related gene expression, associated with the cytokine production, immune response and intestinal mucosal integrity. Allobaculum, Lactobacillus, Alloprevotella increased in genus level after the FVP treatment and showed a strong positive correlation with related gene expression (P < .01). In addition to this, Akkermansia, Alistipes, Parasutterella, Bifidobacterium and Olsenella showed positive correlations (P < .05). Barnesiella and Clostridium, in contrast, decreased in genus level and showed a negative correlation (P < .01 in Barnesiella and P < .05 in Clostridium, respectively).
Figure 8. Correlation of 17 species altered by FVP intervention by the Spearman correlation analysis. Heatmap shows the value of correlation coefficient. * and ** indicate the significant associations (P < .05 and P < .01, respectively). The circles (○) and dots (●) represent the less and more relative abundances of genus in the SD-FVP group compared with the SD group.
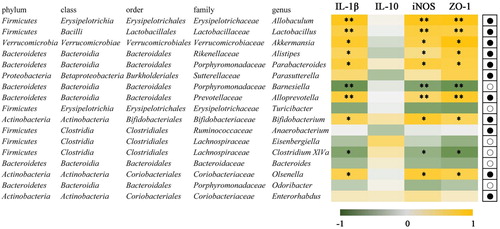
It is reported that Allobaculum may have an intimate relationship with obesity and could be considered as an indicator for obesity (Tachon et al., Citation2013). The SCFA producers, such as Allobaculum, Alloprevotella and Bifidobacterium, could have beneficial effects on the organism through the secretion of SCFA, such as maintaining colon health and anti-inflammation (Shang et al., Citation2017; Wang et al., Citation2018). The beneficial effects of Akkermansia on intestinal function are mainly due to their positive modulation in the mucus thickness and gut barrier integrity (Belzer & de Vos, Citation2012). Dietary fibre and prebiotics could increase the abundance of Akkermansia, which will have a positive effect on associated diseases (Shang et al., Citation2017). Alloprevotella in the genus level may play a key role in protecting newborn mice from maternal mice and Barnesiella has been proved to be linked with energy metabolism in the host (Zhou et al., Citation2019).
3.6. FVP changed microbial community functions predicted by PICRUSt
According to the changes in intestinal microbiota, the functional changes caused by FVP were predicted by PICRUSt, as exhibited in . The SD-FVP group revealed that 28 (16 enriched, 12 depleted) functional modules were altered. The functional changes were mainly noticed in some metabolism pathways. After treated with FVP, 16 functional modules were enriched, mostly in some degradation functions, such as dichlorodiphenyltrichloroethane (DDT) degradation, toluene degradation, riboflavin metabolism, phenylalanine metabolism, arachidonic acid and butanoate metabolism. The functional modules associated with starch and sucrose metabolism, galactose metabolism, methane metabolism, phenylpropanoid biosynthesis were found depleted in the SD-FVP group, compared with the SD group (P < .05).
Figure 9. PICRUSt predicted microbial community function changes reversed by FVP. *, ** and *** indicate the significant associations.
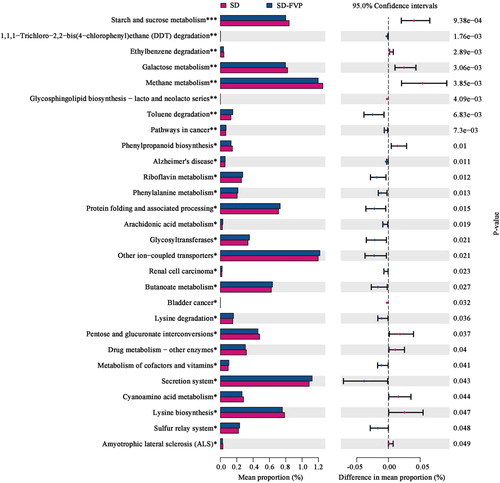
Related research shows that the accumulation of DDT in the body may cause a number of associated complex disease traits, including immune abnormalities and kidney disease, which are also confirmed to be passed on to offspring (Skinner et al., Citation2013). Observations demonstrate that through the effect of FVP on intestinal microbiota, the functional modules of DDT degradation are improved (P = .00176). The enriched functional modules of butanoate metabolism (P = .0266) are mainly due to the increased abundance in intestinal probiotics, including Allobaculum, Alloprevotella and Bifidobacterium as mentioned earlier. Polysaccharides serve as fermentation substrates for acid-producing bacteria, promote the production of butyrate, leading to the expansion of RORgt + Treg cells and IgA-producing cells in the colonic lamina propria (Yamada et al., Citation2019). Butyrate is also represented in regulating the activity of G protein-coupled receptors (GPRs) (Sun & Ye, Citation2012) and inflammation-related pathways, such as NF-κB and JAK/STAT (Aguilar et al., Citation2014), inhibiting the intestinal inflammation and maintaining the intestinal immune balance (Hamer et al., Citation2008). The depleted functional modules of starch and sucrose metabolism might be related to the ratio of Firmicutes/Bacteroidetes. Gut microbes have the ability in encoding carbohydrate cleaving and modifying enzymes, which allows them to take advantage of bioactive macromolecules (Mariat et al., Citation2009). In addition, the butyric acid produced by intestinal microbes also improves the body’s energy balance. Eubacterium rectale and Roseburia intestinalis utilize 4-aminobutyric acid in regulating carbohydrate metabolism and promoting the secretion of insulin to regulate blood glucose (Sanna et al., Citation2019; Zhao et al., Citation2018).
4. Conclusion
In summary, our findings demonstrated that FVPs have the ability in inducing the activation of macrophages. The protective effects of FVP on intestinal immunity were associated with the relative gene expression, including cytokines, iNOS and ZO-1 in mouse’s colon tissue. Moreover, Allobaculum, Lactobacillus, Akkermansia, Alistipes, Alloprevotella, Bifidobacterium and Olsenella were all found increased in mice’s faeces. The role of FVP on intestinal immunity is reflected in the enhancement of harmful chemical degradation and in weakening the carbohydrate metabolism. From this, we hope that insights gained into how certain foods promote human health, coupled with the knowledge of their mechanism, will lead to the development of new functional foods and pharmaceutical products, like FVP, natural product polysaccharides.
Supplemental Material
Download MS Word (580.3 KB)Acknowledgements
This work is financially supported by the National Natural Science Foundation of China (No. 31872898) and the Jiangsu Provincial Key Research and Development Program (BE2017374).
Disclosure statement
No potential conflict of interest was reported by the author(s).
Additional information
Funding
References
- Abarikwu, S. O. (2014). Kolaviron, a natural flavonoid from the seeds of Garcinia kola, reduces LPS-induced inflammation in macrophages by combined inhibition of IL-6 secretion, and inflammatory transcription factors, ERK1/2, NF-kappa B, p38, Akt, p-c-JUN and JNK. Biochimica Et Biophysica Acta-General Subjects, 1840(7), 2373–2381. https://doi.org/10.1016/j.bbagen.2014.03.006
- Aguilar, E. C., Leonel, A. J., Teixeira, L. G., Silva, A. R., Silva, J. F., Pelaez, J. M. N., Capettini, L. S. A., Lemos, V. S., Santos, R. A. S., & Alvarez-Leite, J. I. (2014). Butyrate impairs atherogenesis by reducing plaque inflammation and vulnerability and decreasing NF kappa B activation. Nutrition Metabolism and Cardiovascular Diseases, 24(6), 606–613. https://doi.org/10.1016/j.numecd.2014.01.002
- Belzer, C., & de Vos, W. M. (2012). Microbes inside-from diversity to function: The case of Akkermansia. ISME Journal, 6(8), 1449–1458. https://doi.org/10.1038/ismej.2012.6
- Blacher, E., Levy, M., Tatirovsky, E., & Elinav, E. (2017). Microbiome-modulated metabolites at the interface of host immunity. Journal of Immunology, 198(2), 572–580. https://doi.org/10.4049/jimmunol.1601247
- Castro-Alves, V. C., Gomes, D., Menolli, N., Jr., Sforca, M. L., & Oliveira do Nascimento, J. R. (2017). Characterization and immunomodulatory effects of glucans from Pleurotus albidus, a promising species of mushroom for farming and biomass production. International Journal of Biological Macromolecules, 95, 215–223. https://doi.org/10.1016/j.ijbiomac.2016.11.059
- Chen, X., Fang, D., Zhao, R., Gao, J., Kimatu, B. M., Hu, Q., Chen, G., & Zhao, L. (2019). Effects of ultrasound-assisted extraction on antioxidant activity and bidirectional immunomodulatory activity of Flammulina velutipes polysaccharide. International Journal of Biological Macromolecules, 140, 505–514. https://doi.org/10.1016/j.ijbiomac.2019.08.163
- Cheong, K.-L., Meng, L.-Z., Chen, X.-Q., Wang, L.-Y., Wu, D.-T., Zhao, J., & Li, S.-P. (2016). Structural elucidation, chain conformation and immuno-modulatory activity of glucogalactomannan from cultured Cordyceps sinensis fungus UM01. Journal of Functional Foods, 25, 174–185. https://doi.org/10.1016/j.jff.2016.06.002
- Deng, X., Liu, Q., Fu, Y., Luo, X., Hu, M., Ma, F., Wang, Q., Lai, X., & Zhou, L. (2018). Effects of Lycium barbarum polysaccharides with different molecular weights on function of RAW264.7 macrophages. Food and Agricultural Immunology, 29(1), 808–820. https://doi.org/10.1080/09540105.2018.1457628
- Fan, G. W., Jiang, X. R., Wu, X. Y., Fordjour, P. A., Miao, L., Zhang, H., Zhu, Y., & Gao, X. M. (2016). Anti-inflammatory activity of Tanshinone IIA in LPS-stimulated RAW264.7 macrophages via miRNAs and TLR4-NF-kappa B pathway. Inflammation, 39(1), 375–384. https://doi.org/10.1007/s10753-015-0259-1
- Gao, C., Ganesh, B. P., Shi, Z., Shah, R. R., Fultz, R., Major, A., Venable, S., Lugo, M., Hoch, K., Chen, X., Haag, A., Wang, T. C., & Versalovic, J. (2017). Gut microbe-mediated suppression of inflammation-associated colon carcinogenesis by luminal histamine production. American Journal of Pathology, 187(10), 2323–2336. https://doi.org/10.1016/j.ajpath.2017.06.011
- Hamer, H. M., Jonkers, D., Venema, K., Vanhoutvin, S., Troost, F. J., & Brummer, R. J. (2008). Review article: The role of butyrate on colonic function. Alimentary Pharmacology & Therapeutics, 27(2), 104–119. https://doi.org/10.1111/j.1365-2036.2007.03562.x
- Hou, K., Yu, Q., Hu, X., Ding, X., Hong, J., Chen, Y., Xie, J., Nie, S., & Xie, M. (2019). Protective effect of Ganoderma atrum polysaccharide on acrolein-induced macrophage injury via autophagy-dependent apoptosis pathway. Food and Chemical Toxicology, 133:110757. https://doi.org/10.1016/j.fct.2019.110757
- Ike, K., Kameyama, N., Ito, A., & Imai, S. (2012). Induction of a T-Helper 1 (Th1) immune response in mice by an extract from the Pleurotus eryngii (Eringi) mushroom. Journal of Medicinal Food, 15(12), 1124–1128. https://doi.org/10.1089/jmf.2012.2239
- James, K. R., Gomes, T., Elmentaite, R., Kumar, N., Gulliver, E. L., King, H. W., Stares, M. D., Bareham, B. R., Ferdinand, J. R., Petrova, V. N., Polański, K., Forster, S. C., Jarvis, L. B., Suchanek, O., Howlett, S., James, L. K., Jones, J. L., Meyer, K. B., Clatworthy, M. R., … Teichmann, S. A. (2020). Distinct microbial and immune niches of the human colon. Nature Immunology, 21(3), 343–353. https://doi.org/10.1038/s41590-020-0602-z
- Jorgensen, J., & Mortensen, P. B. (2001). Hydrogen sulfide and colonic epithelial metabolism - Implications for ulcerative colitis. Digestive Diseases and Sciences, 46(8), 1722–1732. https://doi.org/10.1023/A:1010661706385
- Lamas, B., Richard, M. L., Leducq, V., Pham, H.-P., Michel, M.-L., Da Costa, G., Bridonneau, C., Jegou, S., Hoffmann, T. W., Natividad, J. M., Brot, L., Taleb, S., Couturier-Maillard, A., Nion-Larmurier, I., Merabtene, F., Seksik, P., Bourrier, A., Cosnes, J., Ryffel, B., … Sokol, H. (2016). CARD9 impacts colitis by altering gut microbiota metabolism of tryptophan into aryl hydrocarbon receptor ligands. Nature Medicine, 22(6), 598–605. https://doi.org/10.1038/nm.4102
- Li, S. P., Zhao, X. J., & Wang, J. Y. (2009). Synergy of Astragalus polysaccharides and probiotics (Lactobacillus and Bacillus cereus) on immunity and intestinal microbiota in chicks. Poultry Science, 88(3), 519–525. https://doi.org/10.3382/ps.2008-00365
- Liu, C., Chen, J., Chen, L., Huang, X., & Cheung, P. C. K. (2016). Immunomodulatory activity of polysaccharide–protein complex from the mushroom Sclerotia of Polyporus rhinocerusin murine macrophages. Journal of Agricultural and Food Chemistry, 64(16), 3206–3214. https://doi.org/10.1021/acs.jafc.6b00932
- Liu, W., Mi, S., Ruan, Z., Li, J., Shu, X., Yao, K., Jiang, M., & Deng, Z. (2017). Dietary Tryptophan enhanced the expression of tight junction protein ZO-1 in intestine. Journal of Food Science, 82(2), 562–567. https://doi.org/10.1111/1750-3841.13603
- Ma, G., Kimatu, B. M., Zhao, L., Yang, W., Pei, F., & Hu, Q. (2017). In vivo fermentation of a Pleurotus eryngii polysaccharide and its effects on fecal microbiota composition and immune response. Food & Function, 8(5), 1810–1821. https://doi.org/10.1039/C7FO00341B
- Mantovani, A., Sica, A., Sozzani, S., Allavena, P., Vecchi, A., & Locati, M. (2004). The chemokine system in diverse forms of macrophage activation and polarization. Trends in Immunology, 25(12), 677–686. https://doi.org/10.1016/j.it.2004.09.015
- Mariat, D., Firmesse, O., Levenez, F., Guimaraes, V. D., Sokol, H., Dore, J., Corthier, G., & Furet, J. P. (2009). The Firmicutes/Bacteroidetes ratio of the human microbiota changes with age. BMC Microbiology, 9(1):123. https://doi.org/10.1186/1471-2180-9-123
- Meng, X., Liang, H., & Luo, L. (2016). Antitumor polysaccharides from mushrooms: A review on the structural characteristics, antitumor mechanisms and immunomodulating activities. Carbohydrate Research, 424, 30–41. https://doi.org/10.1016/j.carres.2016.02.008
- Mou, J., Li, Q., Shi, W., Qi, X., Song, W., & Yang, J. (2020). Chain conformation, physicochemical properties of fucosylated chondroitin sulfate from sea cucumber Stichopus chloronotus and its in vitro fermentation by human gut microbiota. Carbohydrate Polymers, 228:115359. https://doi.org/10.1016/j.carbpol.2019.115359
- Navegantes, K. C., Albuquerque, R. F. V., Dalla-Santa, H. S., Soccol, C. R., & Monteiro, M. C. (2013). Agaricus brasiliensis mycelium and its polysaccharide modulate the parameters of innate and adaptive immunity. Food and Agricultural Immunology, 24(4), 393–408. https://doi.org/10.1080/09540105.2012.691089
- Nie, L., Xiao, Q., Liu, S., Li, B., Duan, J., Fan, Y., Guo, L., He, C., & Zhu, H. (2019). Immune-enhancing effects of polysaccharides MLN-1 from by-product of Mai-luo-ning in vivo and in vitro. Food and Agricultural Immunology, 30(1), 369–384. https://doi.org/10.1080/09540105.2019.1582612
- Ohnmacht, C., Marques, R., Presley, L., Sawa, S., Lochner, M., & Eberl, G. (2011). Intestinal microbiota, evolution of the immune system and the bad reputation of pro-inflammatory immunity. Cellular Microbiology, 13(5), 653–659. https://doi.org/10.1111/j.1462-5822.2011.01577.x
- Peng, Y., Yan, Y., Wan, P., Chen, D., Ding, Y., Ran, L., Mi, J., Lu, L., Zhang, Z., Li, X., Zeng, X., & Cao, Y. (2019). Gut microbiota modulation and anti-inflammatory properties of anthocyanins from the fruits of lycium ruthenicum Murray in dextran sodium sulfate-induced colitis in mice. Free Radical Biology and Medicine, 136, 96–108. https://doi.org/10.1016/j.freeradbiomed.2019.04.005
- Qin, H., Holdbrooks, A. T., Liu, Y., Reynolds, S. L., Yanagisawa, L. L., & Benveniste, E. N. (2012). SOCS3 deficiency promotes M1 macrophage polarization and inflammation. The Journal of Immunology, 189(7), 3439–3448. https://doi.org/10.4049/jimmunol.1201168
- Reddy, D. B., & Reddanna, P. (2009). Chebulagic acid (CA) attenuates LPS-induced inflammation by suppressing NF-kappa B and MAPK activation in RAW 264.7 macrophages. Biochemical and Biophysical Research Communications, 381(1), 112–117. https://doi.org/10.1016/j.bbrc.2009.02.022
- Sakamoto, M., Takagaki, A., Matsumoto, K., Kato, Y., Goto, K., & Benno, Y. (2009). Butyricimonas synergistica gen. nov., sp nov and Butyricimonas virosa sp nov., butyric acid-producing bacteria in the family ‘Porphyromonadaceae’ isolated from rat faeces. International Journal of Systematic and Evolutionary Microbiology, 59(7), 1748–1753. https://doi.org/10.1099/ijs.0.007674-0
- Sanna, S., van Zuydam, N. R., Mahajan, A., Kurilshikov, A., Vila, A. V., Vosa, U., Mujagic, Z., Masclee, A. A. M., Jonkers, D. M. A. E., Oosting, M., Joosten, L. A. B., Netea, M. G., Franke, L., Zhernakova, A., Fu, J., Wijmenga, C., & McCarthy, M. I. (2019). Causal relationships among the gut microbiome, short-chain fatty acids and metabolic diseases. Nature Genetics, 51(4), 600. https://doi.org/10.1038/s41588-019-0350-x
- Scanlan, P. D., Shanahan, F., & Marchesi, J. R. (2009). Culture-independent analysis of desulfovibrios in the human distal colon of healthy, colorectal cancer and polypectomized individuals. Fems Microbiology Ecology, 69(2), 213–221. https://doi.org/10.1111/j.1574-6941.2009.00709.x
- Segain, J. P., de la Bletiere, D. R., Bourreille, A., Leray, V., Gervois, N., Rosales, C., Ferrier, L., Bonnet, C., Blottière, H. M., & Galmiche, J. P. (2000). Butyrate inhibits inflammatory responses through NF kappa B inhibition: Implications for Crohn's disease. Gut, 47(3), 397–403. https://doi.org/10.1136/gut.47.3.397
- Seth, P., Hsieh, P. N., Jamal, S., Wang, L., Gygi, S. P., Jain, M. K., Coller, J., & Stamler, J. S. (2019). Regulation of MicroRNA machinery and development by interspecies S-nitrosylation. Cell, 176(5), 1014. https://doi.org/10.1016/j.cell.2019.01.037
- Shang, Q., Song, G., Zhang, M., Shi, J., Xu, C., Hao, J., Li, G., & Yu, G. (2017). Dietary fucoidan improves metabolic syndrome in association with increased Akkermansia population in the gut microbiota of high-fat diet-fed mice. Journal of Functional Foods, 28, 138–146. https://doi.org/10.1016/j.jff.2016.11.002
- Skinner, M. K., Manikkam, M., Tracey, R., Guerrero-Bosagna, C., Haque, M., & Nilsson, E. E. (2013). Ancestral dichlorodiphenyltrichloroethane (DDT) exposure promotes epigenetic transgenerational inheritance of obesity. Bmc Medicine, 11(1):228. https://doi.org/10.1186/1741-7015-11-228
- Stevenson, B. R., Siliciano, J. D., Mooseker, M. S., & Goodenough, D. A. (1986). Identification of ZO-1: A high molecular weight polypeptide associated with the tight junction (zonula occludens) in a variety of epithelia. Journal of Cell Biology, 103(3), 755–766. https://doi.org/10.1083/jcb.103.3.755
- Sun, L., & Ye, R. D. (2012). Role of G protein-coupled receptors in inflammation. Acta Pharmacologica Sinica, 33(3), 342–350. https://doi.org/10.1038/aps.2011.200
- Tachon, S., Zhou, J., Keenan, M., Martin, R., & Marco, M. L. (2013). The intestinal microbiota in aged mice is modulated by dietary resistant starch and correlated with improvements in host responses. Fems Microbiology Ecology, 83(2), 299–309. https://doi.org/10.1111/j.1574-6941.2012.01475.x
- Taofiq, O., Martins, A., Barreiro, M. F., & Ferreira, I. C. F. R. (2016). Anti-inflammatory potential of mushroom extracts and isolated metabolites. Trends in Food Science & Technology, 50, 193–210. https://doi.org/10.1016/j.tifs.2016.02.005
- Tran Van, H., & Suzuki, T. (2016). Dietary Fermentable fiber reduces intestinal barrier Defects and inflammation in Colitic mice. Journal of Nutrition, 146(10), 1970–1979. https://doi.org/10.3945/jn.116.232538
- van Tits, L. J. H., Stienstra, R., van Lent, P. L., Netea, M. G., Joosten, L. A. B., & Stalenhoef, A. F. H. (2011). Oxidized LDL enhances pro-inflammatory responses of alternatively activated M2 macrophages: A crucial role for Kruppel-like factor 2. Atherosclerosis, 214(2), 345–349. https://doi.org/10.1016/j.atherosclerosis.2010.11.018
- Wang, J., Niu, X., Du, X., Smith, D., Meydani, S. N., & Wu, D. (2014). Dietary supplementation with White Button mushrooms augments the protective immune response to Salmonella Vaccine in mice. Journal of Nutrition, 144(1), 98–105. https://doi.org/10.3945/jn.113.185165
- Wang, Z., Zhang, W., Wang, B., Zhang, F., & Shao, Y. (2018). Influence of Bactrian camel milk on the gut microbiota. Journal of Dairy Science, 101(7), 5758–5769. https://doi.org/10.3168/jds.2017-13860
- Wang, S., Zhu, H., Lu, C., Kang, Z., Luo, Y., Feng, L., & Lu, X. (2012). Fermented milk supplemented with probiotics and prebiotics can effectively alter the intestinal microbiota and immunity of host animals. Journal of Dairy Science, 95(9), 4813–4822. https://doi.org/10.3168/jds.2012-5426
- Wu, M., Luo, X., Xu, X., Wei, W., Yu, M., Jiang, N., Ye, L., Yang, Z., & Fei, X. (2014). Antioxidant and immunomodulatory activities of a polysaccharide from Flammulina velutipes. Journal of Traditional Chinese Medicine, 34(6), 733–740. https://doi.org/10.1016/S0254-6272(15)30089-3
- Yamada, T., Hino, S., Iijima, H., Genda, T., Aoki, R., Nagata, R., Han, K.-H., Hirota, M., Kinashi, Y., Oguchi, H., Suda, W., Furusawa, Y., Fujimura, Y., Kunisawa, J., Hattori, M., Fukushima, M., Morita, T., & Hase, K. (2019). Mucin O-glycans facilitate symbiosynthesis to maintain gut immune homeostasis. Ebiomedicine, 48, 513–525. https://doi.org/10.1016/j.ebiom.2019.09.008
- Zackular, J. P., Baxter, N. T., Iverson, K. D., Sadler, W. D., Petrosino, J. F., Chen, G. Y., & Schloss, P. D. (2013). The gut microbiome modulates colon tumorigenesis. Mbio, 4(6):e00692-13. https://doi.org/10.1128/mBio.00692-13
- Zhang, T., Ye, J., Xue, C., Wang, Y., Liao, W., Mao, L., Yuan, M., & Lian, S. (2018). Structural characteristics and bioactive properties of a novel polysaccharide from Flammulina velutipes. Carbohydrate Polymers, 197, 147–156. https://doi.org/10.1016/j.carbpol.2018.05.069
- Zhao, R., Hu, Q., Ma, G., Su, A., Xie, M., Li, X., Chen, G., & Zhao, L. (2019). Effects of Flammulina velutipes polysaccharide on immune response and intestinal microbiota in mice. Journal of Functional Foods, 56, 255–264. https://doi.org/10.1016/j.jff.2019.03.031
- Zhao, S., Li, B., Chen, G., Hu, Q., & Zhao, L. (2017). Preparation, characterization, and anti-inflammatory effect of the chelate of Flammulina velutipespolysaccharide with Zn. Food and Agricultural Immunology, 28(1), 162–177. https://doi.org/10.1080/09540105.2016.1230600
- Zhao, L., Zhang, F., Ding, X., Wu, G., Lam, Y. Y., Wang, X., Fu, H., Xue, X., Lu, C., Ma, J., Yu, L., Xu, C., Ren, Z., Xu, Y., Xu, S., Shen, H., Zhu, X., Shi, Y., Shen, Q., … Zhang, C. (2018). Gut bacteria selectively promoted by dietary fibers alleviate type 2 diabetes. Science, 359(6380), 1151. https://doi.org/10.1126/science.aao5774
- Zhou, L., Xiao, X., Zhang, Q., Zheng, J., Li, M., Wang, X., Deng, M., Zhai, X., & Liu, J. (2019). Gut microbiota might be a crucial factor in deciphering the metabolic benefits of perinatal genistein consumption in dams and adult female offspring. Food & Function, 10(8), 4505–4521. https://doi.org/10.1039/C9FO01046G
- Zong, A., Cao, H., & Wang, F. (2012). Anticancer polysaccharides from natural resources: A review of recent research. Carbohydrate Polymers, 90(4), 1395–1410. https://doi.org/10.1016/j.carbpol.2012.07.026