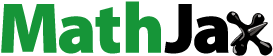
ABSTRACT
This study uses a new approach for the recycling of plant nutrients by co-digesting sewage sludge with fly ash from a wood combustion. Sewage sludge and fly ash both are enriched with nutrients of the wastewater resp. wood, which makes these products an enhanced source for recycled fertilizers. The effects of the ash addition to the anaerobic digestion are studied in several lab scale experiments including effects on the gas production and microbial activity. Following that, the fertilizing qualities of the digestate are evaluated by plant growth experiments. The results show that the fertilizing qualities of the digested sludge were improved by the ash addition. Next to this, gas production results show that the methane production was not affected by the ash addition, while the total gas release was reduced. The sulphur addition by the ash stimulated sulphate reducing bacteria. The sulphate reducing bacteria did not markedly inhibit the methanogens.
GRAPHICAL ABSTRACT
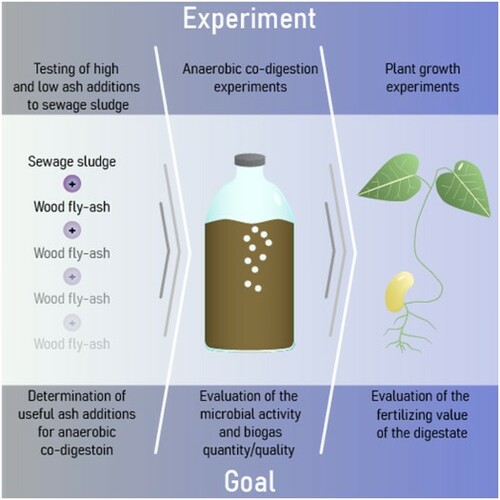
1. Introduction
Although there is no actual limitation in resources of phosphorus and other plant nutrients, it becomes more important to recycle them due to lacks of supplies in the EU and a society focusing on a circular economy [Citation1]. A source with some nutrient content is wastewater. The concentration reaches its maximum in the sewage sludge. Today more than 25 million dry tons of sewage sludge are produced every year [Citation2]. There are several ways of disposing the sewage sludge but only 25% of the nutrients are reused in the EU, mostly by direct application on agricultural soils of the sewage sludge [Citation3]. One of the downsides of direct application is that the fertilizing value of sewage sludge is much lower than the fertilizing value of commercial fertilizers. By combining sewage sludge with a fly ash from a wood combustion and treating them together by anaerobic digestion, the resulting sludge is expected to have a better fertilizing value. This is measured in this study by analysing the growth response of higher plants to additions of the resulting sludge.
Further goals of this study are to find out how the gas production and biological activity are affected by the ash addition. Since no comparable research was done before, this project focuses on a preliminary investigation on the process, with the aim to find advantages and limits to show if the field of research could be interesting for further projects and processes. Other problems of direct application of sewage sludge to agricultural soils, like pathogen, heavy metal or micro plastic contamination, were not part of this study.
2. Methods
2.1. Materials
The sludge was taken from Luleå sewage treatment plant in Sweden. The plant has an activated sludge treatment with pre- and post-settling and a post nitrogen treatment by using immobilized bacteria on plastic carriers. Two different types of sludge are used in a mixture during the experiments. The thickened sludge is a mix of primary and secondary sludge entering the digester, which has substrate and microbes as inoculum. To enhance the gas production rate and to get faster results, thin sludge from the outflow of the digester was added to the thickened sludge as additional inoculum. Three sludge samples were collected at different times to provide fresh sludge to every experiment. The average totals solids (TS), volatile solids (VS) and chemical oxygen demand (COD) of both sludge types is given in .
Table 1. TS, VS and COD of the thickened and digested sludge from Luleå sewage treatment plant from a sample taken after performing the experiments.
Since ash properties depend on feedstock and process conditions, the choice of the right ash can be crucial [Citation4]. In this project, a fly ash was chosen because fly ashes are enriched in inorganic substances like fertilizing compounds (e.g. K, P, S) but also in heavy metals [Citation5]. In contrast to that, bottom ashes mostly consist out of silicates. Although most fly ashes still contain less than 5% P2O5, some ashes from phosphorus rich feedstocks like sewage sludge fly ashes can contain up to 30% P2O5 [Citation6–8]. The fly ash in this project comes from a wood residue combustion at Dåva 2 incineration plant, located at the city of Umeå, Sweden. The plant has a fluidized bed reactor for production of electricity and district heat [Citation9]. The content of fertilizing compounds as well as other trace elements are shown in .
Table 2. Elemental analysis of the used fly ash.
2.2. Initial screening tests
In the screening tests, different influencing factors of ash additions to an anaerobic digestion were tested simultaneously. These included stirring, different ash additions (0.4 and 1.0 gash/gVS) and additions of neutralized ash leachate to measure the effect of trace elements in the ash without pH and particle addition effect. The leachate was produced in a batch leaching test with a liquid to solid ratio of 5. The following neutralization was done by addition of sulphuric acid. The overall leaching procedure was performed according to [Citation10]. The leachate was added to the experiments in amounts which corresponded to the ash additions (equivalent to 0.4 and 1.0 gash/gVS resulting in 2 and 5 mLNS/gVS). The additions with pH effect were chosen with the goal to not exceed pH 9 to stimulate the acidogenic bacteria but not inhibit methanogens totally [Citation11]. Since the acidogenesis lowers the pH by formation of organic acids, the methanogenic activity could be restored after a couple of days. Next to a change in pH, the ash also adds trace elements to the anaerobic digestion. These trace elements can improve the bacterial growth in low amounts but also inhibit it in higher amounts. For cations like Na+, K+, Ca2+ and Mg2+ inhibition has been observed already when concentrations between 1000 and 3500 mg/l are reached [Citation12]. For sulphides inhibition begins when concentrations reach 200 mg/l [Citation12]. All additions were chosen with the goal not to exceed levels with likely inhibitions. The overall procedure of the screening tests accords to [Citation13] with modifications.
The tests were made using septum flasks of 100 mL volume in a heating cabinet at 35°C. To check the effects of salinity an experiment with the sludge mix and an addition of 14% NaCl in mass compared to VS was set up. The amount of NaCl corresponds to the conductivity of the experiments with the highest ash and leachate addition. All experiments were performed in triplicates with an additional control experiment without ash or leachate addition. The gas production was measured daily, the composition of the gas was analysed after one week of digestion by gas chromatography. After 18 days, one flask of each experiment was opened and the sludge/ash mixture was analysed (pH, conductivity, TS and ash content resp. VS). After four weeks, the rest of the flasks were opened and analysed as before specified. Flasks with promising gas production were chosen for elemental analyses. Next to the elemental analyses fluorescence in situ hybridization analyses of the micro flora were performed on a sample of each experimental setting. Based on the screening tests settings were chosen that were used to produce samples for fertilizing tests.
2.3. Microbial analysis
Samples were diluted and then fixed with 3% paraformaldehyde overnight at +4°C. Then they were washed three times with PBS (phosphate buffer saline 130 mM NaCl, 10 mM Na2HPO4, pH 7.2) and stored in 50% ethanol solution at −18°C until analysis. Before the FISH (fluorescent in-situ hybridization) procedure samples were additionally homogenized by passing through a thin pipet tip (1–200 μL) 50–80 times. The FISH procedure was performed according to Seviour and Nielsen (2014) [Citation14]. ARC915 was used as a general probe for methanogens [Citation15]. Additionally DAPI (4′,6-diamidino-2-phenylindole) dye was used to count total number of microbes. Diaphot-300 epifluoresence microscope (Nikon, Japan) with filter sets (Chroma Tech, USA) was used with 1000× magnification.
2.4. Mass balances and production of fertilizer
To produce a larger amount of sludge/ash mixture as a fertilizer for plant experiments, three flasks of 2 L volume were set up with approx. 1 kg of sludge mix each. To the sludge mix ash amounts of 0.4 gash/gVS (experiment SAL) and 0.7 gash/gVS (experiment SAH) were added. Experiment S was run without ash addition. The experimental setup from the screening tests was changed to the bigger flasks with attached gasbags. The temperature in the heating cabinet was kept at 35°C, as in the screening tests. The gas composition was measured once per week by gas chromatography. The amount of gas was measured by emptying the gas bags with a glass syringe after each week.
2.5. Plant growth experiments to evaluate fertilizer
For the plant growth experiments the sludge was thickened in a centrifuge to approx. 15% TS and the clear phase was separated by decanting, since dewatering is an important step in sludge treatment according to [Citation16]. Sand was used as a substrate for the plants. The sand was mixed with the thickened sludge in amounts of 2%, 4% and 6% sludge to sand mass. Water was added in an amount of 20% water to sand mass. Each pot contained approx. 500 g of sand/sludge/water mix and four white beans as seeds. The beans were kept 24 h in the refrigerator for vernalization and 4 h in deionized water for the imbibition phase before planting. All experiments were performed in triplicates and for the three types of sludge. Water was added every day by compensating the weight loss compared to the beginning.
The plant experiments were evaluated by different categories after 32 days of growth, i.e. the number of sprouts per pot, accumulated plant length per pot, plant weight per pot, root weight per pot and leaf area per pot. All data of the evaluation was analysed with the experimental design software MODDE 11.0.1 by Umetrics.
The amounts of sludge were chosen by measuring the electrical conductivity of the sand/sludge/water mix [Citation17]. Since beans are showing fast results in growing and are on the other hand sensitive to salinity, they were chosen as plants for the test. A nutrient free sand was used as substrate.
3. Results and discussion
3.1. Screening tests
The screening tests showed that high ash additions (1.0 gash/gVS) will inhibit the methanogens totally. Therefore further experiments were continued with ash additions of 0.4 or 0.7 gash/gVS. It was also observed that stirring had only a minor effect on the experiments, which led to the conclusion to not apply stirring in later experiments. The addition of leachate as a source for minerals or trace elements had no major effect on the experiments, which also led to the conclusion not to add leachate in later experiments. The small effect of the leachate addition could have been caused by different process conditions in the leaching procedure in comparison to the in situ nutrient mobilization conditions. The anaerobic digestion experiments with addition of NaCl showed similar gas productions like the control experiments. This means that within the examined range of ash additions, salinity (based on electric conductivity measurement) was no limiting factor for the microbes.
3.2. Microbial activity and mass balances
The gas produced in the anaerobic digestions showed a higher methane to carbon dioxide ratio (CH4/CO2) when the experiments had an ash addition, which was expected due to the increased alkalinity. The average CH4/CO2-ratios in the biogas are shown in . In addition, the hydrogen sulphide concentrations in the biogas were elevated in the ash added tests, which indicates that sulphate-reducing bacteria were stimulated by the sulphate contained in the added ash. Since the methane to carbon dioxide ratio still increased, the alkalinity elevation was over-riding the added carbon dioxide release from sulphate reducing bacteria.
Table 3. Gas analyses showing the methane to carbon dioxide ratio and hydrogen sulphide content of produced biogas in experiments with and without ash addition.
Sulphate is reduced to sulphide, which is present in the liquid and gas phase as hydrogen sulphides (see ). At elevated concentrations, hydrogen sulphide can cause problems in the usage of the gas and it may inhibit the methane production when concentrations in the liquid phase reach more than 150 mg/l of HS− [Citation18]. Since methanogens and sulphate reducing bacteria both use organic material as electron donor, they are competing. Since methane production was not affected in the tested range (), the concentrations of hydrogen sulphide did apparently not inhibit the methanogens and the sulphate reducing bacteria were not outcompeting the methanogens. In conclusion, the overall degradation of organic material seem to have increased due to the action of sulphate reducers and methanogens in combination.
Figure 1. Accumulated methane production of the thickened sludge of two screening-test experiments as examples.
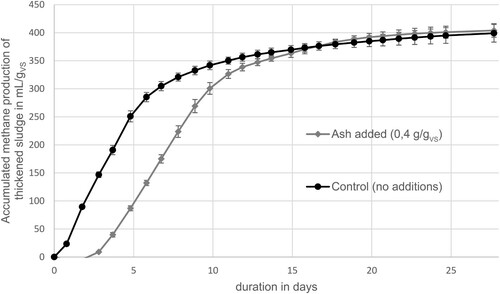
The results of the gas compositions are also supported by fluorescent in situ hybridization (FISH) analyses. The analyses are based on material from the screening tests and show a share of 15% methanogens compared to the total number of microbes for the control experiments and 21% methanogens compared to the total microbes for experiments with an ash addition of 0.4 gash/gVS. The total number of methanogens is about the same in experiments with or without ash addition but the number of total microbes is lower when ash was added. This shows that the ash addition does not inhibit the methanogens over the whole duration of the experiments, since the FISH analyses were performed at the end of the experiments.
For ash added experiments, the pH was elevated in the beginning of the experiments. In the end of the experiments, pH levels were comparable to the control experiment and only slightly elevated. The elevated pH levels in the beginning inhibited the methanogens and led to a slower gas production. After approx. 3–5 days, the gas production rate was comparable again to the control experiments due to more suitable pH range for methanogens (see ). On the other hand literature shows that elevated pH levels help to accelerate the acidogenic fermentation and thereby stimulate the production of volatile fatty acids [Citation19]. The ash addition also led to an elevated electric conductivity (EC) through the course of testing. The elevated EC had no effect on the microbes and the gas production, which follows the results of the screening tests with NaCl addition. The pH levels and EC measurements for the major experiments are shown in .
Table 4. pH levels and EC measurements at the beginning and end of experiments with and without ash addition.
The consumption of volatile solids (VS) in all experiments corresponds to gas volumes similar to the measured ones. For some experiments, the measured gas amounts are smaller than the gas volumes calculated by mass balances but gas leakages were already suspected during the experiments. The consumption of VS is for all experiments comparable or slightly better than the process of the wastewater treatment plant where the sludge came from.
3.3. Fertilizer
The plant growth experiments were conducted by using the sludge with different amounts of ash addition in different amounts and evaluation was done with support of multivariate data analyses. Difficulties occurred due to the strong variation of the results (especially sprouting). Despite the variation, the results show that for all responses the dewatered sludge with added ash (SAL and SAH) had better fertilizing properties than the control (S). Thereby low amounts of sludge addition to the growth medium (2% sludge/sand mass) were more beneficial for plant growth. shows a plot from MODDE. The bars show how each factor influenced the average plant in sprouting, plant length, plant weight, root weight and leaf area.
Figure 2. Normalized coefficients of MODDE, showing how different types of sludges affected plant growth.
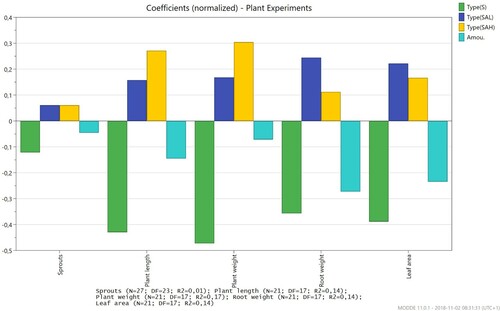
The experimental data of the plant growth experiments gives indications about the ongoing processes during the anaerobic digestion and shows that carbon dioxide was likely transformed to carbonate due to the elevated pH by the ash addition. This makes it likely that due to carbonate formation and elevated pH more potassium phosphate forms instead of calcium phosphate. Calcium and carbonate ions form calcium carbonate, which precipitates and is nearly insoluble at neutral pH-levels. The formed potassium phosphate has a solubility of up to 200 g/100 gwater [Citation20], whereas calcium phosphate is insoluble in water. This could increase the fertilizing value of the sewage sludge, which is normally low due to a low water solubility of phosphorus (1–38% of the contained phosphorus [Citation21]). The pH dependant process follows the following formula in elevated pH levels (formula based on [Citation22,Citation23]):
Although the plant growth experiments show a clear trend, these tests can only be seen as an initial study of the fertilizing properties. The experiments were set up in a way to produce clear results after only a month of plant growth. The long-term impacts and other growth media remains to be tested.
3.4. Outlook
The experiments showed a potential for the treatment of the two waste streams in combination, further studies are necessary for a better understanding of the processes and optimization. These should include the adaption of biological processes in the anaerobic digestion as well as the formation of water-soluble phosphate compounds. In addition, the choice of an ash, which is enriched with desired nutrients and trace elements, can enhance the process further and help designing a valuable final product. More research should also be conducted on separation or immobilization of pollutants in the process.
4. Conclusions
The digestion experiments clearly show that the CO2 release to the gas phase is lower in the ash added experiments in comparison to the control experiments, whereas the methane production remains nearly unchanged in all experiments. This is beneficial for gas upgrading. A downside for gas upgrading processes is the elevated hydrogen sulphide concentration in the biogas of ash added experiments.
The pH, which is elevated in the beginning of ash added experiments but nearly the same at the end of all experiments, shows that the ash addition might affect methanogens in the beginning of the process but not in total. The salinity expressed as electric conductivity was no limiting factor, in the tested ranges. Neither were the methanogens affected by parallel sulphate reduction.
The evaluation of the plant experiments show that dewatered and digested sludge with ash additions has better fertilizing properties than the dewatered and digested sludge without ash additions, thus the fertilizing value of sewage sludge was improved by the ash addition.
Applied in full scale the process could potentially result in a positive resource balance, given that a suitable ash is available and THAT the fertilizing properties can be used.
Acknowledgements
The work has been carried out under the auspices of Graduate School in Energy Systems, financed by the Swedish Energy Agency. Economic support from the Swedish Research Council Formas, within the national research programme Sustainable Spatial Planning, is also gratefully acknowledged (dnr. 2018-00194).
Disclosure statement
No potential conflict of interest was reported by the author(s).
Additional information
Funding
References
- U.S. Geological Survey. Mineral commodity summaries 2017. Reston (Virginia): Geological Survey; 2017.
- Mateo-Sagasta J, Raschid-Sally L, Thebo A. Global wastewater and sludge production. Treatment and Use. 2015;8:15–38.
- LeBlanc RJ, Matthews P, Richard RP. Global atlas of excreta, wastewater, sludge and biosolids management: moving forward the sustainable and welcome uses of a global resource. Nairobi; 2008.
- Ribbing CM, Bjurström HG. The Swedish ash programme with focus on bioashes: ashes are a resource in a sustainable society. In: Insam H, Knapp BA, editors. Recycling of biomass ashes. Berlin, Heidelberg.: Springer; 2011; p. 147–164.
- Dahl O, Nurmesniemi H, Pöykiö R, et al. Comparison of the characteristics of bottom ash and fly ash from a medium-size (32 MW) municipal district heating plant incinerating forest residues and peat in a fluidized-bed boiler. Fuel Process Technol. 2009;90:871–878.
- Herzel H, Kruger O, Hermann L, et al. Sewage sludge ash—a promising secondary phosphorus source for fertilizer production. Sci Total Environ. 2016;542:1136–1143.
- Franz M. Phosphate fertilizer from sewage sludge ash (SSA). Waste Manag. 2008;28:1809–1818.
- Kruger O, Grabner A, Adam C. Complete survey of German sewage sludge ash. Environ Sci Technol. 2014;48:11811–11818.
- Umeå Energi AB. Om oss – Produktion [Internet] [cited 2019 Aug 14]. Available from: https://www.umeaenergi.se/om-oss/produktion/dava-1-och-2.
- Swedish Standards Institute. Characterization of waste – leaching – compliance test for leaching of granular waste materials and sludges –. 10.01.2003. Stockholm: SIS Förlag AB. (13.030.10; 13.030.20).
- Lo HM, Liu MH, Pai TY, et al. Biostabilization assessment of MSW co-disposed with MSWI fly ash in anaerobic bioreactors. J Hazard Mater. 2009;162:1233–1242.
- McCarty PL. Anaerobic waste treatment fundamentals: part three toxic materials and their control. Public Works. 1964;95(11):91–94.
- Owen WF, Stuckey DC, Healy JB, et al. Bioassay for monitoring biochemical methane potential and anaerobic toxicity. Water Res. 1979;13:485–492.
- Seviour R, Nielsen PH. Microbial ecology of activated sludge. Water Intelligence Online. 2010;9; doi:https://doi.org/10.2166/9781780401645.
- Crocetti G, Murto M, Björnsson L. An update and optimisation of oligonucleotide probes targeting methanogenic Archaea for use in fluorescence in situ hybridisation (FISH). J Microbiol Methods. 2006;65:194–201.
- Kelessidis A, Stasinakis AS. Comparative study of the methods used for treatment and final disposal of sewage sludge in European countries. Waste Manag. 2012;32:1186–1195.
- Decagon Devices. Electrical conductivity of soil as a predictor of plant response.
- Hulshoff Pol LW, Lens PNL, Stams AJM, et al. Anaerobic treatment of sulphate-rich wastewaters. Biodegradation. 1998;9:213–224.
- Wu H, Yang D, Zhou Q, et al. The effect of pH on anaerobic fermentation of primary sludge at room temperature. J Hazard Mater. 2009;172:196–201.
- Schrödter K, Bettermann G, Staffel T, et al. Phosphoric acid and phosphates. In: Ullmann’s encyclopedia of industrial chemistry; Weinheim: Wiley-VCH; 2014; p. 679–724.
- Kratz S, Schnug E. Agronomische Bewertung von Phosphat-Düngern. Braunschweig; 2008. (Ressourcen schonender Einsatz von Phosphor in der Landwirtschaft) [cited 16.11.16].
- Wisotzky F. Angewandte Grundwasserchemie, Hydrogeologie und hydrogeochemische Modellierung. Berlin (Heidelberg): Springer; 2011.
- Kongshaug G, Brentnall BA, Chaney K, et al. Phosphate fertilizers. In: Ullmann’s encyclopedia of industrial chemistry; Weinheim: Wiley-VCH; 2014; p. 1–49.