Abstract
Purpose: The premature chromosome condensation (PCC) technique is used to study exposure to external radiation through the determination of chromosome fragments observed in interphase cells. The presence of large telomeric signals in CHO cells interferes with the detection of PCC fragments and the identification of dicentric chromosomes. We present an improved method for the fusion of G0-lymphocytes with mitotic Akodon cells (few chromosomes and weakly-staining telomeric sequences) to induce PCC in combination with rapid quantification of dicentric chromosomes and centric rings as an alternative to the classical CHO cell fusion technique.
Materials and methods: Whole blood from three healthy volunteers was γ-irradiated with 0, 2, or 4 Gy. Following a 24 h incubation post-exposure at 37 °C, chromosome spreads of isolated lymphocytes were prepared by standard PCC procedures using mitotic Akodon cells.
Results: The percentage of scorable fusions, measured by telomere/centromere (T/C) staining, for Akodon-induced PCC was higher than that for CHO-induced PCC, irrespective of radiation exposure. Importantly, both techniques gave the same result for biodosimetry evaluation.
Conclusion: The mitotic Akodon cell-induced PCC fusion assay, in combination with the scoring of dicentric chromosomes and rings by T/C staining of G0-lymphocytes is a suitable alternative for fast and reliable dose estimation after accidental radiation exposure.
Introduction
The conventional colcemid-block protocol has been used popularly for analyzing chromosomes. Over decades, the premature chromosome condensation (PCC) technique has been greatly improved for preparation and analysis as a tool for genotoxic studies and biodosimetry. The reference technique consists of fusing mitotic cells with interphase nuclei. Peripheral blood lymphocytes (PBL) are widely used to assess genetic damages in humans because they circulate throughout the body, are easy to collect by non-invasive methods, and are largely available in the pre-DNA synthesis (G0) stage, which makes them appropriate for evaluating the effects of exposure to various environmental agents. In contrast to the ‘gold standard’ cytogenetic approach (dicentric chromosome analysis in metaphase), PCC allows the immediate processing of non-dividing cells and the visualization of interphase nuclei in a condensed chromosome form. Because of this unique property, PCC is extensively used for studies related to genotoxic assays, cell cycle analysis, and the investigation of chromatin condensation dynamics, radiation induced damage, and DNA repair mechanisms (Gotoh and Durante Citation2006).
The biodosimetry protocol for PCC of interphase chromosomes from human lymphocytes was initially described by Pantelias (Citation1986). The induction of PCC in PBLs is provoked by fusion with mitotic Chinese hamster ovary (CHO) cells using polyethylene glycol, thus enabling the direct analysis of radiation-induced chromosomal aberrations in non-stimulated G0 lymphocytes. The main advantage of PCC is the rapid quantification of exposure by counting the PCC fragments after Giemsa staining (Pantelias and Maillie Citation1985a, Citation1985b; Lamadrid Boada et al. Citation2013). Various developments of the technique have been published, such as the PCC assay in combination with C-banding (Pantelias et al. Citation1993) or fluorescence in situ hybridization (FISH), to facilitate the detection of dicentric chromosomes and translocations (Darroudi et al. Citation1998). More recently, the T/C peptide nucleic acid (PNA) probe, initially developed for metaphase analysis (M’kacher et al. Citation2014), has been combined with the PCC technique (M’kacher et al. Citation2015; Karachristou et al. Citation2015), facilitating dicentric- and ring-chromosome (Dic + R) detection, as well as that of acentric fragments. However, the technique has limitations due to the presence of large interstitial telomeric repeat sequences (ITS) in the large centromeric region of CHO cells.
Here we report, for the first time, an alternative technique for PCC induction by replacing CHO cells. The identification of a species with few chromosomes and a moderate signal for ITS led us to choose the Akodon species, which is also a rodent, for which the cells in culture become spontaneously immortal. Here, we aimed to evaluate the alternative approach to induce PCC with primary mitotic Akodon fibroblasts. We present the protocol and compare the fusion efficiency with that of classical CHO fusion, as well as a dose estimation after in vitro irradiation of whole blood.
Materials and methods
Blood samples and irradiation
Peripheral blood from three healthy blood donors, average age 36 ± 9.5 years, was collected in heparin tubes with informed consent. Lymphocytes were isolated using the standard Ficoll isolation technique (M’kacher et al. Citation2015) and irradiated with 2 or 4 Gy 137Cs γ rays at a dose rate of 0.76 Gy/min at room temperature (RT). The irradiated samples were maintained at 37 °C in a CO2 incubator for 24 h or until fusion.
Cell culture and PCC fusion
CHO and Akodon (Akodon arviculoides) (collection ‘MNHN-TCCV-007’) cells were grown in DMEM/F12 (1:1) medium supplemented with 10% FCS, 1% L-glutamine, and antibiotics and incubated at 37 °C in a humidified atmosphere with 5% CO2 in monolayer cultures in plastic T75-cm2 flasks. Colcemid (final concentration 0.1 µg/ml) was added to the cell culture to obtain mitotic cells, followed by a further incubation of 4 h at 37 °C, and the accumulated mitotic cells harvested by the shake-off method. The mitotic cells were maintained on ice until needed for fusion with interphase cells. Cell fusion and the induction of PCC with polyethylene glycol (PEG) were performed as previously described (M’kacher et al. Citation2015). Briefly, mitotic CHO and Akodon cells and non-stimulated (G0) lymphocytes were mixed in 15 ml round-bottom culture tubes in the presence of colcemid. The ratio of lymphocytes/CHO or Akodon cells was 3/1 and 6/1. After centrifugation, the supernatant was discarded and 150 µl 50% (w/v) PEG prepared in PBS was gently added by inverting the tubes. Subsequently, 1.5 mL of RPMI-1640 medium without FBS and complemented with colcemid (0.1 µg/mL) was gently added and the cell suspension was centrifuged. The supernatant was discarded and to initiate the fusion, the cell pellet was re-suspended in 0.7 ml pre-warmed RPMI-1640 medium supplemented with 10% FCS, colcemid (0.1 µg/mL), and 2% phytohemagglutinin and incubate for 90 min at 37 °C. The harvesting, fixation, and chromosome spreads were performed according to standard cytogenetic procedures.
Staining with PNA probes
Centromere and telomere staining of PCC lymphocytes was performed by FISH using a Cy3-labeled PNA probe specific for telomere sequences and a FAM-labeled PNA probe specific for centromere sequences (Panagene, South Korea). Briefly, the stored slides were maintained at RT overnight, washed in phosphate buffered saline (PBS) for 5 min, fixed in 4% formaldehyde for 2 min, washed twice again in PBS for 5 min, and digested with a pre-warmed pepsin solution (1 mg/ml) for 3 min at 37 °C, and re-fixed with 4% formaldehyde for 2 min. After washing three times with PBS, the slides were dehydrated with 50, 70, and 100% ethanol for 5 min each and air dried for 1 h at RT. PNA probes for C/T staining were applied, co-denatured for 3 min at 80 °C, and the slides incubated for 2 h in a humidified chamber at RT in the dark. After hybridization, the slides were washed as previously described (M’kacher et al. Citation2015) and finally counterstained with DAPI (1 µg/ml) and anti-fade mounting medium.
Image capture and analysis
The PCC fusions were automatically scanned and the images acquired using MSearch software (MetaSystems, Germany). Images of the T/C-stained PCC fusions were captured using automated acquisition module Autocapt software (MetaSystems, version 3.12.7) and a Zeiss Plan-Apochromat 63X/1.40 oil and CoolCube 1 Digital High-Resolution CCD Camera (Carl Zeiss, Germany). The captured gallery was exported as a series of RGB bitmap images and the images analyzed with IMAGE J and a lab-developed plugin (M’kacher et al. Citation2015).
Statistical analysis
A ‘t’ test was performed using online Graphpad QuickCalcs software (San Diego, CA) to compare the mitotic Akodon and CHO cell-induced PCCs.
Results
Analysis of mitotic CHO cell-induced PCC
Mitotic CHO cell-induced PCCs were first stained with Giemsa ( left), followed by T/C staining ( right) for comparison. PCC analysis is generally performed with Giemsa staining for fragment counting but we previously demonstrated that the staining of telomeres and centromeres allowed the detection of chromosomal aberrations and not only the PCC fragments exceeding 46 (M’kacher et al. Citation2015). However, the detection of T/C-stained PCC fragments can be challenging because adjusting the telomere intensity to that of CHO chromosomes does not allow human telomere visualization ( center), whereas adjusting the intensity to that of human telomeres leads to very intense CHO ITS ( right). T/C PNA staining of CHO chromosomes resulted in high-intensity staining of ITS in the centromeric regions ( left), which is not suitable for analysis when human chromosomes are mixed among CHO chromosomes ( right).
Figure 1. Comparison of CHO and Akodon-induced PCC of human PBLs. a. The CHO-induced PCCs are visualized by Giemsa (left) or T/C PNA probe staining, resulting in a low-intensity signal for telomere capture (middle) or adjusted intensity (right), allowing human telomere visualization. b. Staining of CHO metaphase chromosomes and CHO-induced PCCs with T/C PNA probes. c. Staining of Akodon metaphase chromosomes and Akodon-induced PCC by T/C PNA probes.
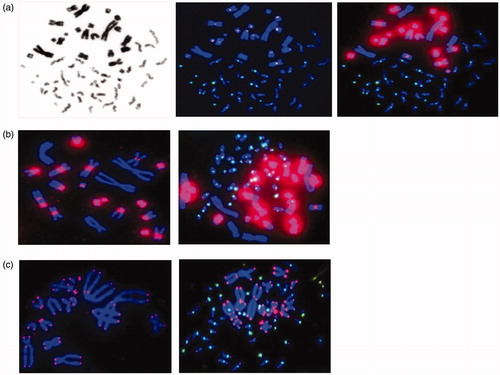
Standardization of mitotic Akodon cell-induced PCC
We sought another species suitable for fusing with interphase PBLs to avoid the interference of T/C staining of CHO chromosomes. The identification of a rodent with fewer chromosomes (n = 14) and no large ITS in the centromeric region led to the selection of the Akodon species (Akodon arviculoides) (). Cell cultures of primary fibroblasts were performed until the obtention of an immortal cell line, which is a prerequisite for obtaining a large number of mitotic cells. Mitotic Akodon cell-induced PCC was standardized and the slides stained with T/C PNA probes () for observation and analysis.
Qualitative and quantitative analysis of PCC obtained from CHO and Akodon cells
We compared CHO- and Akodon-induced PCC by calculating a PCC index. Overall, PCC images were classified into three sub-groups among the total number of metaphase images to determine this index (): ‘total fusions’ (left), ‘scorable fusions’ (center), and ‘complete fusions, with the presence of the 46 centromeres’ (right).
Figure 2. Illustration of the PCC index calculation. a. Images of PCCs obtained by fusion with CHO cells b. Images of PCCs obtained by fusion with Akodon cells. a.b. The PCC fusions are classified into three groups: total fusions (left panels), scorable fusions (middle panels), or PCCs with 46 human centromeres (right panels). c. The graph shows the percentage of PCC fusions displaying 46 human centromeres among the total fusions captured. Three slides were counted and the standard deviation is shown.
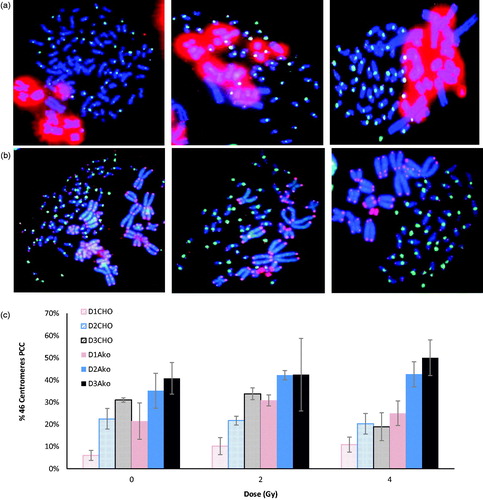
Blood samples were exposed to 2 and 4 Gy Cs-137 γ-rays or sham irradiated. The irradiated cells of the three donors were pooled and used for further analysis to minimize inter-individual variation in the PCC yields. The observed average total fusions were 2.2%, 2.1%, and 2.3% for CHO mitotic cells and 0.7%, 0.6%, and 0.3% for Akodon mitotic cells (not presented) for 0, 2, and 4 Gy, respectively. Although the percentage of PCC fusions was lower for Akodon mitotic cells, the percentage of scorable fusions was the converse, with 42.4%, 41.5%, and 35.5% for mitotic CHO cells and 69.5%, 71.8, and 71.2% for mitotic Akodon cells for 0, 2, and 4 Gy, respectively (). Finally, the percentage of ‘complete fusion’ was higher for Akodon cells ().
Table 1. Comparison of CHO and Akodon-induced PCC.
Analysis and comparison of γ-irradiated mitotic Akodon and CHO cell-induced PCC
The PCCs obtained with CHO and Akodon cells were scored for Dic + R detection (). The observed Dic + R frequencies were not significantly different between mitotic Akodon and CHO cells (p > .05). Akodon cells can be considered as an alternative of the PCC technique for biodosimetry and will facilitate analysis.
Figure 3. Validation of mitotic Akodon cell-induced PCC. PCCs were obtained from the fusion of human peripheral blood samples, exposed in vitro to γ-radiation, with Akodon or CHO cells. Dicentric and ring chromosomes were counted after T/C PNA staining. The observed difference was not statistically significant (p > 0.05).
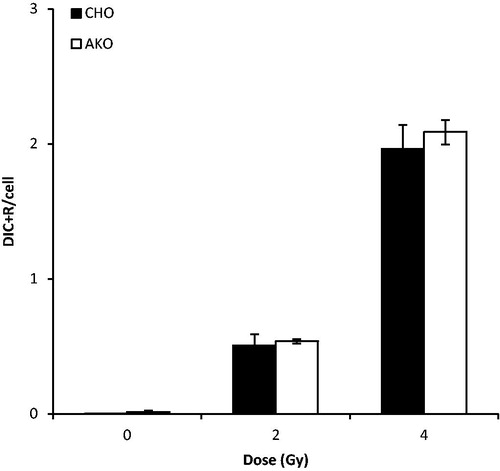
Discussion
In biodosimetry, the dicentric-chromosome assay is the ‘gold standard’ because of its specificity and sensitivity to assess an absorbed dose during exposure to ionizing radiation in the absence of physical dosimetry (IAEA Annual Report for 2011 | CitationIAEA). During an unexpected mass casualty event, the time required to obtain metaphases by culturing whole blood for two days is still the major hurdle for attaining a rapid dose estimate. The immediate induction of PCC in interphase lymphocytes allows rapid scoring of radiation-induced chromosomal aberrations, but the difficulty of the technique and subsequent analysis of the data restrict its use. Various endpoints have been recommended: the frequency of chromosome rings (Lamadrid et al. Citation2007), the mixture of the total number of chromosomes and the G2-PCC index (Gotoh et al. Citation2005), the dimension ratio between the shortest and longest chromosome (Gotoh & Durante Citation2006), and the yield of additional chromosome pieces (Balakrishnan et al. Citation2010). The frequency of PCC rings has been reported to increase for doses of up to 20 Gy, indicating its suitability for dose assessment after high-dose radiation exposure (Puig et al. Citation2013).
The classical staining procedure used to count the total number of chromosome fragments for PCC scoring in biodosimetry is Giemsa (Lamadrid Boada et al. Citation2013). The mitotic CHO cell-induced PCC technique has been standardized, harmonized, and validated world-wide by an inter-laboratory biodosimetry comparison, although it was restricted to the few experts in the field (Terzoudi et al. Citation2017).
The development of C/T PNA probes and their simultaneous use in lymphocyte PCC in combination with FISH has offered a new life to the PCC method, enabling the analysis of Dic + R chromosomes and acentric fragments directly in non-stimulated lymphocytes, with a level of accuracy and ease not previously possible (M’kacher et al. Citation2015).
Although the analysis of PCC in fused cells with C/T PNA probes should make the detection of Dic + R easier, the high intensity of hamster ITS often masks a part of the human chromosomes. No biodosimetry assay can yet be used as a stand-alone tool to meet the requirements of time-efficiency, accuracy, and throughput. Here, we present a new technique to perform Akodon-induced PCC to provide an attractive alternative to classical CHO-induced PCC. Unlike PCC using CHO cells, mitotic Akodon cell-induced PCC did not exhibit an intense signal at the telomeric regions, which made it easy to visualize and score all human chromosomes (Fagundes et al. Citation1997). With the use of Akodon cells, the scoring of excess Dic + R chromosomes, as well as acentric fragments, can become a reliable and rapid method for triage biodosimetry (Karachristou et al. Citation2015). Although the calculated PCC fusion index was smaller in mitotic Akodon cells, the frequency of scorable and complete fusions (46 centromeres) was relatively high compared to that of PCC using mitotic CHO cells. This observed difference may be due to T/C staining, which gives a normal staining pattern in mitotic Akodon cells. The frequency of Dic + R obtained for different doses of irradiation was comparable between Akodon and CHO-induced PCC, which also shows that mitotic Akodon cells are suitable for inducing PCC in irradiated PBLs. This is the first study to use Akodon cells for PCC fusion and our results show that they can be used for the standardized PCC protocol.
Acknowledgement
The authors are in debt to Michèle Gerbault-Sereau for the Akodon cells (MNHN Cell bank collection "MNHN-TCCV-007") and Bernard Dutrillaux for his useful advice on the choice of species. The authors gratefully thank William Hempel for his valuable contribution for the re-reading and the correction of the manuscript.
Disclosure statement
The authors declare no potential conflict of interest.
Additional information
Funding
Notes on contributors
Tamizh Selvan Gnana Sekaran
Tamizh Selvan Gnana Sekaran, was a Postdoctoral fellow at PROCyTox, where he developed cytogenetic approaches. He has now a permanent position at K S Hegde Medical Academy, NITTE (Deemed to be University), Mangalore, India.
Michelle Ricoul
Michelle Ricoul, is a Senior Scientist at CEA and is the head of PROCyTox dedicated to genotoxic studies. Her main field of interest is the study of chromosomal instability and the development of new techniques. She is highly involved in biodosimetry approaches.
Patricia Brochard
Patricia Brochard, is a well-experienced technician at PROCyTox, she is expert in cytogenetics assays and analysis. She is also skilled in virology and immunology on primate models.
Cecile Herate
Cecile Herate, is a postdoctoral fellow in radiobiology. She is expert in molecular and cellular biology, immunology and virology. She recently acquired expertise in molecular cytogenetics.
Laure Sabatier
Laure Sabatier, is a Research Director and has been head of the Laboratory of Radiobiology and Oncology in CEA (1996-2015). She is now the deputy-director of the programs division at DRF Toxicology Program. Her main interest is to study telomere maintenance and the role of chromosome instability in multistep carcinogenesis.
References
- Balakrishnan S, Shirsath K, Bhat N, Anjaria K. 2010. Biodosimetry for high dose accidental exposures by drug induced premature chromosome condensation (PCC) assay. Mutat Res. 699:11–16.
- Darroudi F, Fomina J, Meijers M, Natarajan AT. 1998. Kinetics of the formation of chromosome aberrations in X-irradiated human lymphocytes, using PCC and FISH. Mutat Res. 404:55–65.
- Fagundes V, Vianna-Morgante AM, Yonenaga-Yassuda Y. 1997. Telomeric sequences localization and G-banding patterns in the identification of a polymorphic chromosomal rearrangement in the rodent Akodon cursor (2n = 14,15 and 16). Chromosome Res Int J Mol Supramol Evol Asp Chromosome Biol. 5:228–232.
- Gotoh E, Durante M. 2006. Chromosome condensation outside of mitosis: mechanisms and new tools. J Cell Physiol. 209:297–304.
- Gotoh E, Tanno Y, Takakura K. 2005. Simple biodosimetry method for use in cases of high-dose radiation exposure that scores the chromosome number of Giemsa-stained drug-induced prematurely condensed chromosomes (PCC). Int J Radiat Biol. 81:33–40.
- IAEA Annual Report for 2011 | IAEA; 2012 [cited 2019 Jan 31]. Available from: https://www.iaea.org/publications/reports/annual-report-2011
- Karachristou I, Karakosta M, Pantelias A, Hatzi VI, Karaiskos P, Dimitriou P, Pantelias G, Terzoudi GI. 2015. Triage biodosimetry using centromeric/telomeric PNA probes and Giemsa staining to score dicentrics or excess fragments in non-stimulated lymphocyte prematurely condensed chromosomes. Mutat Res Genet Toxicol Environ Mutagen. 793:107–114.
- Lamadrid AI, García O, Delbos M, Voisin P, Roy L. 2007. PCC-ring induction in human lymphocytes exposed to gamma and neutron irradiation. J Radiat Res. 48:1–6.
- Lamadrid Boada AI, Romero Aguilera I, Terzoudi GI, González Mesa JE, Pantelias G, García O. 2013. Rapid assessment of high-dose radiation exposures through scoring of cell-fusion-induced premature chromosome condensation and ring chromosomes. Mutat Res Toxicol Environ Mutagen. 757:45–51.
- M’kacher R, El Maalouf E, Terzoudi G, Ricoul M, Heidingsfelder L, Karachristou I, Laplagne E, Hempel WM, Colicchio B, Dieterlen A, et al. 2015. Detection and automated scoring of dicentric chromosomes in nonstimulated lymphocyte prematurely condensed chromosomes after telomere and centromere staining. Int J Radiat Oncol Biol Phys. 91:640–649.
- M’kacher R, Maalouf EEL, Ricoul M, Heidingsfelder L, Laplagne E, Cuceu C, Hempel WM, Colicchio B, Dieterlen A, Sabatier L. 2014. New tool for biological dosimetry: reevaluation and automation of the gold standard method following telomere and centromere staining. Mutat Res. 770:45–53.
- Pantelias GE. 1986. Radiation-induced cytogenetic damage in relation to changes in interphase chromosome conformation. Radiat Res. 105:341–350.
- Pantelias GE, Iliakis GE, Sambani CD, Politis G. 1993. Biological dosimetry of absorbed radiation by C-banding of interphase chromosomes in peripheral blood lymphocytes. Int J Radiat Biol. 63:349–354.
- Pantelias GE, Maillie HD. 1985a. The measurement of immediate and persistent radiation-induced chromosome damage in rodent primary cells using premature chromosome condensation. Health Phys. 49:425–433.
- Pantelias GE, Maillie HD. 1985b. Direct analysis of radiation-induced chromosome fragments and rings in unstimulated human peripheral blood lymphocytes by means of the premature chromosome condensation technique. Mutat Res. 149:67–72.
- Puig R, Barrios L, Pujol M, Caballín MR, Barquinero J-F. 2013. Suitability of scoring PCC rings and fragments for dose assessment after high-dose exposures to ionizing radiation. Mutat Res. 757:1–7.
- Terzoudi GI, Pantelias G, Darroudi F, Barszczewska K, Buraczewska I, Depuydt J, Georgieva D, Hadjidekova V, Hatzi VI, Karachristou I, et al. 2017. Dose assessment inter-comparisons within the RENEB network using G0-lymphocyte prematurely condensed chromosomes (PCC assay). Int J Radiat Biol. 93:48–57.