ABSTRACT
The concepts of comfort and health may be related but are not synonyms. New knowledge has been gathered regarding metabolic health effects of temperature exposure outside the human thermal comfort zone. Mild cold and warm environments increase metabolism, thereby targeting obesity by counterbalancing excess energy intake. Furthermore, mild cold influences glucose metabolism. Ten days of intermittent mild cold exposure in type 2 diabetes patients increased insulin sensitivity, and thereby glucose handling by more than 40%. This is comparable with the best available pharmaceutical or physical activity therapies. Lastly, there are indications that cardiovascular parameters may be positively affected by regular exposure to heat and cold. Does this mean that we have to suffer from discomfort in order to become healthy? Probably not. Firstly, prolonged temporal excursions outside the thermal comfort zone result in acclimatization resulting in increased comfort ratings. Secondly, low or high temperatures in a dynamic thermal environment may be perceived as acceptable or even pleasant (evoking thermal alliesthesia). The study of dynamic thermal conditions is advocated: linking this to the adaptive comfort model, and monitoring these conditions in actual living conditions. This information is needed to support the design of healthy, comfortable and energy-friendly indoor environments.
Introduction: environmental temperature and health
The effects of environmental temperature on human health are difficult to study, since changes in health status develop slowly and may also have indirect causality with temperature. Moreover, other environmental parameters, such as light conditions (Te Kulve, Schellen, Schlangen, & van Marken Lichtenbelt, Citation2016), may have a confounding effect, which makes it even more difficult to analyse the isolated impact of temperature. Nevertheless, prevalent diseases linked to the metabolic syndrome seem to be related to a constantly comfortable environmental temperature (Keith et al., Citation2006; McAllister et al., Citation2009). The metabolic syndrome is one of the most widespread adverse health conditions worldwide and is characterized by obesity, accompanied by a high risk of developing type 2 diabetes, cardiovascular diseases and even certain forms of cancer (Singh et al., Citation2013). Though wider health implications of excursions outside the comfort zone may be evident, this article mainly addresses the metabolic syndrome.
The indoor climate is an important environmental factor to address in order to create a healthy living environment. People spend most of their time indoors in dwellings and offices and nowadays most buildings in Organisation for Economic Co-operation and Development (OECD) countries are equipped with heating and air-conditioning to ensure optimal thermal comfort for the occupants. It has, however, been hypothesized that prolonged exposure to such ‘thermoneutral’ conditions can contribute to the ‘weighty’ tipping of the energy balance (Hansen, Gilman, & Odland, Citation2010; van Marken Lichtenbelt & Kingma, Citation2013; van Marken Lichtenbelt, Kingma, van der Lans, & Schellen, Citation2014). Positive energy balance is the most important causal factor for overweight and obesity.
The main emphasis of most lifestyle programmes tackling overweight and obesity is on weight reduction or weight maintenance after weight loss. Clearly, food intake determines energy intake and a reduction of food intake results in a negative energy balance and thereby results in weight loss. Exercise increases energy expenditure and thereby helps to level out the energy balance or even to promote a negative energy balance. In order actually to lose weight, a reduction of the energy intake may be easier to accomplish than an increase of physical activity. On the other hand, it is well known that increasing physical activity can bring along other health benefits, e.g. it reduces the risk for cardiovascular diseases and it improves insulin sensitivity (Meex et al., Citation2010). Furthermore the efficacy on physiological remodelling may depend on the intensity and duration of the exercise training (MacInnis & Gibala, Citation2016). Nowadays, lifestyle programmes often address both healthy nutrition and physical activity levels. A serious issue is, however, that long-term adherence to these programmes is often not met, though options are available and warrant further investigation. In general, people tend to decrease or even stop the newly acquired behaviours linked to the healthy lifestyle, or compensate for the beneficial effects. In order to induce a healthy lifestyle that is also sustained for the long-term, we hypothesize that the environment (both physical and social) needs to be taken into consideration.
The environment plays a role in human energy balance because when the human body remains in thermoneutral conditions, heat production is minimal to maintain body temperature (Kingma, Frijns, & van Marken Lichtenbelt, Citation2012). By contrast, it has been shown that mild cold can increase human energy metabolism without shivering (Davis, Citation1961; van Ooijen, van Marken Lichtenbelt, van Steenhoven, & Westerterp, Citation2004; Warwick & Busby, Citation1990) and both cold and warmth influence other factors related to metabolic health (Hanssen et al., Citation2015; Pallubinsky et al., Citation2017).
The following sections elaborate on these findings regarding the relations between environmental temperature and the metabolic health and then speculate on the importance of the indoor climate for creating a healthy environment.
Obesity: environmental temperature related to energy and weight balance
The authors hypothesize that environmental temperature relates to the body (energy) metabolism and thereby may affect our body weight and health status. Ideas were formulated earlier (Johnson, Mavrogianni, Ucci, Vidal-Puig, & Wardle, Citation2011; van Marken Lichtenbelt & Kingma, Citation2013; van Marken Lichtenbelt et al., Citation2014), but in the meantime, new data has been collected and insights have been expanded.
Classical thermoregulation models for mammals show that both cold and warm environments can increase our energy expenditure (Scholander, Hock, Walters, & Irving, Citation1950). The so-called Scholander model uses the concept of the thermoneutral zone () (Kingma et al., Citation2012). The thermoneutral zone coincides with minimal metabolic rate being required to sustain body temperature.
Figure 1. The physiological thermoneutral zone is the ambient temperature range where the metabolic rate is at the basal level and there are no regulatory changes in metabolic rate (non-shivering thermogenesis, NST; shivering thermogenesis; or heat-related thermogenesis, HT) or perspiration. In the thermoneutral zone, body core temperature is regulated by skin blood perfusion. The depicted range of the thermoneutral zone is for an average nude person. With clothing, the zone shifts to the left.
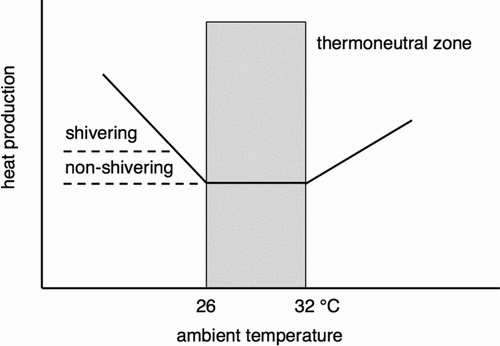
The range and position of the thermoneutral zone on the temperature axis depend on relative humidity, air speed and the level of clothing, but also on the morphology of the body, body composition and the amount of physical activity which affect the metabolic rate (heat production) and heat loss of the body (Kingma et al., Citation2012; Kingma, Schweiger, Wagner, & van Marken Lichtenbelt, Citation2017). Empirical studies show that the human thermoneutral zone in a nude situation ranges from 26 to 32°C (Erikson, Krog, Andersen, & Scholander, Citation1956; Hardy & Du Bois, Citation1938). Furthermore, Erikson et al. (Citation1956) show that increasing resting activity to light cycling and wearing winter sports clothing decreases the lower bound to about 14°C. In practice this means that thermoneutral temperatures depend strongly on clothing and activity levels as well as on the thermal environment and physiology.
In the past, thermoregulation research mainly focused on extreme temperatures, which may not be relevant to our daily circumstances. With the exception of some older studies (Dauncey, Citation1981; Warwick & Busby, Citation1990), investigations on mild temperature variations, as encountered in daily practice, originate from the last 15 years (Celi et al., Citation2010; van der Lans et al., Citation2013; van Marken Lichtenbelt, Schrauwen, Kerckhove, & Westerterp-Plantenga, Citation2002; van Marken Lichtenbelt, Westerterp-Plantenga, & van Hoydonk, Citation2001; Yoneshiro et al., Citation2013). Mild cold (i.e. cold conditions that do not evoke shivering) leads to non-shivering thermogenesis (NST).
The amount of NST is highly variable between individuals (van Ooijen et al., Citation2004), which can be attributed to, among other factors, the amount of acclimatization (Davis, Citation1961) and age (Kingma, Frijns, Saris, van Steenhoven, & van Marken Lichtenbelt, Citation2011). In addition, the temperature that elicits NST is highly variable depending on body size and body composition, as described above. The research team in Maastricht developed a personal cooling protocol by which subjects are cooled to maximize NST (for details, see van der Lans et al., Citation2014). In such circumstances, NST can account for up to 30% of resting metabolism in healthy young adults (van Ooijen et al., Citation2004) and in extreme cases even up to 40% (Vosselman, Vijgen, Kingma, Brans, & van Marken Lichtenbelt, Citation2014). The typical air temperatures for maximal NST are around 14–16°C for lightly clothed, lean adults. However, another study indicates that exposure to 19°C, experienced as a mild and tolerably cold temperature, already results in a predictable increase in energy expenditure (Celi et al., Citation2010). In addition, a cold acclimation study also revealed NST at 19°C, and regular exposure for two hours per day for six weeks to 17°C increased NST and even resulted in significant body fat mass loss (Yoneshiro et al., Citation2013).
NST has been studied for many years in hibernators and small mammals. The tissue responsible for the heat production in these mammals, and also in human babies, is brown adipose tissue (BAT) (Cannon & Nedergaard, Citation2004). Brown fat, when activated by cold, produces more heat than any other tissue in the body. In 2009, the Maastricht research team (the author’s research group) were among the first to evidence the existence of functionally active BAT in adult humans (van Marken Lichtenbelt et al., Citation2009; Saito et al., Citation2009; Virtanen et al., Citation2009). BAT is different from white fat: white fat stores energy whereas brown fat burns fatty acids and glucose when extra body heat is needed during cold exposure. Many rodent studies show that brown fat is the sole tissue responsible for NST, and a reduction or absence of BAT is linked to diet-induced obesity and type 2 diabetes. Both NST and BAT activity are lower in obese persons and in the elderly (van Marken Lichtenbelt et al., Citation2009; Yoneshiro et al., Citation2011). Interestingly, regular exposure to mild cold leads to an increased capacity for NST and brown fat activity in lean healthy young adults () (van der Lans et al., Citation2013; Yoneshiro et al., Citation2013), and also in obese subjects and elderly (Hanssen et al., Citation2016). Importantly, cold acclimation leads to a decrease of thermal discomfort in cold conditions (Hanssen et al., Citation2016). This means that accompanied with a change in the physiological response to cold, a psychological change also occurs. Gradually the cooler situation leads to comfort and a higher acceptance.
Figure 2. Non-shivering thermogenesis (NST) and brown fat activity (arrows) before (PRE) and after (POST) cold acclimation. Source: Figure from van der Lans et al. (Citation2013).
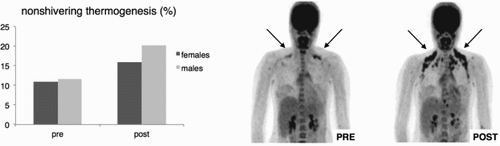
High temperatures can also affect energy expenditure, although the reason for that cannot be attributed to one bodily function or tissue only (like BAT for NST). Comparable with the lack of mild cold research, hardly any studies have been carried out on the effect of mild heat (warmth) exposure and acclimation on thermophysiological parameters and health. Most studies performed so far combined more extreme heat and exercise to evaluate important implications for miners, athletes and the military (Taylor, Citation2014). These so-called active heat-acclimation studies reveal important physiological changes such as a decrease of core temperature, more effective evaporative heat loss and other physiological adaptations, which together result in an improved resilience to heat (for details, see Taylor, Citation2014). However, the results of those very intense heat-acclimation studies are difficult to translate into less extreme temperature challenges as encountered in everyday life. In an attempt to close the knowledge gap, the present authors performed mild passive-warmth-acclimation tests (without exercise) and showed that thermophysiological and behavioural parameters such as body temperatures and temperature perception can be affected by prolonged passive exposure to warmth (Pallubinsky et al., Citation2017). The results indicate that higher indoor temperatures are more easily accepted after passive mild-warmth acclimation. Typical temperatures for mild heat acclimation still need to be determined, but our studies show that significant physiological changes already occur by passive heat acclimation to 33°C, wearing shorts and a T-shirt (Pallubinsky et al., in press). Since the effect is a function of duration and magnitude of exposure, it would be worthwhile to check whether slightly lower temperatures with longer duration (office hours) may yield a similar effect.
Diabetes: environmental temperature related to glucose metabolism
The effect of environmental temperatures on human energy metabolism is relatively straightforward, as depicted by the Scholander model. The mechanisms behind the potential effects of environmental temperature on diabetes type 2 and insulin sensitivity are more complicated. The hormone insulin is one of the main regulators of blood glucose levels. Insulin sensitivity describes the body’s sensitivity to this hormone (Bilous & Donnelly, Citation2010). High insulin sensitivity means that relatively small amounts of insulin are needed to metabolize glucose. Low insulin sensitivity or insulin resistance can ultimately lead to type 2 diabetes, which can subsequently cause other severe health problems. As shown, there are highly significant effects of cold acclimation on energy metabolism by mild cold and because the substrates for energy metabolism are mainly fatty acids (fats) and glucose, it is meaningful to address the effect of cold on insulin sensitivity in patients with type 2 diabetes. Hints also come from animal studies, showing that increased BAT activity prevents both obesity and type 2 diabetes (Bartelt et al., Citation2011; Stanford et al., Citation2013). Some studies in humans also reveal an effect of low environmental temperature on insulin sensitivity (Lee et al., Citation2014).
Following up on this, a cold acclimation experiment was recently performed on patients with type 2 diabetes. One of the primary outcome variables was insulin sensitivity. The acclimation protocol of 10 days was used, with intermittent cold exposure of six hours per day at 15°C. The results derived from the first experiment clearly show that NST was increased, which is in line with former studies in healthy volunteers (see above). The most striking result, however, was that cold acclimation significantly, and to a high extent, affected insulin sensitivity (Hanssen et al., Citation2015). On average, insulin sensitivity increased by 43%. This is comparable with the best treatments strategies of which the authors are aware, such as intense long-term exercise programmes. Cold acclimation did indeed increase brown fat activity, but not to the extent expected. Interestingly, it was found that cold acclimation affected metabolic pathways in skeletal muscle (). Muscle is known to be the main tissue involved in the uptake of glucose, partly because the total amount of skeletal muscle relative to body mass is large. Therefore, small changes in skeletal muscle metabolic pathways may significantly affect whole-body glucose metabolism.
Figure 3. Insulin sensitivity and skeletal muscle glucose transporter type 4 (GLUT4) localization before and after cold acclimation: (A) group mean ± SEM for glucose infusion rate during a hyperinsulinaemic euglycaemic clamp, which is the measurement for insulin sensitivity; (B) GLUT4 translocation in skeletal muscle before and after acclimation in type 2 diabetes patients, as made visible in: (C) representative images of GLUT4 immunostaining of skeletal muscle tissue sections from three individuals in the study. GLUT4 is the insulin-regulated glucose transporter in skeletal muscle fibres. The pictures show that there is more GLUT 4 on the membranes of the cells after cold acclimation. Source: Figure from Hanssen et al. (Citation2015).
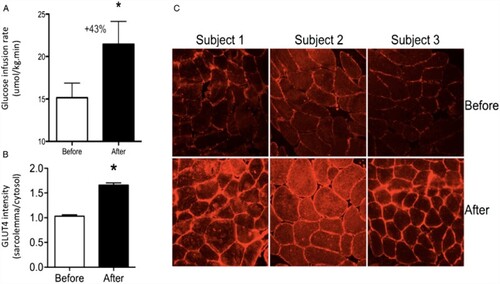
Parallel to the study in type 2 diabetic patients, we also studied cold acclimation in healthy obese subjects. This showed that cold acclimation in this population also led to a cold-induced increase in brown fat activity, and also affected the same skeletal muscle metabolic pathways (Hanssen et al., Citation2016).
Remarkably, in addition to the effect of cold on insulin sensitivity, heat also seems to be linked to glucose metabolism. Results from Hooper (Citation1999) suggest that after three weeks of hot-water immersion for 30 min per day, six days a week, mean fasting plasma glucose levels and other measures related to insulin sensitivity of type 2 diabetes patients improved significantly (Hooper, Citation1999). Other studies also show that heat stress may improve insulin sensitivity (Chung et al., Citation2008). In order to explore the application of these results in a daily living situation, we are currently exploring the effect of passive mild-heat acclimation on insulin sensitivity.
Cardiovascular and other diseases
Both obesity and diabetes are known to increase the risk of developing cardiovascular diseases. Therefore, thermal conditions may indirectly affect this risk, but it may also work more directly. Though cold exposure can result in significant increase in blood pressure and cardiovascular strain (even within the thermoneutral zone), several studies show the positive effects of repeated (severe) cold acclimation during acute cold exposure on skin temperatures, blood pressure and activation of the heart by the autonomic nervous system (e.g. Jansky et al., Citation2006; Makinen et al., Citation2008). Active heat acclimation results in increased blood volume which may increase the cardiac stroke volume (Nielsen et al., Citation1993), lower the heart rate at a given work rate (e.g. Weller, Linnane, Jonkman, & Daanen, Citation2007), change the skin perfusion and increase maximal cardiac output (Lorenzo & Minson, Citation2010). Combined effects of active heat acclimation help to maintain perfusion pressure and improve cardiovascular stability (Corbett, Neal, Lunt, & Tipton, Citation2014).
Changes in environmental temperature, with respect to both heat and cold, affect the heat strain on the body and a significant redistribution of the blood pool is accomplished, which also affects cardiac output and cardiac and vascular strain. Our hypothesis is that through variation in environmental temperatures, the cardiovascular system is exercised, which may affect the resistance to heat and cold. Potentially also the human immune system and resistance to flu or pneumonia may be positively affected. Indeed, several studies have shown that short-term cold exposure affects the immune system in humans, an effect that may be mediated via sympathetic activation as well as by factors released by skeletal muscle (Brenner et al., Citation1999; Kox et al., Citation2014). However, the effect of cold (or warmth) acclimation needs to be investigated in the near future. A first indication of the effect of mild cold acclimation on the immune system derives from our study inducing 10 days of intermittent cold exposure in healthy lean subjects: Here considerable changes of immune cell marker expression in skeletal muscle were observed (van der Lans et al., Citation2015). The physiological consequences and therapeutic relevance of these changes remain to be explored.
In conclusion, (mild) temperature excursions outside the thermal neutral zone affect people’s energy metabolism, but also our glucose metabolism and reduce the risks of cardiovascular diseases. The authors’ current hypothesis on this matter is that temporally exposure to (mild) heat and cold improves our metabolic health (Schrauwen & van Marken Lichtenbelt, Citation2016).
Environmental temperatures, health and the built environment
The above made clear that, apart from the well-known healthy lifestyle factors (diet and physical exercise), the thermal environment can affect human health at least with respect to the metabolic syndrome. Certainly, more studies are needed to confirm the results in different populations and to determine better time and intensity effects, as well as to study additional health-related parameters. For instance, the elderly may be relatively vulnerable to changes in ambient temperature. Cold exposure in the elderly results in a greater increase in blood pressure than in young adults (Kingma et al., Citation2011). On the other hand, regular exposure to mild changes in temperature may increase resilience to more extreme temperatures.
With respect to the duration of the cold or heat exposure, additional studies are needed. As indicated above, the dedicated cold acclimation studies varied from 10 days to six weeks. A systematic approach comparing different durations and intensities of the temperature interventions is warranted. Finally, there is a need to address long-term effects on health. Nevertheless, in recent years the field is rapidly progressing and several important health-related parameters have been identified that are now known to be affected by temperature and can be studied in greater depth, e.g. energy expenditure, brown fat activity, skeletal muscle metabolism, insulin sensitivity, blood pressure, cardiac output and the immune system. Apart from these parameters, future studies on thermal behaviour and long-term compliance need attention.
Despite this, the authors suggest that enough physiological information on the effects of indoor temperature on health has been gathered to start putting this knowledge into practice. Therefore, the question arises: how can this knowledge be translated into the built environment? Here, several aspects need to be taken into account. First, the proposed interventions must be acceptable and feasible. Second, the anticipated level of compliance with the intervention should be considered. Lifestyle intervention studies identified many reasons for the lack of compliance that may in part also affect temperature interventions: habits, discomfort, social conformity and lack of appropriate information.
With respect to thermal (dis)comfort, it was shown (as mentioned above) that during the experiments involving 10 days of cold acclimation, thermal comfort changes from uncomfortable to just comfortable. This change in comfort was significant and may even increase with longer duration of acclimation. Those experiments used quite low (15°C) temperatures, but as stated above others (Yoneshiro et al., Citation2013) have used 17–19°C with significant effects on energy metabolism approaching realistic indoor temperature conditions. Our warmth acclimation experiments did not reveal significant changes in comfort ratings, but the ratings in general were mostly only ‘slightly uncomfortable’ (see Pallubinsky et al., Citation2017, this issue). Studies on more severe active heat acclimation on thermal comfort are not available, but it is reported that the perceived exertion and thermal sensation are improved after heat acclimation (Pandolf et al., Citation1988).
The available data on comfort changes upon heat/cold acclimation are in line with the adaptive comfort model that shows that variation in daily and seasonal temperatures can be experienced without discomfort (de Dear & Brager, Citation1998; Nicol & Humphreys, Citation1973) and ASHRAE’s adaptive comfort model ASHRAE Standard 55 (ASHRAE, Citation2010) as long as people are able to adapt to their climate (season) through a combination of behavioural, physiological and psychological mechanisms (de Dear & Brager, Citation1998) and have some (actual or perceived) personal control. Moreover, it has been shown that independent of season, both young adults and elderly accept a day time variation of 8 K (e.g. 17–25°C) in a dynamic situation (Schellen, van Marken Lichtenbelt, Loomans, Toftum, & de Wit, Citation2010). Though the elderly reported slightly lower comfort than young adults, both groups reported that the temperatures were acceptable. It can be speculated that a cold environment feels acceptable when in a dynamic situation one feels the temperature is rising, and vice versa. In addition, it is well known that dependent on the thermal history of the subjects, low or high temperatures in a dynamic thermal environment may even be perceived as pleasant and support alliesthesia (Cabanac, Citation1971; de Dear, Citation2011; Parkinson & de Dear, Citation2015). It is important to realize that the temperatures of the experiment of Schellen et al. (Citation2010), in addition to the temperatures as described in the adaptive comfort model as being acceptable, include those of which are now known to increase the body’s energy metabolism, and may increase glucose metabolism and the cardiovascular system. Another benefit of a dynamic indoor climate is the potential for energy savings, which could be achieved by implementing a dynamic temperature profile rather than a steady-state temperature in buildings (Kolarik, Toftum, Olesen, & Jensen, Citation2011).
Change of indoor temperature
Currently, the recommended design indoor temperatures are based on standards such as ASHRAE 55 (Citation2010) and EN-ISO 7730 (Citation2005). Application of these guidelines in practice results in buildings that are designed for a homogenous population with comparable preferences. This gradual thermal homogenization across the years has been attributed to the use of central heating (e.g. Unander, Ettestol, Ting, & Schipper, Citation2004) and air-conditioning (e.g. Biddle, Citation2008). In addition, it has been postulated that during the last 40 years, the generally used thermal comfort criteria based on the PMV model (Fanger, Citation1970) resulted in these uniform indoor air temperatures, with minimal variations according to time of day or season. Gradually, temperatures become more and more homogenous and there is an upwards trend in winter indoor temperatures, especially so in bedrooms (Mavrogianni et al., Citation2011), and a downward trend in summer and in the tropics (e.g. Bae & Chun, Citation2009). The latter study in Seoul, Korea, also showed that the comfort temperature increased in the heating period and decreased in the cooling period during the last 25 years. This suggests that in parallel with the changes in the last half-century tending towards a homogeneous temperature in all seasons; also our comfort ranges narrowed. There is some circumstantial evidence from a Dutch newspaper search from 1872 onwards (using the keyword ‘room-temperature’) that c.1870 a temperature of 13–15°C was experienced as being comfortable (Knip, Citation2016). This links to the conclusion of Mavrogianni et al. (Citation2011) that in the UK an increase in winter in mean dwelling indoor temperatures of 1.3°C per decade has occurred between 1978 and 1996.
The trend towards higher temperatures in wintertime and lower in summer in combination with the above-described health benefits of temperatures outside the thermal comfort zone therefore can have significant effects on our metabolic health. It also shows that potentially this trend can be reversed in order to promote metabolic health.
Practical implications
Dynamic (drifting) and locally varying temperatures can quite easily be implemented in practice. In fact, there already exist modern buildings that use dynamic temperature drifts and different local indoor climate zones. Moreover, we echo that the use of personal comfort systems (Brager, Zhang, & Arens, Citation2015; Zhang, Arens, & Zhai, Citation2015) to expand the indoor temperature band in offices needs consideration not only in order to achieve comfort but also to create healthy conditions. In theory the health implications of the thermal environment are general applicable, but they need to be attuned to the type of building (dwellings, offices, care centres, schools etc.), its users (age, gender), the anticipated (physical) activities and the adaptive opportunities offered by the buildings. In future, there is a need to undertake monitoring studies under living laboratory and actual daily living conditions, preferably comparing different thermal strategy interventions. This can be accomplished by using living laboratory environments and by studying effects in neighbourhoods. The latter can ideally be used for research involving long-term effects on health and wellbeing in combination with other lifestyle interventions.
Disclosure statement
No potential conflict of interest was reported by the authors.
Additional information
Funding
References
- ASHRAE. (2010). Standard 55: Thermal Environmental Conditions for Human Occupancy. Atlanta: American Society of Heating, Refrigerating and Air-Conditioning Engineers.
- Bae, C., & Chun, C. (2009). Research on seasonal indoor thermal environment and residents’ control behavior of cooling and heating systems in Korea. Building and Environment, 44(11), 2300–2307. doi:10.1016/j.buildenv.2009.04.003
- Bartelt, A., Bruns, O. T., Reimer, R., Hohenberg, H., Ittrich, H., Peldschus, K., … Heeren, J. (2011). Brown adipose tissue activity controls triglyceride clearance. Nature Medicine, 17(2), 200–205. doi:10.1038/nm.2297
- Biddle, J. (2008). Explaining the spread of residential air conditioning, 1955–1980. Explorations in Economic History, 45(4), 402–423. doi:10.1016/j.eeh.2008.02.004
- Bilous, R. & Donnelly, R. (2010). Handbook of diabetes. Oxford: Wiley-Blackwell.
- Brager, G., Zhang, H., & Arens, E. (2015). Evolving opportunities for providing thermal comfort. Building Research & Information, 43(3), 274–287. doi:10.1080/09613218.2015.993536
- Brenner, I. K., Castellani, J. W., Gabaree, C., Young, A. J., Zamecnik, J., Shephard, R. J., & Shek, P. N. (1999). Immune changes in humans during cold exposure: effects of prior heating and exercise. Journal of Applied Physiology, 87(2), 699–710.
- Cabanac, M. (1971). Physiological role of pleasure. Science, 173(4002), 1103–1107. doi: 10.1126/science.173.4002.1103
- Cannon, B., & Nedergaard, J. (2004). Brown adipose tissue: function and physiological significance. Physiological Reviews, 84(1), 277–359. doi: 10.1152/physrev.00015.2003
- Celi, F., Butler, P. W., Brychta, R. J., Linderman, J. D., Alberobello, A. T., Smith, S., … Chen, K. Y. (2010). Minimal changes in environmental temperature result in a significant increase in energy expenditure and changes in the hormonal homeostasis in healthy adults. European Journal of Endocrinology, 163(6), 863–872. doi: 10.1530/EJE-10-0627
- Chung, J., Nguyen, A. K., Henstridge, D. C., Holmes, A. G., Chan, M. H., Mesa, J. L., … Febbraio, M. A. (2008). HSP72 protects against obesity-induced insulin resistance. Proceedings of the National Academy of Sciences, 105(5), 1739–1744. doi:10.1073/pnas.0705799105
- Corbett, J., Neal, R. A., Lunt, H. C., & Tipton, M. J. (2014). Adaptation to heat and exercise performance under cooler conditions: a new hot topic. Sports Medicine, 44(10), 1323–1331. doi:10.1007/s40279-014-0212-8
- Dauncey, M. J. (1981). Influence of mild cold on 24 h energy expenditure, resting metabolism and diet-induced thermogenesis. British Journal of Nutrition, 45(2), 257–267. doi: 10.1079/BJN19810102
- Davis, T. R. A. (1961). Chamber cold acclimatization in man. Journal of Applied Physiology, 16, 1011–1015.
- de Dear, R. (2011). Revisiting an old hypothesis of human thermal perception: alliesthesia. Building Research & Information, 39(2), 108–117. doi: 10.1080/09613218.2011.552269
- de Dear, R., & Brager, G. S. (1998). Developing an adaptive model of thermal comfort and preference. ASHRAE Transactions, 104(1), 145–167.
- EN-ISO. (2005). ISO 7730: Ergonomics of the thermal environment – Analytical determination and interpretation of thermal comfort using calculation of the PMV and PPD indices and local thermal comfort criteria. Geneva: International Organization for Standards.
- Erikson, H., Krog, J., Andersen, K. L., & Scholander, P. F. (1956). The critical temperature in naked man. Acta Physiologica Scandinavica, 37(1), 35–39. doi: 10.1111/j.1748-1716.1956.tb01339.x
- Fanger, P. O. (1970). Thermal comfort. New York: Danish Technical University.
- Hansen, J. C., Gilman, A. P., & Odland, J. O. (2010). Is thermogenesis a significant causal factor in preventing the ‘globesity’ epidemic? Medical Hypotheses, 75(2), 250–256. doi:10.1016/j.mehy.2010.02.033
- Hanssen, M. J., Hoeks, J., Brans, B., van der Lans, A. A., Schaart, G., van den Driessche, J. J., … Schrauwen, P. (2015). Short-term cold acclimation improves insulin sensitivity in patients with type 2 diabetes mellitus. Nature Medicine, 21(8), 863–865. doi:10.1038/nm.3891
- Hanssen, M. J., van der Lans, A. A., Brans, B., Hoeks, J., Jardon, K. M., Schaart, G., … van Marken Lichtenbelt, W. D. (2016). Short-term cold acclimation recruits brown adipose tissue in obese humans. Diabetes, 65, 1179–1189. doi:10.2337/db15-1372
- Hardy, J. D., & Du Bois, E. F. (1938). Basal metabolism, radiation, convection and vaporization at temperatures of 22 to 35 ˚C. Journal of Nutrition, 15, 477–497.
- Hooper, P. L. (1999). Hot-tub therapy for type 2 diabetes mellitus. New England Journal of Medicine, 341(12), 924–925. doi:10.1056/NEJM199909163411216
- Jansky, L., Matouskova, E., Vavra, V., Vybiral, S., Jansky, P., Jandova, D., … Kunc, P. (2006). Thermal, cardiac and adrenergic responses to repeated local cooling. Physiological Research, 55(5), 543–549.
- Johnson, F., Mavrogianni, A., Ucci, M., Vidal-Puig, A., & Wardle, J. (2011). Could increased time spent in a thermal comfort zone contribute to population increases in obesity? Obesity Reviews, 12(7), 543–551. doi:10.1111/j.1467-789X.2010.00851.x
- Keith, S. W., Redden, D. T., Katzmarzyk, P. T., Boggiano, M. M., Hanlon, E. C., Benca, R. M., … Allison, D. B. (2006). Putative contributors to the secular increase in obesity: exploring the roads less traveled. International Journal of Obesity, 30(11), 1585–1594. doi: 10.1038/sj.ijo.0803326
- Kingma, B., Frijns, A., & van Marken Lichtenbelt, W. (2012). The thermoneutral zone: implications for metabolic studies. Frontiers in Bioscience, E4, 1975–1985. doi: 10.2741/e518
- Kingma, B., Schweiger, M., Wagner, A., & van Marken Lichtenbelt, W. D. (2017). Exploring internal body heat balance to understand 1 thermal sensation. Building Research & Information, 45(7). doi:10.1080/09613218.2017.1299996
- Kingma, B. R. M., Frijns, A. J. H., Saris, W. H. M., van Steenhoven, A. A., & van Marken Lichtenbelt, W. D. (2011). Increased Systolic blood pressure after mild cold and rewarming: relation to cold-induced thermogenesis and age. Acta Physiologica, 203(4), 419–427. doi:10.1111/j.1748-1716.2011.02336.x
- Knip, K. (2016, September 23). Waaron de septemberhitte in ‘59 erger voelde. NRC.
- Kolarik, J., Toftum, J., Olesen, B. W., & Jensen, K. L. (2011). Simulation of energy use, human thermal comfort and office work performance in buildings with moderately drifting operative temperatures. Energy and Buildings, 43(11), 2988–2997. doi: 10.1016/j.enbuild.2011.07.008
- Kox, M., van Eijk, L. T., Zwaag, J., van den Wildenberg, J., Sweep, F. C., van der Hoeven, J. G., & Pickkers, P. (2014). Voluntary activation of the sympathetic nervous system and attenuation of the innate immune response in humans. Proceedings of the National Academy of Sciences, 111(20), 7379–7384. doi:10.1073/pnas.1322174111
- van der Lans, A. A., Boon, M. R., Haks, M. C., Quinten, E., Schaart, G., Ottenhoff, T. H., & van Marken Lichtenbelt, W. D. (2015). Cold acclimation affects immune composition in skeletal muscle of healthy lean subjects. Physiological Reports, 3(7), e12394. doi:10.14814/phy2.12394
- van der Lans, A. A., Hoeks, J., Brans, B., Vijgen, G. H., Visser, M. G., Vosselman, M. J., … van Marken Lichtenbelt, W. D. (2013). Cold acclimation recruits human brown fat and increases nonshivering thermogenesis. Journal of Clinical Investigation, 123(8), 3395–3403. doi:10.1172/JCI68993
- van der Lans, A. A., Wierts, R., Vosselman, M. J., Schrauwen, P., Brans, B., & van Marken Lichtenbelt, W. D. (2014). Cold-activated brown adipose tissue in human adults: methodological issues. AJP: Regulatory, Integrative and Comparative Physiology, 307, R103–113. doi:10.1152/ajpregu.00021.2014
- Lee, P., Smith, S., Linderman, J., Courville, A. B., Brychta, R. J., Dieckmann, W., … Celi, F. S. (2014). Temperature-acclimated brown adipose tissue modulates insulin sensitivity in humans. Diabetes, 63(11), 3686–3698. doi:10.2337/db14-0513
- Lorenzo, S., & Minson, C. T. (2010). Heat acclimation improves cutaneous vascular function and sweating in trained cyclists. Journal of Applied Physiology, 109(6), 1736–1743. doi:10.1152/japplphysiol.00725.2010
- MacInnis, M. J., & Gibala, M. J. (2016). Physiological adaptations to interval training and the role of exercise intensity. Journal of Physiology. doi:10.1113/JP273196
- Makinen, T. M., Mantysaari, M., Paakkonen, T., Jokelainen, J., Palinkas, L. A., Hassi, J., … Rintamaki, H. (2008). Autonomic nervous function during whole-body cold exposure before and after cold acclimation. Aviation, Space, and Environmental Medicine, 79(9), 875–882. doi: 10.3357/ASEM.2235.2008
- van Marken Lichtenbelt, W. D., & Kingma, B. (2013). Building and occupant energetics: a physiological hypothesis. Architectural Science Review, 56(1), 48–53. doi: 10.1080/00038628.2012.759377
- van Marken Lichtenbelt, W. D., Kingma, B., van der Lans, A., & Schellen, L. (2014). Cold exposure – an approach to increasing energy expenditure in humans. Trends in Endocrinology and Metabolism, 25(104), 165–167. doi:10.1016/j.tem.2014.01.001
- van Marken Lichtenbelt, W. D., Schrauwen, P., Kerckhove, S., & Westerterp-Plantenga, M. S. (2002). Individual variation in body temperature and energy expenditure in response to mild cold. American Journal of Physiology, 282, E1077–1083.
- van Marken Lichtenbelt, W. D., Vanhommerig, J. W., Smulders, N. M., Drossaerts, M. A. F. L., Kemerink, G. J., Bouvy, N. D., … Teule, G. J. J. (2009). Cold-activated brown adipose tissue in healthy men. New England Journal of Medicine, 360(15), 1500–1508. doi: 10.1056/NEJMoa0808718
- van Marken Lichtenbelt, W. D., Westerterp-Plantenga, M. S., & van Hoydonk, P. (2001). Individual variation in the relation between body temperature and energy expenditure in response to elevated ambient temperature. Physiology & Behavior, 73, 235–242. doi: 10.1016/S0031-9384(01)00477-2
- Mavrogianni, A., Johnson, F., Ucci, M., Marmot, A., Wardle, J., Oreszczyn, T., & Summerfield, A. (2011). Historic Variations in Winter Indoor Domestic Temperatures and Potential Implications for Body Weight Gain. Indoor and Built Environment, 22, 360–375. doi: 10.1177/1420326X11425966
- McAllister, E. J., Dhurandhar, N. V., Keith, S. W., Aronne, L. J., Barger, J., Baskin, M., Benca, R. M., … Allison, D. B. (2009). Ten putative contributors to the obesity epidemic. Critical Reviews in Food Science and Nutrition, 49, 868–913. doi: 10.1080/10408390903372599
- Meex, R. C., Schrauwen-Hinderling, V. B., Moonen-Kornips, E., Schaart, G., Mensink, M., Phielix, E., … Hesselink, M. K. (2010). Restoration of muscle mitochondrial function and metabolic flexibility in type 2 diabetes by exercise training is paralleled by increased myocellular fat storage and improved insulin sensitivity. Diabetes, 59(3), 572–579. doi:10.2337/db09-1322
- Nicol, J. F., & Humphreys, M. A. (1973). Thermal comfort as part of a self-regulating system. Building Research and Practice, 6(3), 191–197. doi:10.1080/09613217308550237
- Nielsen, B., Hales, J. R., Strange, S., Christensen, N. J., Warberg, J., & Saltin, B. (1993). Human circulatory and thermoregulatory adaptations with heat acclimation and exercise in a hot, dry environment. Journal of Physiology, 460, 467–485. doi: 10.1113/jphysiol.1993.sp019482
- van Ooijen, A. M. J., van Marken Lichtenbelt, W. D., van Steenhoven, A. A., & Westerterp, K. (2004). Seasonal changes in metabolic and temperature responses to cold air in humans. Physiology & Behavior, 82, 545–553. doi: 10.1016/j.physbeh.2004.05.001
- Pallubinsky, H., Kingma, B. R. M., Schellen, L., Dautzenberg, B., van Baak, M., & van Marken Lichtenbelt, W. D. (2017). The effect of warmth acclimation on behaviour, thermophysiology and perception. Building Research & Information, 45(7). doi:10.1080/09613218.2017.1278652
- Pallubinsky, H., Schellen, L., Kingma, B., Dautzenberg, B., van Baak, M., & van Marken Lichtenbelt, W. D. (2017). Thermophysiological adaptations to passive mild heat acclimation. Temperature. doi:10.1080/23328940.2017.1303562
- Pandolf, K. B., Cadarette, B. S., Sawka, M. N., Young, A. J., Francesconi, R. P., & Gonzalez, R. R. (1988). Thermoregulatory responses of middle-aged and young men during dry-heat acclimation. Journal of Applied Physiology, 65(1), 65–71.
- Parkinson, T., & de Dear, R. (2015). Thermal pleasure in built environments: physiology of alliesthesia. Building Research & Information, 43(3), 288–301. doi: 10.1080/09613218.2015.989662
- Saito, M., Okamatsu-Ogura, Y., Matsushita, M., Watanabe, K., Yoneshiro, T., Nio-Kobayashi, J., … Tsujisaki, M. (2009). High incidence of metabolically active brown adipose tissue in healthy adult humans: effects of cold exposure and adiposity. Diabetes, 58(7), 1526–1531. doi: 10.2337/db09-0530
- Schellen, L., van Marken Lichtenbelt, W. D., Loomans, M. G., Toftum, J., & de Wit, M. H. (2010). Differences between young adults and elderly in thermal comfort, productivity, and thermal physiology in response to a moderate temperature drift and a steady-state condition. Indoor Air, 20(4), 273–283. doi: 10.1111/j.1600-0668.2010.00657.x
- Scholander, P. F., Hock, R., Walters, V., & Irving, L. (1950). Adaptation to cold in arctic and tropical mammals and birds in relation to body temperature, insulation, and basal metabolic rate. Biological Bulletin, 99(2), 259–271. doi:10.2307/1538742
- Schrauwen, P., & van Marken Lichtenbelt, W. (2016). Combatting type 2 diabetes by turning up the heat. Diabetologia, 59, 2269–2279. doi: 10.1007/s00125-016-4068-3
- Singh, G. M., Danaei, G., Farzadfar, F., Stevens, G. A., Woodward, M., Wormser, D., … Prospective Studies, C. (2013). The age-specific quantitative effects of metabolic risk factors on cardiovascular diseases and diabetes: a pooled analysis. PLoS ONE, 8(7), e65174. doi:10.1371/journal.pone.0065174
- Stanford, K. I., Middelbeek, R. J., Townsend, K. L., An, D., Nygaard, E. B., Hitchcox, K. M., … Goodyear, L. J. (2013). Brown adipose tissue regulates glucose homeostasis and insulin sensitivity. Journal of Clinical Investigation, 123(1), 215–223. doi:10.1172/JCI62308
- Taylor, N. A. (2014). Human heat adaptation. Comprehensive Physiology, 38(1), 325–365. doi:10.1002/cphy.c130022
- Te Kulve, M., Schellen, L., Schlangen, L. J., & van Marken Lichtenbelt, W. D. (2016). The influence of light on thermal responses. Acta Physiologica, 216(2), 163–185. doi:10.1111/apha.12552
- Unander, F., Ettestol, I., Ting, M., & Schipper, L. (2004). Residential energy use: an international perspective on long-term trends in Denmark, Norway and Sweden. Energy Policy, 32(12), 1395–1404. doi:10.1016/S0301-4215(03)00106-X
- Virtanen, K. A., Lidell, M. E., Orava, J., Heglind, M., Westergren, R., Niemi, T., … Nuutila, P. (2009). Functional brown adipose tissue in healthy adults. New England Journal of Medicine, 360(15), 1518–1525. doi: 10.1056/NEJMoa0808949
- Vosselman, M. J., Vijgen, G. H., Kingma, B. R., Brans, B., & van Marken Lichtenbelt, W. D. (2014). Frequent extreme cold exposure and brown fat and cold-induced thermogenesis: a study in a monozygotic twin. PLoS ONE, 9(7), e101653. doi:10.1371/journal.pone.0101653
- Warwick, P. M., & Busby, R. (1990). Influence of mild cold on 24 h energy expenditure in ‘normally’ clothed adults. British Journal of Nutrition, 63(3), 481–488. doi: 10.1079/BJN19900135
- Weller, A. S., Linnane, D. M., Jonkman, A. G., & Daanen, H. A. (2007). Quantification of the decay and re-induction of heat acclimation in dry-heat following 12 and 26 days without exposure to heat stress. European Journal of Applied Physiology, 102(1), 57–66. doi:10.1007/s00421-007-0563-z
- Yoneshiro, T., Aita, S., Matsushita, M., Kayahara, T., Kameya, T., Kawai, Y., … Saito, M. (2013). Recruited brown adipose tissue as an antiobesity agent in humans. Journal of Clinical Investigation, 123(8), 3404–3408. doi:10.1172/JCI67803
- Yoneshiro, T., Aita, S., Matsushita, M., Okamatsu-Ogura, Y., Kameya, T., Kawai, Y., … Saito, M. (2011). Age-related decrease in cold-activated brown adipose tissue and accumulation of body fat in healthy humans. Obesity, 19(9), 1755–1760. doi:10.1038/oby.2011.125
- Zhang, H., Arens, E., & Zhai, Y. (2015). A review of the corrective power of personal comfort systems in non-neutral ambient environments. Building and Environment, 91, 15–41. doi: 10.1016/j.buildenv.2015.03.013