Abstract
Over the last decade X‐ray absorption near edge structure (XANES) spectroscopy has been used in an increasing number of microbiological studies. In addition to other applications it has served as a valuable tool for the investigation of the sulphur globules deposited intra‐ or extracellularly by certain photo‐ and chemotrophic sulphur‐oxidizing (Sox) bacteria. For XANES measurements, these deposits can easily be concentrated by filtration or sedimentation through centrifugation. However, during oxidative metabolism of reduced sulphur compounds, such as sulphide or thiosulphate, sulphur deposits are not the only intermediates formed. Soluble intermediates such as sulphite may also be produced and released into the medium. In this study, we explored the potential of XANES spectroscopy for the detection and speciation of sulphur compounds in culture supernatants of the phototrophic purple sulphur bacterium Allochromatium vinosum. More specifically, we investigated A. vinosum ΔsoxY, a strain with an in frame deletion of the soxY gene. This gene encodes an essential component of the thiosulphate‐oxidizing Sox enzyme complex. Improved sample preparation techniques developed for the ΔsoxY strain allowed for the first time not only the qualitative but also the quantitative analysis of bacterial culture supernatants by XANES spectroscopy. The results thus obtained verified and supplemented conventional HPLC analysis of soluble sulphur compounds. Sulphite and also oxidized organic sulphur compounds were shown by XANES spectroscopy to be present, some of which were not seen when standard HPLC protocols were used.
Introduction
The phototrophic purple sulphur bacterium Allochromatium vinosum (formerly Chromatium vinosum) oxidizes reduced sulphur compounds, such as sulphide, thiosulphate and elemental sulphur, to sulphate with ‘elemental’ sulphur stored in globules as an obligate intermediate [Citation1]. To analyse the turnover of sulphide, thiosulphate, elemental sulphur and sulphite into sulphate, the concentrations of these sulphur compounds have so far been determined using established high‐performance liquid chromatography (HPLC) techniques [Citation2–Citation5]. However, oxidized organic sulphur compounds (e.g. sulphone, sulphonate) as well as unidentified thiols may also play an important role. We therefore considered it worthwhile to search for more sensitive techniques that allow the speciation analysis of soluble sulphur compounds present in concentrations below the detection limit of HPLC.
X‐ray absorption near edge structure (XANES) spectroscopy is non‐destructive, and a ‘quantitative analysis’ of XANES spectra can be performed (for examples see references [Citation6–Citation12]). So far, XANES spectroscopy in bacteria has mainly been used to analyse sulphur globules produced by a variety of photo‐ and chemotrophic sulphur bacteria [Citation13]. Recently, a study on the nature of the elemental sulphur taken up by A. vinosum has been carried out [Citation14]. Sulphur deposited in sulphur globules and elemental sulphur added to bacterial cultures can easily be separated and concentrated from culture fluids by filtration or sedimentation through centrifugation. Sample preparation for XANES spectroscopy, i.e. application of essentially solid material to Kapton® film, is therefore relatively straightforward. Considering the high sensitivity of the method, analysis of liquid samples by XANES would also be highly desirable; however, it has not yet been undertaken for bacterial culture supernatants. The application of the method for analysis of liquid samples from other biological sources has been reported [Citation15,Citation16] and can serve as a starting point for the development of suitable sample preparation techniques.
Though not of critical importance for the following results and their discussion, it should be mentioned that there is an ongoing discussion as to whether it is possible to prepare samples from ‘pure, solid’ sulphur that are suitable for reliable measurements, i.e. measurements that are not distorted by experimental artefacts of sulphur K‐edge XANES spectra in transmission mode [Citation17]. Spectra taken in this mode have been the basis for the analyses of various sulphur globules regarding the chemical form of sulphur, i.e. the ratio between polymeric and cyclo‐octasulphur [e.g. Citation7,Citation14]. Though the methodological problems described in detail by George et al. [Citation17] are real and have to be considered seriously, there are experimental results published in the literature (not quoted by George et al. in their recent discussion) that strongly support the analyses as carried out by Prange et al. [e.g. Citation7,Citation13] For example, Chauvistré et al. [Citation18] studied the in situ vulcanization of rubber – a sample where grain sizes and homogeneity do not play a role because of the low sulphur concentration – by sulphur K‐edge XANES spectroscopy, and they observed in their spectra the opening of the cyclo‐octaring leading to ‘polymeric’ sulphur: a process well known in rubber chemistry. Durand et al. [Citation19] measured directly the sulphur K‐edge XANES spectra of cyclic and polymeric sulphur using the photoyield mode – a technique not very sensitive to the specific preparation of the sample – and they observed also some distinct differences between the two allotropic forms. Apart from the information here, the reader who is interested in more details on the XANES technique and measurement modes is referred to the excellent textbooks of Koningsberger and Prins [Citation20] and Stoehr [Citation21] or to the articles by George et al. [Citation17] and Prange et al. [Citation13].
In this study, we chose the A. vinosum mutant strain ΔsoxY [Citation5] as a well‐suited model for the analysis of soluble sulphur compounds in bacterial culture fluids. The SoxY protein is an essential part of the thiosulphate‐oxidizing Sox enzyme complex [Citation22]. The A. vinosum ΔsoxY strain lacks soxY because of an in frame deletion of the gene [Citation5]. This leads to an interesting phenotype: the strain is not only unable to oxidize thiosulphate to sulphate [Citation5] but the oxidation of externally added sulphite to sulphate is also severely impaired [Citation23]. In addition, sulphite is excreted during the oxidation of sulphide [Citation24]. In contrast, sulphite does not accumulate during growth of the wild type in batch culture [Citation25]. In addition to sulphite, other inorganic and/or organic sulphones may be formed by the ΔsoxY mutant strain turning it into an ideal candidate for the assessment and improvement of new detection methods.
Materials and methods
Bacterial strains, media and growth conditions
Allochromatium vinosum ΔsoxY was cultivated photolithoautotrophically in batch culture at 30 °C under anaerobic conditions in a thermostatted glass fermenter (culture volume 1.5 L) containing modified Pfennig's medium (0 medium without sulphide) [Citation5]. Sulphide (2 mM) was added to the cultures as the sole sulphur source. For sodium sulphide (Sigma‐Aldrich) 1 M stock solutions was prepared, autoclaved and used for the experiments. To maintain pH 7.0 ± 0.1, sterile HCl and Na2CO3 solutions were added. As an inoculum for the growth experiments, cells of A. vinosum, grown photo‐organoheterotrophically on malate, were used and added to the 0 medium. For growth experiments in which even traces of oxygen were excluded, the inoculum was prepared in an anaerobic chamber (Coy Laboratory Products Inc., Grass Lake, MI) and then transferred into the fermenter under anoxic conditions.
Determination of sulphur compounds by HPLC
Sulphide, thiosulphate, sulphite and sulphate were determined by HPLC (Thermo Separation Products, Egelsbach, Germany) using the method of Rethmeier et al. [Citation3] and Hensen et al. [Citation5].
XANES spectroscopy – experimental
XANES spectra were recorded at the Double Crystal Monochromator (DCM) beamline of the Center for Advanced Microstructures and Devices (CAMD), Baton Rouge, LA [Citation26]. The CAMD's storage ring was operated at an energy of 1.3 GeV with electron currents between 200 and 80 mA. The synchrotron radiation was monochromatized by a modified Lemonnier‐type double crystal monochromator [Citation27] equipped with InSb (111) crystals. The monochromatic flux rate per second on the sample was about 5 × 108 photons (at 200 mA). Measurements (repeated twice) of bacterial cells were performed in transmission mode with ionization chambers (42 torr air pressure inside). Measurements of supernatants (repeated twice) were performed in fluorescence mode using a Vortex™ silicon drift fluorescence detector (SII Nano Technology Inc., Northampton, MA, USA) (sample chamber filled with helium in cases of ‘anaerobic’ samples). For energy calibration, the spectrum of zinc sulphate was used as a ‘secondary standard’, setting the maximum of the first resonance (white line) to an energy of 2481.44 eV (reproducibility ± 0.1 eV). The spectra were scanned with step widths of 0.5 eV between 2440 and 2468 eV, 0.1 eV between 2468 and 2485 eV, and 0.3 eV between 2485 and 2520 eV (integration time 1 s). Using the Origin program (OriginLab, Chiba‐Shi, Chiba, Japan), a linear background determined in the pre‐edge region was subtracted from the raw data to correct the spectra for contributions from higher shells and from supporting materials. Spectra were normalized at 2510 eV.
Quantitative analysis of spectra
The fitting and plotting package WINXAS [Citation28] was used. The program provides tools to find the minimum value of a multi‐parameter function and to analyse the shape of the function around the minimum. Different reference spectra were used for fitting the bacterial spectra (see the section on Reference compounds). A chi square criterion was applied to find the linear combination of these spectra that reproduced the XANES spectrum of interest with the highest probability. Further details concerning the quantitative analysis, especially its verification, potential and restrictions, have been published previously [Citation7,Citation29]. The errors of the percentage contributions of sulphur species (Table ) can be estimated to be <10% (absolute value) (for details on estimation of errors within the microbiological system ‘bacterial sulphur globules’ see [Citation7,Citation29]).
Table 1. Results of fitting the sulphur K‐edge XANES spectra of the culture supernatants to the sum of the reference spectra.
Sample preparation
For XANES measurements of culture supernatants, 2 mL of culture suspension were centrifuged for five minutes at 9000 × g and an aliquot of the supernatants was pipetted onto sulphur‐free filter paper and directly measured. Bacterial cells were harvested and handled as described previously [Citation7,Citation30]. When anoxic conditions were required, sample preparation was performed in an anaerobic chamber (Coy Laboratory Products Inc., Grass Lake, MI, USA). For XANES measurements in the absence of oxygen, the samples were prepared under a nitrogen atmosphere in a glove box using a special sample holder that allowed transfer into the beamline filled with helium under inert gas conditions.
Reference compounds
Glutathione (oxidized and reduced), sodium sulphite, sodium thiosulphate, methionine sulphone, cysteic acid, methionine and zinc sulphate were used as reference compounds for fitting the spectra. These compounds were of reagent grade, purchased from Sigma and used as received. Furthermore, pure cyclo‐octasulphur (S8 rings) and polymeric sulphur were used as reference compounds, both kindly provided by Professor Dr. Ralf Steudel, Technical University Berlin, Germany. The reference compounds were ground into a fine powder and placed homogeneously on a sulphur‐free, self‐adhesive Kapton® film (type 7010, CWC Klebetechnik, Frankenthal, Germany) and/or sulphur‐free filter paper covered with polypropylene (SPEX Industries, Edison, NJ, USA).
Results and discussion
The substrate‐binding protein SoxYZ is an essential component of the Sox enzyme complex of A. vinosum. In strain ΔsoxY, one of the two genes (soxY) encoding this protein is deleted [Citation5]. This leads to complete inhibition of thiosulphate oxidation and a reduction in the sulphite oxidation rate by about 60% as compared with the wild type [Citation5,Citation23,Citation24]. Figure shows a characteristic development of concentrations of sulphide, thiosulphate, sulphite, sulphur and sulphate during photolithoautotrophic growth of A. vinosum ΔsoxY on 2 mM sulphide as determined via established HPLC techniques. Within the first seven hours, sulphide is completely oxidized and transformed into intracellular sulphur globules. Polysulphides are known intermediates of this step [Citation2] but are not shown, for clarity. When sulphide is used up, further oxidation of the stored sulphur starts. Because of strongly decreased sulphite oxidation rate of strain ΔsoxY, sulphite excretion into the medium starts about one hour after the onset of sulphur globule degradation. Sulphite accumulates up to a maximum of 0.6 mM. When intracellular sulphur is almost completely oxidized, sulphite is taken up again and finally oxidized to sulphate. During sulphide depletion, the formation of thiosulphate has been reported for A. vinosum wild type [Citation31] and several green sulphur bacteria (Chlorobiaceae) [Citation32,Citation33]. This also occurs in mutant A. vinosum ΔsoxY. Up to 0.4 mM thiosulphate was detected in the culture supernatants. This thiosulphate could not be further oxidized because of the lack of SoxYZ. The phenotype of A. vinosum ΔsoxY and its implication on the pathway of sulphur oxidation in purple sulphur bacteria will be discussed in detail elsewhere.
Figure 1 Turnover of sulphur compounds by A. vinosum δsoxY during photolithoautotrophic growth on 2 mM sulphide. A representative of triplicate experiments is shown. Protein concentrations were between 80 and 120 mg mL−1.
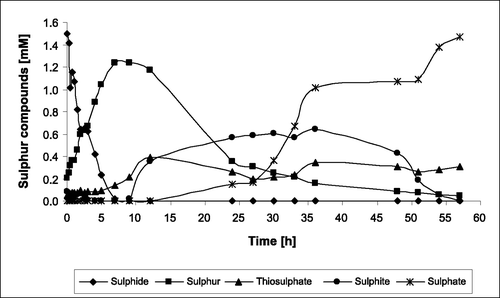
Due to the observed accumulation of a whole range of soluble sulphur compounds of different oxidation states, strain A. vinosum ΔsoxY proved to be an ideal candidate for the investigation of culture supernatants by XANES spectroscopy.
Culture supernatants of the A. vinosum mutant strain ΔsoxY, grown with sulphide as electron donor for anoxygenic photosynthesis, were investigated by XANES spectroscopy. The described HPLC measurements served as internal controls. For XANES spectroscopy, samples of culture fluid were taken at regular time intervals. Supernatants and cells were separated by centrifugation as described in Materials and Methods. The XANES spectra of supernatants were recorded before the excretion of sulphite (3 h after addition of sulphide), during the excretion of sulphite (27 h after addition of sulphide) and after complete oxidation of sulphite to sulphate (58 h after addition of sulphide). Figure shows the spectra obtained for supernatants. In Table the results of fitting the sulphur K‐edge XANES spectra using WINXAS are summarized. Spectra of the reference substances used for fitting the supernatant spectra are presented in Figure . For all culture supernatants, the percentage contributions of sulphite, thiosulphate and sulphate agree with the respective concentrations determined by HPLC. Only traces of sulphite are present in the sample taken 3 h after sulphide addition, whereas, in the sample taken after 27 h, sulphite makes up 56% of all sulphur compounds present. Sulphite gives rise to a typical, pronounced structure at an energy of 2477.3 eV (Figure : spectrum b). After 58 h sulphite is used up: only traces are detectable by XANES and the structure at 2477.3 eV is nearly completely missing in the spectrum (Figure : spectrum c). Significant thiosulphate contributions to the spectra for all culture supernatants reflect the formation of thiosulphate and its impaired degradation. The contribution of sulphate to the spectra increases within 58 h as a result of complete oxidation of intracellularly stored sulphur. After 58 h the sulphate maximum at 2481.4 eV is the dominating spectral feature (Figure : spectrum c). The contribution of sulphate to the spectra obtained after 3 h is caused by micromolar amounts of sulphate in the medium before inoculation, which were added via the trace element solution containing iron sulphate. These amounts of sulphate were below the detection limit of the HPLC method used to analyse the supernatants. Nevertheless, they obviously caused a pronounced local maximum in the XANES spectra thereby demonstrating the higher sensitivity of this method.
Figure 2 Sulphur K‐edge XANES spectra (solid lines) of the supernatants of A. vinosum δsoxY cultures before (a), during (b) and after (c) excretion of sulphite and accompanying fits (dashed lines). Samples where prepared under (A) oxic and (B) anoxic conditions. (a.u. = arbitrary units).
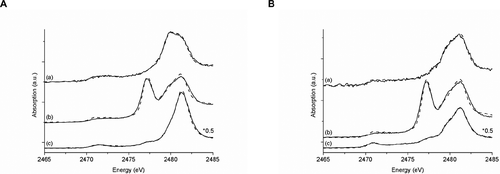
Figure 3 Sulphur K‐edge XANES spectra of the reference compounds which were used for fitting bacterial and supernatant spectra: polymeric sulphur (a), cyclo‐octasulphur (S8 rings) (b), oxidized glutathione (c), reduced glutathione (d), methionine (e), sodium thiosulphate (f), sodium sulphite (g), methionine sulfone (h), cysteic acid (i) and zinc sulphate (j). (a.u. = arbitrary units).
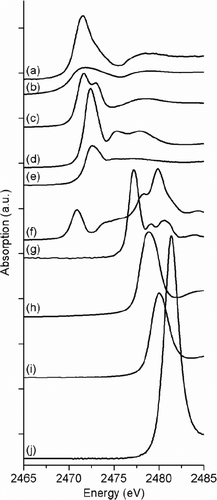
While the percentage contributions of sulphite, thiosulphate and sulphate calculated from the XANES spectra match well to the HPLC results, surprisingly, sulphide is not visible in the XANES spectra, although it is present at a concentration of 0.6 mM in the sample taken after 3 h, according to HPLC analysis. This is probably due to the fact that sodium sulphide is protonated in water forming the volatile hydrogen sulphide, H2S (boiling point = −60.33 °C, pK a = 6.89). Thus, sulphide is probably completely used up as hydrogen sulphide during preparation and transport of the samples. The nitrogen and/or helium atmosphere (approx. 5 min) required during preparation of the samples (see Materials and Methods) may additionally have contributed to this effect. Prange [Citation34] successfully measured XANES spectra of a sulphide solution. However, it should be kept in mind that the ‘feeding solution’ investigated in the course of that study contained much higher concentrations of sulphide. Losses as hydrogen sulphide could be neglected in the latter case, while they led to the observed drastic effect on the comparatively dilute culture supernatants. When HPLC is used to determine sulphide concentrations, only traces of sulphide are lost because the samples are immediately derivatized with the fluorescent thiol labelling reagent monobromobimane, an obvious and indispensable advantage for the identification and determination of sulphide. To rule out that sulphide was lost by transformation into more oxidized forms by exposure to air during sample preparation, supernatants were also prepared and measured under anoxic conditions (nitrogen atmosphere). However, the same sulphur compounds with slightly different percentage contributions were found in the ‘anoxic’ supernatants (Table ). Sulphide was missing in these samples as well, which is usually volatilized (outgassing) as hydrogen sulphide during transport and preparation (can be sniffed!) instead of undergoing chemical oxidation. The observed differences in percentage contributions were probably caused by different handling of the cultures yielding the ‘oxic’ and ‘anoxic’ samples. For example, it was necessary to draw samples from two different sets of cultures of A. vinosum ΔsoxY. While independent cultures do show comparable growth, they are never completely identical. These minor variations in growth lead to the differences in percentage contributions.
For thiosulphate, sulphite and sulphate, the XANES fitting results not only reflect the development of their concentrations but are also in accordance with the molar concentration ratio of these sulphur compounds as determined by HPLC. Due to the presence of some sulphur species that are not detectable by HPLC but cause characteristic absorption features in the XANES spectra (see below), the sulphur balances (sum of the molar concentrations of all soluble sulphur compounds is equivalent to 100%) obtained from HPLC results could not be used for direct calculation of the molar concentrations from the different percentage contributions. For that reason, indirect calculations were applied: for all points in time, the molar concentration of thiosulphate determined by HPLC was used as a fix point and set equivalent to the respective percentage contribution of thiosulphate obtained by fitting the three supernatant spectra with WINXAS. Then, on reverse, the molar concentrations for sulphite and sulphate were deduced from their percentage contributions to the XANES spectra and compared with the values obtained by HPLC. Table shows that all concentration values thus obtained are in good agreement with the measured HPLC values. Variations were between 2 µM for sulphite after 3 h and sulphate after 27 h and 122 µM for sulphate after 58 h. In summary, our findings are an impressive example for the suitability of XANES as a tool for analysing sulphur speciations of culture supernatants of A. vinosum and probably also other sulphur‐oxidizing and sulphur‐reducing bacteria.
Table 2. Molar concentrations of different sulphur compounds in the culture supernatants calculated from their percentage contributions observed by fitting the spectra compared to the concentrations measured by HPLC.
In addition to the compounds that were readily detectable by HPLC, C‐S‐S‐C, C‐S‐H, C‐SO2‐C and C‐SO3 − species significantly contributed to the XANES spectra. Their percentage contributions and accordingly their concentrations in the samples were quite low and with one exception did not exceed 0.18 mM (Tables and ). Only C‐SO3 − was present in higher amounts in the samples taken before and after excretion of sulphite (43% and 21%, respectively). We can exclude the possibility that the oxidized sulphur species detected in the supernatants resulted from oxidation of organic sulphur compounds by atmospheric oxygen rather than from true cellular activity, because the same sulphur compounds with only slightly different percentage contributions were also found in the supernatants prepared under anoxic conditions (Table ). The presence of oxidized organic sulphur compounds indicates that they may play a role in the oxidation of sulphide by A. vinosum ΔsoxY. Whether this generally applies to A. vinosum wild type or is a special feature of the mutant will be investigated in the future. However, the presence of thiols and oxidized organic sulphur compounds shows that XANES spectroscopy of culture supernatants is a valuable complementary method in the analysis of this type of samples.
Table 3. Results of fitting the sulphur K‐edge XANES spectra of A. vinosum ΔsoxY culture pellets to the sum of the reference spectra. Sample preparation was performed under oxic conditions.
In addition to the above described analysis of culture supernatants, the effects of a lack of SoxYZ on the formation and degradation of sulphur globules, as well as on the concentrations of intracellular sulphur compounds, were studied. To this end, cell pellets of the A. vinosum mutant strain ΔsoxY, sampled at the same points in time as the supernatants, were investigated using XANES spectroscopy. These measurements did not yield any new results. In the pellets taken after 3 h and 27 h, polymeric sulphur is the dominating sulphur species (Figure : spectra a and b, Table ). This is in accordance with the results reported by Prange et al. [Citation7] who showed that sulphur globules of A. vinosum consist of polymeric sulphur chains terminated by organic residues. Fifty‐eight hours after sulphide addition, polymeric sulphur is not present any more (Figure : spectra c, Table ) because the sulphur globules are completely degraded. Fitting of the respective XANES spectrum was only possible with a modified set of reference substances. While polymeric sulphur and cyclo‐octasulphur were excluded, methionine, as well as oxidized and reduced glutathione, was included as references for C‐S‐S‐C and C‐S‐H species. This set of reference substances represents the sulphur speciation in sulphur‐globule‐free cells that are dominated by the sulphur‐containing amino acids cysteine and methionine together with C‐S‐S‐C and C‐S‐H species. In addition, cell pellets sampled under anoxic conditions were investigated (spectra and fitting data not completely shown), showing only slight differences. The cell pellet sampled after 58 h under anoxic conditions contained 6% C‐SO3 −, 30% C‐S‐S‐C, 22% C‐S‐H, 37% C‐S‐C and 6% SO4 2− compared with 7%, 25%, 15%, 45% and 6%, respectively, for the pellets prepared in the presence of air. These differences can be attributed to variations that are regularly observed between independent cultures (see above).
Figure 4 Sulphur K‐edge XANES spectra (solid lines) of the cell pellets of A. vinosum δsoxY cultures before (a), during (b) and after (c) excretion of sulphite and accompanying fits (dashed lines). Samples where prepared under oxic conditions. (a.u. = arbitrary units).
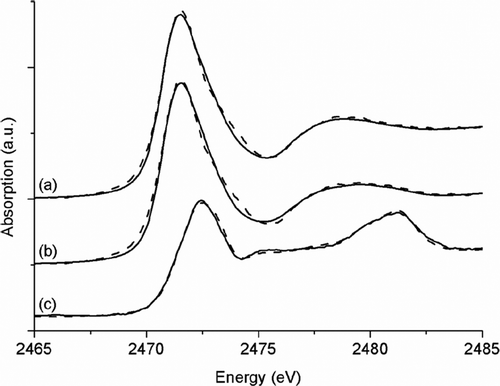
It is noteworthy that neither the pellets prepared under oxic conditions nor those treated under anoxic conditions contained any sulphite, although mutant A. vinosum ΔsoxY is dramatically inhibited in sulphite oxidation. Sulphur globules in A. vinosum are probably reductively activated first and then oxidized to sulphite [Citation35]. The latter process takes place in the cytoplasm and could theoretically lead to increased intracellular sulphite concentrations when sulphite oxidation is impaired as is the case in strain ΔsoxY. However, just as in A. vinosum wild type cells [Citation7,Citation30], sulphite was not detectable by the quite sensitive XANES spectroscopy. It should be kept in mind that spectral features caused by very low sulphite concentrations may be hidden by the dominating structure of polymeric sulphur. Nevertheless, all results obtained thus far indicate that A. vinosum cells generally contain only very low concentrations of the very reactive and toxic sulphite. Instead, sulphite is immediately further oxidized to sulphate in the wild type. When sulphite oxidation is impaired as in strain ΔsoxY it is apparently immediately released into the medium.
Conclusions
When culture supernatants of the A. vinosum mutant strain ΔsoxY were investigated as an example, XANES spectroscopic results reflected the same sulphur compounds as detected by HPLC and in comparable ratios. Furthermore, it was possible to determine from the XANES spectra additional oxidized organic sulphur compounds in the culture supernatants. Their role in oxidation of reduced sulphur compounds in A. vinosum has to be investigated in the future with the aid of this method. In summary, our results show that XANES spectroscopy can serve as an excellent complementary method to HPLC analysis of culture supernatants of photo‐ and chemotrophic sulphur‐oxidizing bacteria. XANES spectroscopy provides additional important qualitative (and semi‐quantitative) information, as well as the possibility of detecting soluble sulphur compounds even in very low concentrations and of investigating samples without the necessity for modification by derivatization.
Acknowledgements
This work was supported by the Deutsche Forschungsgemeinschaft (DFG grants no. PR708/4‐1 and DA351/5‐1). The authors would like to thank the State of Louisiana for providing the operating budget of CAMD. The authors are grateful to CAMD's staff, especially Amitava Roy, Chris W. Bianchetti and Russell ‘Rusty’ L. Louis, for their support during measurements at the DCM beamline.
References
- Dahl , C. 2008 . “ Inorganic sulfur compounds as electron donors in purple sulfur bacteria ” . In Sulfur Metabolism in Phototrophic Organisms , Edited by: Hell , R. , Dahl , C. , Knaff , D.B. and Leustek , T. 289 – 317 . Dordrecht : Springer .
- Prange , A. , Engelhardt , H. , Trüper , H.G. and Dahl , C. 2004 . The role of the sulfur globule proteins of Allochromatium vinosum: Mutagenesis of the sulfur globule protein genes and expression studies by real‐time RT PCR . Arch. Microbiol. , 182 : 165 – 174 .
- Rethmeier , J. , Rabenstein , A. , Langer , M. and Fischer , U. 1997 . Detection of traces of oxidized and reduced sulfur compounds in small samples by combination of different high‐performance liquid chromatography methods . J. Chromatogr. A , 760 : 295 – 302 .
- Sander , J. , Engels‐Schwarzlose , S. and Dahl , C. 2006 . Importance of the DsrMKJOP complex for sulfur oxidation in Allochromatium vinosum and phylogenetic analysis of related complexes in other prokaryotes . Arch. Microbiol. , 186 : 357 – 366 .
- Hensen , D. , Sperling , D. , Trüper , H.G. , Brune , D.C. and Dahl , C. 2006 . Thiosulfate oxidation in the phototrophic sulfur bacterium Allochromatium vinosum . Mol. Microbiol. , 62 : 794 – 810 .
- Modrow , H. , Visel , F. , Zimmer , R. and Hormes , J. 2001 . Monitoring thermal oxidation of sulfur crosslinks in SRB‐elastomers by quantitative analysis of sulfur K‐edge XANES‐spectra . Rubber Chem. Technol. , 74 : 281 – 294 .
- Prange , A. , Chauvistré , R. , Modrow , H. , Hormes , J. , Trüper , H.G. and Dahl , C. 2002 . Quantitative speciation of sulfur in bacterial sulfur globules: X‐ray absorption spectroscopy reveals at least three different speciations of sulfur . Microbiology , 148 : 267 – 276 .
- Prange , A. , Modrow , H. , Hormes , J. , Krämer , J. and Köhler , P. 2005 . Influence of mycotoxin producing fungi (Fusarium, Aspergillus, Penicillium) on gluten proteins during suboptimal storage of wheat after harvest and competetive interactions between field and storage fungi . J. Agric. Food Chem. , 53 : 6930 – 6938 .
- Akabayov , B. , Doonan , C.J. , Pickering , I.J. , George , G.N. and Sagi , I. 2005 . Using softer X‐ray absorption spectroscopy to probe biological systems . J. Synchrotron Radiat. , 12 : 392 – 401 .
- Rompel , A. , Cinco , R.M. , Latimer , M.J. , McDermott , A.E. , Guiles , R.D. , Quintanilha , A. , Krauss , R.M. , Sauer , K. , Yachandra , V.K. and Klein , M.P. 1998 . Sulfur K‐edge X‐ray absorption spectroscopy: A spectroscopic tool to examine the redox state of S‐containing metabolites in vivo . Proc. Nat. Acad. Sci. U.S.A. , 95 : 6122 – 6127 .
- Viamajala , S. , Smith , W.A. , Sani , R.K. , Apel , W.A. , Petersen , J.N. , Neal , A.L. , Roberto , F.F. , Newby , D.T. and Peyton , B.M. 2007 . Isolation and characterization of Cr(VI) reducing Cellulomonas spp. from subsurface soils: Implications for long‐term chromate reduction . Biosresour. Technol. , 98 : 612 – 622 .
- Francis , A.J. , Dodge , C.J. and Gillow , J.B. 2008 . Reductive dissolution of Pu(IV) by Clostridium sp. under anaerobic conditions . Environ. Sci. Technol. , 42 : 2355 – 2360 .
- Prange , A. , Hormes , J. and Modrow , H. 2008 . “ X‐ray absorption spectroscopy as tool for the detection and identification of sulfur compounds in phototrophic organisms ” . In Sulfur Metabolism in Phototrophic Organisms , Edited by: Hell , R. , Dahl , C. , Knaff , D.B. and Leustek , T. 461 – 482 . Dordrecht : Springer .
- Franz , B. , Lichtenberg , H. , Hormes , J. , Modrow , H. , Dahl , C. and Prange , A. 2007 . Utilization of solid ‘elemental’ sulfur by the phototrophic purple sulfur bacterium Allochromatium vinosum: A sulfur K‐edge XANES spectroscopy study . Microbiology , 153 : 1268 – 1274 .
- Cinque , G. , Bellisola , G. , Colombatti , M. and Burattini , E. 2006 . X‐ray absorption near edge spectroscopy of sulfur in biomolecules: Two examples from glutathione and insulin . Acta Phys. Polon. A , 109 : 335 – 340 .
- Solomon , D. , Lehmann , J. and Martínez , C.E. 2003 . Sulfur K‐edge XANES spectroscopy as a tool for understanding sulfur dynamics in soil organic matter . Soil Sci. Soc. Am. J. , 67 : 1721 – 1731 .
- George , G.N. , Gnida , M. , Bazylinski , D.A. , Prince , R.C. and Pickering , I.J. 2008 . X‐ray absorption spectroscopy as a probe of microbial sulfur biochemistry: The nature of bacterial sulfur globules revisited . J. Bacteriol. , 190 : 6376 – 6383 .
- Chauvistré , R. , Hormes , J. , Brück , D. , Sommer , K. and Engels , H.‐W. 1992 . Structural analysis of sulfur in rubber by X‐ray near edge absorption spectroscopy . Kautsch. Gummi Kunstst. , 45 : 808 – 813 .
- Durand , J.M. , Olivier‐Foucade , J. , Jumas , J.C. , Womes , M. , Teodorescu , C.M. , Elafif , A. , Esteva , J.M. and Karnatak , R.C. 1996 . K edge absorption spectra of sulphur in vapour, molecular and polymerized solid phases . J. Phys. B , 45 : 5773 – 5784 .
- Koningsberger , D.C. and Prins , R. , eds. 1988 . “ X‐ray Absorption: Principles, Applications, Techniques of EXAFS, SEXAFS and XANES ” . New York : John Wiley .
- Stoehr , J. 1996 . “ NEXAFS Spectroscopy ” . Berlin : Springer .
- Friedrich , C.G. , Bardischewsky , F. , Rother , D. , Quentmeier , A. and Fischer , J. 2005 . Prokaryotic sulfur oxidation . Curr. Opin. Microbiol. , 8 : 253 – 259 .
- Franz , B. 2005 . “ Untersuchungen zur physiologischen Bedeutung der sox‐Gene in Allochromatium vinosum. Diploma of Science thesis ” . University of Bonn . (In German)
- Hensen , D. 2006 . “ Biochemical and genetic analysis of the Sox multienzyme complex in the purple sulfur bacterium Allochromatium vinosum ” . University of Bonn . Ph.D. diss.
- Dahl , C. 1996 . Insertional gene inactivation in a phototrophic sulphur bacterium: APS‐reductase‐deficient mutants of Chromatium vinosum . Microbiology , 142 : 3363 – 3372 .
- Hormes , J. , Scott , J.D. and Suller , V. 2006 . Facility update: The Center for Advanced Microstructures and Devices: A status report . Synchroton Radiat. News , 19 : 27 – 30 .
- Lemonnier , M. , Collet , O. , Depautex , C. , Esteva , J.‐M. and Raoux , D. 1978 . High vacuum two crystal soft X‐ray monochromator . Nucl. Instrum. Methods , 152 : 109 – 111 .
- Ressler , T. 1998 . WinXAS: A program for X‐ray absorption spectroscopy data analysis under MS‐Windows . J. Synchrotron Radiat. , 5 : 118 – 122 .
- Prange , A. , Birzele , B. , Krämer , J. , Chauvistré , R. , Modrow , H. , Hormes , J. and Köhler , P. 2003 . Characterization of sulfur speciation in low molecular weight subunits of glutenin after reoxidation with potassium iodate and potassium bromate at different pH values using X‐ray absorption near edge structure (XANES) spectroscopy . J. Agric. Food Chem. , 51 : 7431 – 7438 .
- Prange , A. , Arzberger , I. , Engemann , C. , Modrow , H. , Schumann , O. , Trüper , H.G. , Steudel , R. , Dahl , C. and Hormes , J. 1999 . In situ analysis of sulfur in the sulfur globules of phototrophic sulfur bacteria by X‐ray absorption near edge spectroscopy . Biochim. Biophys. Acta , 1428 : 446 – 454 .
- Steudel , R. , Holdt , G. , Visscher , P.T. and van Gemerden , H. 1990 . Search for polythionates in cultures of Chromatium vinosum after sulfide incubation . Arch. Microbiol. , 155 : 432 – 437 .
- Trüper , H.G. and Fischer , U. 1982 . Anaerobic oxidation of sulphur compounds as electron donors for bacterial photosynthesis . Phil. Trans. R. Soc. Lond. B , 298 : 529 – 542 .
- Trüper , H.G. 1984 . “ Phototrophic bacteria and their sulfur metabolism ” . In Sulfur: Its Significance for Chemistry, for the Geo‐, Bio‐, and Cosmosphere and Technology , Edited by: Müller , A. and Krebs , B. 367 – 382 . Amsterdam : Elsevier Science .
- Prange , A. 2008 . “ Speciation analysis of microbiologically produced sulfur by X‐ray absorption near edge structure spectroscopy ” . In Microbial Sulfur Metabolism , Edited by: Dahl , C. and Friedrich , C.G. 259 – 272 . Berlin : Springer .
- Grimm , F. , Franz , B. and Dahl , C. 2008 . “ Thiosulfate and sulfur oxidation in purple sulfur bacteria ” . In Microbial Sulfur Metabolism , Edited by: Dahl , C. and Friedrich , C.G. 101 – 116 . Berlin : Springer .