Abstract
Long-term irrigation using wastewater from paper industry may cause seriously problems to the receiving soil. This work surveyed and monitored the soil quality of a wastewater irrigation wetland system in Yancheng City, Jiangsu Province in China in 2014 and 2015. Τhe wetland soil showed different soil properties and TX, AOX, heavy metal contents after long-term wastewater irrigation. Long-term irrigation also accumulated the heavy metals such as Cu, Cd, Zn, and Pb in the wetland soil. Compared to the control, TX in the irrigated soil increased by 47.7–69.8% (2014) and 61.5–83.1% (2015). AOX varied in concentration from 1.7 to 55.0 mg kg−1 (2014) and 11.0 to 53.0 mg kg−1 (2015). The long-term irrigation of wastewater to wetland systems caused the accumulations of heavy metals, TX, and AOX in the soil and the levels of accumulations were related to several factors including soil properties, wastewater quality, and irrigation time.
1. Introduction
The pulp and paper industry generates the third largest volume of industrial wastewater in the world after chemical and steel industries.[Citation1] Both raw and treated wastewater are often reused in agricultural practices, especially for irrigation and landscaping in many developing countries.[Citation2,3] Irrigation using wastewater may have adverse effects to the receiving soil system by altering its physicochemical and microbiological properties and contaminating it with toxic chemical and biological compounds.[Citation1,4] The latter may pose serious risks to the ecosystem and public health.
Wetland systems are widely used for the treatment of wastewater, especially wastewater from the pulp and paper industry.[Citation5] For example, using the constructed reed wetland to treat wastewater generated during paper production has been regarded as environmentally and economically beneficial because not only this technology has minimum adverse impact on the environment but also the nutrients in the wastewater can improve the production of reeds.[Citation6] However, there are also increasing concerns over the accumulations of heavy metals and persistent organic substances in wetland soils. In considerations of the nature of many contaminants in wastewater, especially their persistence in the subsurface and potential toxic effects, it is crucial to understand their distribution in the receiving soils.[Citation7]
The pulp and paper wastewater often has high levels of organic matters, lignin and its derivatives, heavy metals, and alkaline.[Citation6,8] In addition, chlorine compounds are often used to bleach paper and they react with the organic matters to produce organic chlorine compounds such as adsorbable organic halogens (AOX) in the wastewater. The AOX compounds include chemicals with differing structures and toxicological profiles, such as trichloroethylene, dichloroethylene, dichloroethane, and vinyl chloride. They are hydrophobic, recalcitrant, and persistent and cannot be removed from wastewater through primary and secondary treatment. Although current analytic techniques still cannot evaluate or predict the toxic effects of all the AOX compounds in paper wastewater,[Citation9–11] it is recognized that they are highly toxic and some are carcinogenic and mutagenic.[Citation12,13] When the pulp and paper wastewater discharge enters a wetland, both AOX and heavy metals may be retained in the system and deteriorating soil quality, particularly after long-term operations. It is thus very important to monitor and assess soil quality of the wetland receiving wastewater from the pulp and paper industry.
The main objective of this work was to investigate the changes in soil properties, and spatial distribution of heavy metals and AOX in soil samples in a wetland for the treatment of wastewater from Shuangdeng Paper Co., Ltd., which is the largest nonwood tissue production company in China. The company used a reed field ecological system (RFES) of approximately 34,000 ha for pulp and paper wastewater treatment. The RFES was in continuous stable operation for 13 years since 2001, but stopped its operation since 2014 for ecological protection. Soil samples were taken from different locations in the RFES and analyzed for their basic properties and the contents of heavy metals, total halogenated organic compounds (TX) and AOX.
2. Materials and methods
2.1. Description of the site
The RFES (wetland) was belonged to the Halosols and located in the east of Sheyang, Yancheng, China. The pulp and paper wastewater was first treated by a sewage disposal plant of the company and then discharged into the reed field. The RFES was in continuous stable operation for 13 years from 2001 to 2014. As shown in Figure , the RFES includes a series of ponds to preprocess the treated wastewater discharged from the paper mill (P0, which has been continuously running for 10 years since 2004), six reed fields (P1–P6), and a water distribution network including one total water distribution canal, three sewage irrigation ditches (D1–D3), and four water stored gutters (G1–G4) (Figure ). Good ecological treatment of the RFES was achieved by intermediate diffusion flooded irrigation using a continuous irrigation method combined with an intermittent one. Due to the gradual exploitation of each reed, P1 was not irrigated for a year after continuous irrigation for 13 years since 2001, P2 and P3 were not irrigated for 2 years after continuous irrigation for 12 years since 2002, P4 was continuously irrigated for 10 years since 2004, P5 was continuously irrigated for 9 years since 2005, and P6 was continuously irrigated for 7 years since 2007. Pck is a small size control plot that had never received the wastewater. For the other plots, the RFES was irrigated from April to May every year when the reeds grew. Low level hazardous substances (such as AOX and heavy metals) thus were accumulated in the soil of the wetland.
Figure 1. Schematic diagram of the reed wetland.
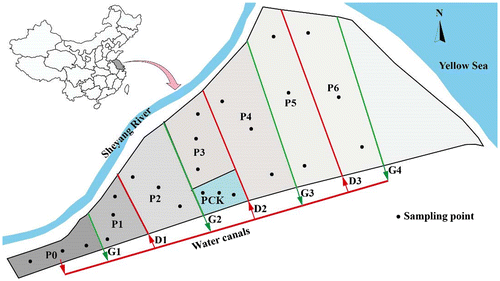
Soil samples were taken from 24 locations in the same place with GPS and ranged from 32.85° N to 34.20° N and from 19.67° E to 120.45° E within the wetland in 2014 and 2015. The soil in the study region is typical coastal solonchaks. The wetland is located in the transit belt from the subtropics to a warm temperate zone, which is characterized by a subtropical, humid monsoon ocean climate with a mean annual temperature and precipitation of 14.6 °C and 960 mm, respectively. The mean maximum and minimum temperature ranges are 29–31 °C (July) and 0–3 °C (January), respectively, with a mean of 230 frost-free days.
2.2. Sample collection
Soil samples were collected in the April of 2014 and 2015. The sampling sites were selected based on wastewater flow conditions at P0, P1, P2, P3, P4, P5, P6, and Pck (Figure ). Four soil profiles were collected from each plot. Soil samples were collected at four depths: 0–20, 20–40, 40–60, and 60–80 cm. Additionally, a composite sample was obtained by mixing four samples from the same soil layers from each of the four soil profiles. All soil samples were stored in plastic valve bags at 4 °C during the sampling process. They were sent to the laboratory within 12 h after sampling. Plant roots from each sample were removed. The air-dried samples were then homogenized and sieved using a 2-mm mesh.
Water samples were also collected at P0, P2, and P4 for analyses. They were collected with plastic vessels from about 10 cm under the surface to represent the whole water column.
2.3. Methods
After carefully removing the roots, part of soil samples with grain sizes of <0.15 mm were prepared. The soil organic matter (SOM), total N, P, pH, and cation exchange capacity (CEC) of each sample were determined in laboratory. Soil pH was measured using a glass electrode in 0.01 mol−1 CaCl2 with a soil-to-solution ratio of 1:2.5.[Citation14] SOM was determined using the dichromate oxidation method.[Citation15] Total soil nitrogen (total N) was determined using the semimicro-Kjeldahl procedure. Total soil phosphorus (total P) was measured using the NaOH fusion–Mo–Sb anti-spectrophotometric method.[Citation16] Heavy metals in the soil were digested using a mixture of aqua regia and hydrofluoric acid at 100 °C for 60 min and further at 250 °C until the sample was concentrated to an approximate volume of 2 mL in the PTFE (polyfluortetraethylene) crucibles, and then dilute with water to 25 mL. These solution was filtered and determined with a Flame Atomic Absorption Spectrophotometer (FAAS, TAS-986, Persee, China).[Citation17] The soil AOX (AOCl, AOBr, AOI) was adsorbed by activated carbon and determined by coupling the AOX-analyzer with a multi X® 2500 (Analytik Jena, Jena, Germany). The procedure was described by Olesky-Frenzel et al.[Citation18]
For the water samples, pH, SS and concentrations of organic compounds (COD) of each sample were determined using the standard methods.[Citation19–21] The nutrient elements (N, TP, Ca, Mg) and harmful elements (Na, Cu, Pb, Cd, AOX) in the water samples were measured using the same methods of the soil samples.[Citation17]
2.4. Statistical analysis
All statistical analyses were performed using office 2013 and SPSS, version 20.0. Differences between the treatments were examined using a two-way analysis of variance (significance level p < 0.05).
3. Results and discussion
3.1. Heavy metals, TX and AOX in the soils
In comparison to that of the Pck, irrigation using pulp and paper wastewater resulted in an increase of 8.3–58.4% (2014) and 6.8–30.7% (2015), 67.5–133.9% (2014) and 8.3–26.2% (2015), 1.9–36.8% (2014) and 19.6–33.0% (2015), and 15.7–38.4% (2014) and 2.6–11.5% (2015) in Cu, Cd, Zn, and Pb in the topsoil (0–20 cm), respectively (Figures (a)–(d), (a)–(d)). The highest concentrations of Cu, Zn, and Pb in the soils were 39.2 mg kg−1(2014) and 42.5 mg kg−1 (2015), 94.0 mg kg−1 (2014) and 81.6 mg kg−1 (2015), 43.4 mg kg−1(2014) and 35.9 mg kg−1 (2015), respectively, which were all lower than the contamination level of the ‘Environmental Quality Standard for Soils (GB15618-1995)’ of China. The Cd in the soils, however, was far above the contamination level with concentrations of 1.0–2.7 mg kg−1 (2014) and 0.6–2.7 mg kg−1 (2015). The concentration of heavy metals decreased with an increase in the depth of the soil, probably because their strong bonding to the soil. To further assess the impact of long-term wastewater irrigation on soil quality, the build-up of heavy metals in soils was computed separately for 13, 12, 10, 10, 9, and 7 years of irrigation. The results showed the longer the duration of irrigation, the higher the concentration of metals, indicating the heavy metals in the wastewater were retained and accumulated in the wetland soils.
The concentration ranges of TX in the profiles soil was increased by 50.3–68.2% (0–20 cm, 2014) and 61.5–81.5% (0–20 cm, 2015), 47.7–67.2% (20–40 cm, 2014) and 64.8–81.5% (20–40 cm, 2015), 50.6–69.8% (40–60 cm, 2014) and 66.4–83.1% (40–60 cm, 2015), and 49.5–69.3% (60–80 cm, 2014) and 63.7–80.8% (60–80 cm, 2015), respectively, when compared to that of Pck (Figure (a), (c)). In general, the topsoil TX concentration was higher than the subsoil and higher TX concentration was also observed closer to the source of wastewater (oxidation pond), suggesting the organic halogen in the soil samples was mainly from the pulp and paper wastewater.
Figure 4. The concentration of TX (a) and AOX (b) in 2014 and TX (c) and AOX (d) in 2015 in the soil profile.
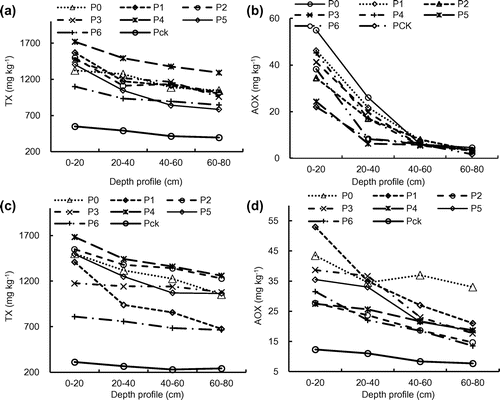
The concentration ranges of total AOX in the soil profiles were 22–55 mg kg−1 (0–20 cm, 2014) and 12.3–53.0 mg kg−1 (0–20 cm, 2015), 8.3–26.1 mg kg−1 (20–40 cm, 2014) and 11.0–36.6 mg kg−1 (20–40 cm, 2015), 5.3–8.1 mg kg−1 (40–60 cm, 2014) and 8.3–37.0 mg kg−1 (40–60 cm, 2015), and 1.7–5.1 mg kg−1 (60–80 cm, 2014) and 7.6–33.0 mg kg−1 (60–80 cm, 2015), respectively (Figure (b), (d)). The changing patterns of soil AOX concentrations was similar to that of the TX concentrations. The top soil had higher AOX concentration than the subsoil and the AOX concentration decreased with the distance from nearer the source of the wastewater. The distribution patterns of AOX and TX in the soil might be attributed to their attenuation in the soil environment.[Citation22] Ding et al. [Citation13] reported that two multi-pollution ciliates (Chaenea teres and Hemiophrys pleurosigma) and one amoeba (Hartmannella cantabrigiensis) emerged and colonized in soil irrigated with the pulp and paper wastewater. Therefore, the soil quality of a field that was rested for a year without irrigation after 10 years of operation was better than that of a field that was continuously operated for 9 years.
3.2. Irrigation water quality
After flowing through the reed field, the quality of the wastewater improved dramatically (Table ). The values of pH, SS and COD of samples in P2 and P4 were lower than that in P0. In addition, both the nutrient elements (N, TP, Ca, Mg) and harmful elements (Na, Cu, Pb, Cd, AOX) in the wastewater were significantly reduced after the treatment. These results indicated that the RFES was effective in treated the pulp and paper wastewater. They also suggested that the toxic heavy metals and AOX might be retained and accumulated in the wetland soil.
Table 1. Quality of the water samples from the wetland system (mg L−1, except pH).
3.3. Soil pH and electrical conductivity
The soil in the study area was heavily alkaline with the pH values ranging from 8.0 to 8.7 (2014) and 8.3 to 9.1 (2015). In comparison to the control samples (Pck), the pH and electrical conductivity (EC) values of the wetland soil samples were lowered after long-term wastewater irrigation. The pH values of all the samples increased with depth from the topsoil to 80 cm by 0.2–0.4 and 0.11–0.38 for year 2014 and 2015, respectively (Figure (a), (c)). Because the pH of wastewater was slightly lower than the soils, wastewater irrigation for 6–13 years had slightly decreased the soil pH (as compared to the Pck), and the largest decrease was observed in the P0 soil, which was the abandoned stabilization pond for wastewater (Figure (a), (c)). This was probably due to the high concentrations of chemical substances such as ammonium in the wastewater, which moved from the surface to the depth.[Citation23] It has been reported in the literature that long-term use of wastewater effluents may also increase the soil pH due to the high concentration of basic cations such as Na, Ca, and Mg in the water.[Citation24] The EC values of the soil samples varied from 1525 μS cm−1 to 4148 μS cm−1 (2014) and from 1066 μS cm−1 to 2258 μS cm−1 (2015). With an increase in the depth, the EC increased by 8.5–31.0% (2014) and 2.6–22.9% (2015) compared with depth of 0–20 cm (Figure (b), (d)). In comparison to that of the Pck, the EC of the wetland soil samples (P1–P6) increased significantly by 14.3–67.2% (2014) and 1.4–52.7% (2015). The EC values of the wastewater pond soil (P0), however, were generally lower than that of the Pck. These results reflected that the increased salinity of the wetland soil was due to wastewater irrigation. The decrease in the pH and EC in P0 soil might be attributed to high levels of dissolved organic matter in the original wastewater. The soil pH had strong correlation with EC (p < 0.01, pH = 8.09 + 0.0001582 × EC; p < 0.01, pH = 9.362959 − 0.000405 × EC), suggesting that the pH values of the wetland soils might be affected by the irrigation of the wastewater that contained high level of salt.
3.4. Total P, N, SOM, and change in CEC in the soils
The peak values of the total N and P of the soil samples were generally observed in the top soil, and the lowest values were generally observed at a depth of 60–80 cm (Figure ). Moreover, the N and P contents were higher with an increase in the duration of irrigation, especially in the 0–20 cm, indicating the pulp and paper wastewater irrigation might improve the soil fertility in some way.[Citation16] The concentrations of N and P were significantly higher when the duration of irrigation was >10 years as compared with 6–9 years (Figures (a), (b) and (a), (b)).
CECs of the P0, P1, P2, P3, P4, P5, and P6 soils were 5.4–24.4% (0–20 cm, 2014) and 17.8–41.3% (0–20 cm, 2015), 14.0–23.7% (20–40 cm, 2014) and 30.6–46.3% (20–40 cm, 2015), 1.3–26.0% (40–60 cm, 2014) and 30.7–48.3% (40–60 cm, 2015), and 2.9–29.0% (60–80 cm, 2014) and 23.8–43.2% (60–80 cm, 2015), which all were higher than that of Pck (Figures (c) and (c)). The origin of these distinctly different behaviors might be due to the difference in charge distribution and presence of Fe coatings.[Citation25] The underlying mechanisms are still unclear, however, the presence of surface coatings might play an important role.
Irrigation with pulp and paper wastewater had significantly increased the SOM content in the top soils compared to the soils that were not irrigated with wastewater (Pck) (Figures (d), (d)). The SOM contents in P0, P1, P2, P3, P4, P5, and P6 soils were 5.5–47.9% (0–20 cm, 2014) and 55.8–73.1% (0–20 cm, 2015), 7.2–57.1% (20–40 cm, 2014) and 29.1–64.2% (20–40 cm, 2015), 3.6–105.3% (40–60 cm, 2014) and 33.6–64.2% (40–60 cm, 2015), and 10.3–93.4% (60–80 cm, 2014) and 6.9–58.6% (60–80 cm, 2015), which were all higher than that of Pck. Previous studies have shown that long-term irrigation with wastewater increases the SOM contents directly or indirectly and improves the soil nutrient conditions,[Citation26,27] which can be attributed to the high COD in the wastewater. Alvarez-Bernal et al. [Citation28] also reported that irrigation using water from a river that receives wastewater from tanneries for >25 years significantly increases SOM contents in the topsoil.
In this work, the wastewater contained high levels of COD and showed large differences in nutrient concentrations among different sites in the canals. The highest concentrations of nutrients (N, and TP) were observed in the water samples from P2 and P4, and the same trend was also observed with regard to SS, which may lead to its accumulation in the soil of the wetland. The high organic load of the wastewater resulted in an increase in the level of SOM in most of the soils irrigated with wastewater. Furthermore, the longer the duration of continuous irrigation of wastewater, the higher the levels of concentration of SOM in the P1–P6 soils. The slight reduction in the levels of SOM in the deep soils might be partly attributed to the uptake by reed.[Citation29] These results suggested that the accumulation of SOM from wastewater irrigation depended on not only the levels of organic carbon input, but also the rate of plant root consumption.
3.5. Principal component analysis on soil factors
The accumulation of heavy metals, TX, and AOX in the wetland soil revealed that wastewater in the wetland deteriorated soil quality, which was related to both soil properties and irrigation time. To further determine the characteristics of heavy metals, TX, and AOX in the soils of the study area, a principal component analysis (PCA) was used for the measured concentrations. The depth, pH, EC, SOM, CEC, total N, and total P were selected to study the long-term impact of wastewater irrigation on the spatial distribution of heavy metals, TX, and AOX. Figure shows the results of the PCA for the distribution of heavy metals, TX and AOX. Four groups were categorized such as group 1 (SOM, CEC, total N, and total P, Cu, Cd, Pb, TX and AOX in 2014) and (SOM, CEC, total N, total P, Cu, Cd, Pb, Zn, TX and AOX in 2015), group 2 (pH and EC in 2014) and (pH in 2015), group 3 (depth in 2014 and 2015), and group 4 (Zn in 2014, EC in 2015). Two principal components (PCs) were obtained, which contributed 78% (2014) and 70.4% (2015) of the variance for explaining 52.4% (factor 1) and 15.6% (factor 2) in 2014 and 58.3% (factor 1) and 12.1% (factor 2) in 2015, respectively. PC 1 mainly expresses the chemical properties of soil of group 1, which impacted the distribution of heavy metals, TX, and AOX in the soil, and the results also showed higher concentrations of heavy metals, TX and AOX with higher SOM and CEC. It thus can be concluded that the soil nutrition, heavy metals and organic chloride pollutants came from the same source, namely the pulp and paper wastewater.[Citation30] On the other hand, PC 2 is dominated by physical properties of soil of groups 2–4, which came from the nature properties of soil. PCA analysis also showed that increase of irrigation time increased pollutant accumulation in the soil and the distribution and concentration of heavy metals and AOX were dependent of chemical and physical properties of the soil and original wastewater quality.
Figure 8. Principal component analysis (PCA) for the evaluation of distribution and concentrations of heavy metals, TX and AOX in 2014 (a) and 2015 (b).
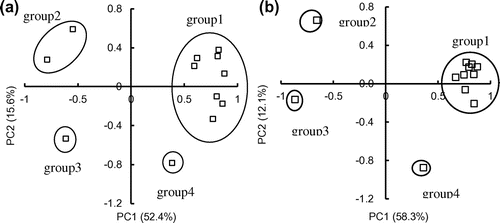
A correlation matrix of soil properties is shown in Table with several consistent results. Heavy metals (Cu, Cd, Pb, Zn) were positively correlated with N, P and SOM content and negatively correlated with depth, pH in the tested two years. SOM concentration showed a positive correlation with N, P concentration, which was greater in the P0 than in the Pck during the two years. Gray et al. [Citation31] also reported that soil properties were negatively correlated with soil microbial biomass carbon and AOX concentration was related with waste water and soil characteristics.[Citation32,33]
Table 2. Correlation matrix of the factors in 2014 and 2015.
4. Conclusions
Long-term irrigation of reed wetland soil with pulp and paper wastewater in the study area improved the soil fertility, but heavy metals, TX and AOX were also accumulated in the soil and posed potential risks. Furthermore, experimental results also showed that the concentrations of heavy metals, TX and AOX in the soils were higher when the wetland was either closer to the wastewater source or irrigated with wastewater for longer time, suggesting both the wastewater quality and irrigation time played important role in affecting the soil quality of the wetland system.
Funding
This study was partially supported by the National Natural Science Foundation of China under [grant numbers 21277115, 41501339], Jiangsu Province Science Foundation for Youths under [grant numbers BK20140468 and BK20130426], Talent Introduction Plan under [grant number kjc2012022], Jiangsu Six Talents Peak Project (2011 Agricultural Fields) grants, and Open Project of Key Laboratory for Ecology and Pollution Control of Coastal Wetlands (Environmental Protection Department of Jiangsu Province) [grant number KLCW1208].
Disclosure statement
The authors declare no conflict of interest.
References
- Becerra-Castro C, Lopes AR, Vaz-Moreira I, et al. Wastewater reuse in irrigation: a microbiological perspective on implications in soil fertility and human and environmental health. Environ Int. 2015;75:117–135.10.1016/j.envint.2014.11.001
- Ndour NYB, Baudoin E, Guissé A, et al. Impact of irrigation water quality on soil nitrifying and total bacterial communities. Biol Fertil Soils. 2008;44:797–803.10.1007/s00374-008-0285-3
- Pedrero F, Kalavrouziotis I, Alarcón JJ, et al. Use of treated municipal wastewater in irrigated agriculture—Review of some practices in Spain and Greece. Agric Water Manage. 2010;97:1233–1241.10.1016/j.agwat.2010.03.003
- Adrover M, Farrús E, Moyà G, et al. Chemical properties and biological activity in soils of Mallorca following twenty years of treated wastewater irrigation. J Environ Manage. 2012;95(Suppl.):S188–S192.10.1016/j.jenvman.2010.08.017
- Gessner TP, Kadlec RH, Reaves RP. Wetland remediation of cyanide and hydrocarbons. Ecol Eng. 2005;25:457–469.10.1016/j.ecoleng.2005.07.015
- Ding C, Li ZX. Adsorption characters of pulping wastewater organic pollutant in the reed wetland soil. Trans. China Pulp Pap. 2008;23:41–45.
- Drewes JE, Jekel M. Behavior of DOC and AOX using advanced treated wastewater for groundwater recharge. Water Res. 1998;32:3125–3133.10.1016/S0043-1354(98)00064-5
- Vinder A, Simonič M. Removal of AOX from waste water with mixed surfactants by MEUF. Desalination. 2012;289:51–57.10.1016/j.desal.2012.01.007
- Ammari TG, Al-Labadi I, Tahboub A, et al. Assessment of unmodified wetland bio-waste: shoots of Cyperus laevigatus, for cadmium adsorption from aqueous solutions. Process Saf Environ Prot. 2015;95:77–85.10.1016/j.psep.2015.02.015
- Tsiridis V, Petala M, Samaras P, et al. Interactive toxic effects of heavy metals and humic acids on Vibrio fischeri. Ecotoxicol Environ Saf. 2006;63:158–167.10.1016/j.ecoenv.2005.04.005
- Shomar B. Sources of adsorbable organic halogens (AOX) in sludge of Gaza. Chemosphere. 2007;69:1130–1135.10.1016/j.chemosphere.2007.03.074
- Savant DV, Abdul-Rahman R, Ranade DR. Anaerobic degradation of adsorbable organic halides (AOX) from pulp and paper industry wastewater. Bioresour Technol. 2006;97:1092–1104.10.1016/j.biortech.2004.12.013
- Ding C, Chen T, Li Z, et al. Assessing and monitoring the ecotoxicity of pulp and paper wastewater for irrigating reed fields using the polyurethane foam unit method based on monitoring protozoal communities. Environ Sci Pollut Res. 2015;26:1–11.
- NY/T 1377-2007. Determination of pH in soil. 2007.
- Lu RK, Zhu HZ, He PA, et al. Methods of inorganic pollutants analysis. In: Soil and agro-chemical analysis methods. Beijing: Agricultural Science and Technology Press; 2000. p. 205–266.
- Wang W, Wang H, Zu Y. Temporal changes in SOM, N, P, K, and their stoichiometric ratios during reforestation in China and interactions with soil depths: importance of deep-layer soil and management implications. For Ecol Manage. 2014;325:8–17.10.1016/j.foreco.2014.03.023
- Ndour NYB, Baudoin E, Guissé A, et al. Impact of irrigation water quality on soil nitrifying and total bacterial communities. J Hazard Mater. 2008;44:797–803.
- Olesky-Frenzel J, Wischnack S, Jekel M. Application of Ion-Chromatography for the Determination of the Organic-Group Parameters AOCl, AOBr and AOI in Water. Fresenius J Anal Chem. 2000;366:89–94.
- GB 6920-1986. Water quality; determination of pH value; glass electrode method. 1986.
- GB 11901-1989. Water quality; determination of suspended substance; gravimetric method. 1989.
- GB/T 11914-1989. The determination of chemical oxygen demand; dichromate method. 1989.
- Zhang C, Liao X, Li J, et al. Influence of long-term sewage irrigation on the distribution of organochlorine pesticides in soil–groundwater systems. Chemosphere. 2013;92:337–343.
- Liang Q, Gao R, Xi B, et al. Long-term effects of irrigation using water from the river receiving treated industrial wastewater on soil organic carbon fractions and enzyme activities. Agric Water Manage. 2014;135:100–108.10.1016/j.agwat.2014.01.003
- Schipper LA, Williamson JC, Kettles HA, et al. Impact of land-applied tertiary-treated effluent on soil biochemical properties. J Environ Qual. 1996;25:1073–1077.10.2134/jeq1996.00472425002500050020x
- Favre F, Bogdal C, Gavillet S, et al. Changes in the CEC of a soil smectite–kaolinite clay fraction as induced by structural iron reduction and iron coatings dissolution. Appl Clay Sci. 2006;34:95–104.10.1016/j.clay.2006.04.010
- Mancino CF, Pepper IL. Irrigation of turfgrass with secondary sewage effluent: soil quality. Agron J. 1992;84:650–654.10.2134/agronj1992.00021962008400040023x
- Kiziloglu FM, Turan M, Sahin U, et al. Effects of untreated and treated wastewater irrigation on some chemical properties of cauliflower Brassica olerecea L. var. botrytis; and red cabbage Brassica olerecea L. var. rubra; grown on calcareous soil in Turkey. Agric Water Manage. 2008;95:716–724.10.1016/j.agwat.2008.01.008
- Alvarez-Bernal D, Contreras-Ramos SM, Trujillo-Tapia N, et al. Effects of tanneries wastewater on chemical and biological soil characteristics. Appl Soil Ecol. 2006;33:269–277.10.1016/j.apsoil.2005.10.007
- Kayikcioglu HH. Short-term effects of irrigation with treated domestic wastewater on microbiological activity of a Vertic xerofluvent soil under Mediterranean conditions. J Environ Manage. 2012;102:108–114.10.1016/j.jenvman.2011.12.034
- Wiseman CL, Zereini F, Püttmann W. Metal and metalloid accumulation in cultivated urban soils: a medium-term study of trends in Toronto, Canada. Sci Total Environ. 2015;538:564–572.10.1016/j.scitotenv.2015.08.085
- Gray DM, Swanson J, Dighton J. The influence of contrasting ground cover vegetation on soil properties in the NJ pine barrens. Appl Soil Ecol. 2012;60:41–48.10.1016/j.apsoil.2012.06.007
- Sharma A, Thakur VV, Shrivastava A, et al. Xylanase and laccase based enzymatic kraft pulp bleaching reduces adsorbable organic halogen (AOX) in bleach effluents: a pilot scale study. Bioresour Technol. 2014;169:96–102.10.1016/j.biortech.2014.06.066
- Deshmukh NS, Lapsiya KL, Savant DV, et al. Upflow anaerobic filter for the degradation of adsorbable organic halides (AOX) from bleach composite wastewater of pulp and paper industry. Chemosphere. 2009;75:1179–1185.10.1016/j.chemosphere.2009.02.042