Abstract
Aeroterrestrial green algae are among the most ubiquitous members of the microbial flora colonizing aerial surfaces. Filamentous green algae, in particular, produce large populations in several natural and artificial habitats. In recent years it has been shown that the bases of the walls of urban environments are frequently colonized by filamentous green algae. However, information concerning the physiology of these organisms and the factors that determine their distribution is extremely limited. We studied the physiological responses of a strain of Klebsormidium sp. (Klebsormidiales, Klebsormidiophyceae) collected in an urban area (Konstanz, Germany). Growth responses, photosynthetic performance, desiccation tolerance and accumulation of organic osmolytes were measured in several different combinations of environmental factors. Klebsormidium sp. exhibited optimal growth and highest photosynthetic efficiency under relatively low photon fluence rates, performing optimally between 15 and 30 µmol photons m−2 s−1 and showing increasing inhibition above 85 µmol photons m−2 s−1. Although it could survive at salinities up to 60 psu, this alga was relatively stenohaline; growth was optimal between 1.2 and 15 psu and declined considerably at higher salinities. Sucrose acted as the major organic osmolyte, and its concentration increased almost linearly between 1.2 and 30 psu from 29.7 to 171.2 µmol g−1 dry weight. Further increases in salinity, however, were accompanied by a strong reduction in sucrose content pointing to insufficient osmotic adjustment. Klebsormidium sp. was able to photosynthesize efficiently for up to 2 hours under complete desiccation. Afterwards, however, there was no measurable photosynthetic activity although complete photosynthetic efficiency (F v/F m) was recovered upon rehydration for 48 hours. Overall, the results correlate well with the ecology of Klebsormidium sp. in the field and its distribution in continental Europe.
Introduction
Although most green algae typically inhabit aquatic habitats such as lakes, rivers and the oceans, some taxa are also widespread in terrestrial environments (Ettl & Gärtner, Citation1995). Here, these so-called aeroterrestrial green algae colonize the interface between a wide range of terrestrial surfaces and the atmosphere. They participate in symbiotic associations with fungi, forming lichens (e.g. Trebouxia; Friedl, Citation1997), or grow on (and partly in) various natural surfaces such as the bark of trees, rocks and soil (Bell, Citation1993; Belnap & Lange, Citation2001; Johansen & Shubert, Citation2001; Büdel, Citation2005; Cardon et al., Citation2008, and references therein). In urban areas, extensive growth on man-made surfaces such as roof tiles, building facades and concrete pathways may become a biofouling problem (Rindi & Guiry, Citation2004; Eggert et al., Citation2006; Karsten et al., Citation2007b ). Whether aeroterrestrial green algae actively corrode surface materials remains the subject of debate (Ortega-Calvo et al., Citation1995), but the aesthetic damage caused by their patinas and incrustations is unquestionable. Furthermore, the growth of aeroterrestrial algae causes retention of humidity and accumulation of organic matter, which favour the growth of lichens, fungi and other organisms for which a direct effect of biodeterioration has been demonstrated (Ortega-Calvo et al., Citation1995; Gaylarde & Morton, Citation1999; Rindi, Citation2007).
The information available on distribution and ecology of aeroterrestrial green algal assemblages is rather limited, particularly for urban environments (Rindi, Citation2007, and references therein). Rindi & Guiry (Citation2004) undertook a comprehensive survey of the composition and spatial variability of these algae in various northern and southern European cities. Most interestingly, these authors reported filamentous green algae as the most abundant morphotypes in the algal assemblages colonizing the bases of urban walls. However, while in the more rainy and cool Atlantic regions of Europe these communities were formed by filamentous forms of the genera Prasiola and Rosenvingiella (Prasiolales, Trebouxiophyceae), in continental and Mediterranean regions species of the genus Klebsormidium P.C. Silva, Mattox et Blackwell (Klebsormidiophyceae, Streptophyta) (Silva et al., Citation1972) were dominant (Rindi & Guiry, Citation2004). Based on these distributional data, Rindi & Guiry (Citation2004) distinguished a Prasiolales assemblage and a Klebsormidium assemblage. These authors suggested that the physiological responses of different species in relation to different climatic regimes may play a fundamental role in controlling the relative distribution of these assemblages. However, Rindi & Guiry (Citation2004) did not have any physiological data allowing a direct correlation with climatic variables.
Compared with the aquatic environment, aeroterrestrial green algae are exposed to harsher environmental conditions, such as diurnally and seasonally high insolation, strong temperature fluctuations and restricted water availability (Karsten et a l., Citation2007 a, Citation2007b ). Extensive exposure to the atmosphere results in dehydration, which strongly affects photosynthesis and growth. While optimal growth and photosynthesis occur in liquid culture conditions, aeroterrestrial green algae can dry to inactivity, then fully recover when rehydrated (Häubner et al., Citation2006). Water availability, which includes rainfall precipitation, condensation and water vapour, is therefore the key ecological prerequisite for long-term survival of these organisms, because only fully hydrated cells are physiologically functional. Although the extent of water loss from algae in terrestrial habitats is related to external climatic conditions, internal factors such as surface to volume ratio, thick cell walls, mucilage layers, ‘self-protection’ by cell aggregates or biosynthesis/accumulation of ‘water-holding’ organic molecules (e.g. compatible solutes) also affect the ability of algal cells to retain cellular water (Yancey, Citation2005; Flechtner, Citation2007; Karsten et al., Citation2007b ; Rindi, Citation2007; Gustavs et al., Citation2009a ). The presence of one or more of these mechanisms provides some explanation for the ecological success of aeroterrestrial green algae in their specific habitats.
Species of the genus Klebsormidium represent one of the most widespread groups of aeroterrestrial microchlorophytes in the world (see Rindi et al., Citation2008), ranging in distribution from polar to tropical habitats. Taxonomy of this genus is a challenge, because of the morphological simplicity and probable hidden high cryptic diversity (Rindi et al., Citation2008). Consequently, species identification in Klebsormidium is still a difficult task, although it is a prerequisite for phylogenetic as well as for ecophysiological studies. Although many ultrastructural and molecular studies confirm the position of Klebsormidium in the streptophytan lineage along with higher plants (Rindi et al., Citation2008 and references therein), and often highlight its high ecological importance, only few data exist on ecophysiological performance under a range of environmental parameters.
Klebsormidium flaccidum from a dune soil crust can survive in dehydrated conditions and is able to maintain 80–90% of photosynthetic CO2 fixation for some time after almost complete loss of cellular water (De Winder et al., Citation1990). However, several days without liquid water led to complete inhibition of CO2 fixation, while rehydration was accompanied by fast and full recovery (De Winder et al., Citation1990), indicating efficient, but still unstudied protective mechanisms against desiccation. In contrast to desiccation, cold acclimation and freezing resistance in various Klebsormidium strains are better understood (Elster et al., Citation2008; Nagao et al., Citation2008). In K. flaccidum the accumulation of various low molecular weight carbohydrates (LMWCs) and amino acids together with ultrastructural changes such as chloroplast enlargement, vacuole size reduction, etc. contribute to an enhanced freezing tolerance (Nagao et al., Citation2008). However, to date no detailed data on the ecophysiological performances of Klebsormidium have been obtained.
In the present study, for the first time, an urban strain of Klebsormidium sp. from concrete at the base of an old wall in Konstanz, Germany, was ecophysiologically investigated in terms of desiccation tolerance. In addition, growth and photosynthesis were evaluated under a range of photon flux densities (PFDs) and salinities to determine the respective tolerances. The data explain the widespread abundance of Klebsormidium sp. in continental Europe.
Materials and methods
Algal material
The strain of Klebsormidium sp. used in this study was collected by Dr Ylenia Chiari and mailed to the second author, who identified it and established a uni-algal culture. This isolate was removed from a population forming green patches at the base of an urban wall in the city centre of Konstanz, Germany, on 26 August 2005. The alga was originally isolated into culture using Jaworski's Medium (Tompkins et al., Citation1995) and maintained in the culture collection of the National University of Ireland, Galway; a voucher specimen is deposited in the phycological herbarium of the National University of Ireland, Galway (GALW 015499). The material transferred to Rostock was grown in 500 ml Erlenmeyer flasks filled with modified Bold's Basal Medium (3 NMBBM) (Starr & Zeikus, Citation1993; BBM modified by addition of triple nitrate concentration) and kept at 20°C and 30–35 µmol photons m−2 s−1 under a light/dark cycle of 16:8 h L:D. Osram Daylight Lumilux Cool White lamps (L36W/840) were used as light sources. Radiation measurements were carried out with a Solar Light PMA 2132 cosine corrected PAR sensor connected to a Solar Light PMA 2100 radiometer (Solar Light Co., Inc., Philadelphia, USA). Log-phase stock cultures were used for all experiments. The rbcL gene of this strain was sequenced as part of the study of Rindi et al. (Citation2008). In order to ensure that the strain was not swapped or contaminated during its maintenance in Rostock, it was re-amplified in this study using the same primers and PCR protocols of Rindi et al. (Citation2008); PCR products were sequenced commercially by Eurofins Genetic Services Ltd. (Wolverhampton, UK). The rbcL sequence obtained was identical to that of Rindi et al. (Citation2008), confirming the identity of the strain. Morphologically, the strain is close to Klebsormidium flaccidum; at present, however, problems with the taxonomy of the genus Klebsormidium (explained in detail by Rindi et al., Citation2008) prevent an unambiguous identification to species level.
Growth measurements
Growth responses under a range of photon fluence densities and salinities were followed using an in vivo growth fluorometer (Hansatech MFMS, Norfolk, UK) according to the methodological approach of Gustavs et al. (Citation2009b ), which monitors increases of in vivo chlorophyll a fluorescence Ft over time as an indicator for biomass accumulation (Karsten et al., Citation1996). Fluorescence measurements were performed every 24 h for up to 10 days. Disposable Petri dishes with transparent lids (Licefa GmbH & Co. KG, Bad Salzuflen, Germany) filled with 20 ml medium (3 NMBBM for light experiments, 3 NMBBM plus different sea salt concentrations for salinity experiments) were used as incubation vessels. All measurements started with the addition of 150–200 µl log-phase stock culture. Growth rate μ was calculated as the mean value from replicate samples using the equation Ft = F 0 eµt (F 0: initial fluorescence; Ft : fluorescence after t days) for each individual sample (Gustavs et al., Citation2009b ).
For the light experiments PFDs of 4, 8, 15, 30, 85 and 110 µmol photons m−2 s−1 were applied under a light/dark cycle of 16:8 h L:D using the Osram lamps mentioned above. The PFDs were adjusted by varying the distance between light source and Petri dish and by using neutral grey plastic filter foil (Lichttechnik Hahne, Duesseldorf, Germany). Algae were grown in the stock culture medium at 20°C.
Saline 3 NMBBM (1.2 (control, normal 3 NMBBM), 5, 15, 30, 45, 60 psu) media were prepared by adding respective concentrations of artificial sea salt (Sel marin hw professional, Wiegandt GmbH, Krefeld, Germany). Salinity was controlled using a refractometer. All salinity treatments were carried out at 20°C and 30–35 µmol photons m−2 s−1 under a light/dark cycle of 16:8 h L:D.
Photosynthesis measurements
Photosynthetic oxygen evolution rates under increasing photon fluence densities (PI curves) were measured with a Presens Fibox 3 oxygen optode (Presens, Nürnberg, Germany) using a 3 ml thermostatic acrylic chamber DW1 (Hansatech Instruments, UK) at 20°C combined with a magnetic stirrer according to Remias et al. (Citation2010). Three ml of log-phase algal suspension were enriched with HCO3 (2 mM final concentration) before each measurement to ensure sufficient carbon supply. Nine PFDs ranging from 0 to 500 µmol photons m−2 s−1 PAR provided by a halogen lamp were calibrated with a Hansatech QRT1 PAR sensor inside the cuvette and adjusted with Hansatech A5 neutral glass filters. The O2 production per unit PFD and time was referenced to the concentration of total chlorophyll a per sample. After each PI curve measurement, cell suspension was filtered on a Whatman GF/F glass fibre filter using a glass Pasteur pipette. Chlorophyll a on the filters was extracted with dimethyl formamide (DMF) and photometrically quantified using the equation of Porra et al. (Citation1989). PI curve data were calculated and fitted by the mathematical photosynthesis model of Webb et al. (Citation1974).
In vivo chlorophyll fluorescence was measured in samples treated for 72 h at 20°C at different salinities using a pulse-amplitude modulated fluorimeter (PAM 2000, Heinz Walz GmbH, Effeltrich, Germany). The optimum quantum yield (F v/F m) of photochemistry was determined in five replicate samples, each of which was concentrated on a Whatman GF/F glass fibre filter by mild filtration and kept moist with the respective saline medium during the fluorescence measurements. Determination of F v/F m was conducted as described by Holzinger et al. (Citation2006). After exposure for 10–15 min to darkness, minimal fluorescence (F 0) was measured with a pulsed measuring beam (approximately 0.3 µmol photons m−2 s−1, 650 nm), followed by short pulses of saturating white light (0.4–0.8 s, 1000–5000 µmol photons m−2 s−1) to record F m (F v = F m − F 0).
Desiccation experiments
Log-phase cultures were concentrated on 5 replicate Whatman GF/F glass fibre filters by mild filtration and air-dried for 2 h on a rack under ambient room temperatures at about 22°C and 20–25 µmol photons m−2 s−1. The optimum quantum yield (F v/F m) of the algae was regularly determined during the desiccation period using the PAM 2000 as described above. The distance between fibre optic and filter surface was always kept constant at 5 mm. After the desiccation period, the dried filters were rehydrated by immersion in standard growth medium and recovery of F v/F m was followed.
Organic osmolyte analysis
Algal material in 300 ml Erlenmeyer flasks was treated for 72 h with the saline 3 NMBBM (1.2 (control, normal 3 NMBBM), 5, 15, 30, 45, 60 psu) media used for the growth experiments (see above), and kept at 20°C and 30–35 µmol photons m−2 s−1 under a light/dark cycle of 16:8 h L:D. Afterwards filaments were harvested by filtration onto Whatman GF/C glass fibre filter following mild oven-drying at 50°C overnight. These samples were stored under dark, dry and cool conditions prior to HPLC analysis of LMWCs. For HPLC algal samples of 10–20 mg dry weight were extracted with 70% aqueous ethanol (v/v) in capped centrifuge tubes at 70°C in a water bath for 4 h according to Karsten et al. (Citation1991a ). After centrifugation for 5 min at 5000 g, 700 µl of the supernatant were evaporated to dryness under vacuum (Speed Vac Concentrator SVC 100H). Dried extracts were re-dissolved in 700 µl distilled water and vortexed for 30 s. Samples were analysed with an isocratic Agilent HPLC system equipped with a differential refractive index detector. LMWCs were separated and quantified on a Bio-Rad resin-based column (Aminex Fast Carbohydrate Analysis, 100 × 7.8 mm) using a Phenomenex Carbo-Pb2+ (4 × 3 mm) guard cartridge. LMWCs were eluted with 100% HPLC grade water at a flow rate of 1 ml min−1 at 70°C (modified after Karsten et al., Citation1991a ). Sucrose could be identified as the major LMWC by comparison of retention times with those of a commercial standard compound (Roth, Karlsruhe, Germany), prepared as 1 mM aqueous solution and quantified by peak areas. All concentrations are expressed in µmol g−1 dry weight.
Data analysis
All experiments were carried out with three to five independent replicates and data are shown as means and standard deviations. Salinity and light effects on growth rates, and salinity effects on sucrose content, as well as desiccation and salinity effects on optimum quantum yield were analysed using one-way ANOVAs. The Tukey test was used to find a posteriori homo-geneous sub-groups of means that differed significantly. Data analysis was performed using InStat (GraphPad Software Inc., La Jolla, CA, USA).
Results
Klebsormidium sp. grew under all PFDs tested ranging from 4 to 110 µmol photons m−2 s−1 (). At the lowest light level (4 µmol photons m−2 s−1) a growth rate of 0.13 (µ d−1) was recorded. Increasing PFDs up to 15 µmol photons m−2 s−1 were accompanied by an almost linear rise in growth rates. However, a further increase in PFD to 30 µmol photons m−2 s−1 was accompanied by a small, but insignificant stimulation of the growth rate (0.39 µ d−1) (). Treatment with 85 and 110 µmol photons m−2 s−1 led to a strong reduction in growth rates. At 85 µmol photons m−2 s−1 0.28 µ d−1 were measured, and at 110 µmol photons m−2 s−1 growth rate further decreased to 0.19 µ d−1 (). All these data indicate low light requirements of Klebsormidium sp. for growth.
Fig. 1. Growth rates (µ d−1) as a function of photon fluence density (a) and of salinity (b) in Klebsormidium sp. (n = 4, mean value ± SD). In vivo growth was measured using a fluorometer according to the technique of Gustavs et al. (Citation2009b ). Significance of differences among the treatments was calculated by one-way ANOVA (P < 0.001). Different letters represent significant differences among the photon fluence rates as revealed by Tukey's post hoc test. psu, practical salinity units.
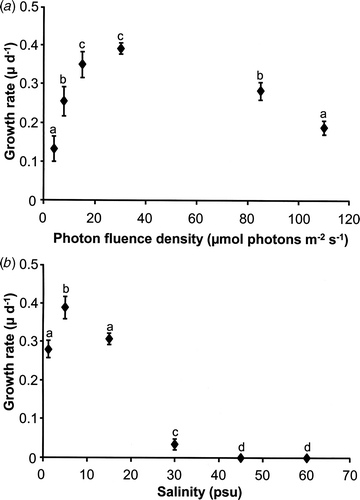
Salinity had a highly significant effect on growth rates of Klebsormidium sp. between 1.2 and 60 psu (P < 0.001; ). This isolate grew in a rather narrow salinity range from 1.2 to 30 psu with a distinct maximum at 5 psu (0.39 ± 0.03 µ d−1). While growth rates at 1.2 and 15 psu were similar, 0.28 and 0.31 µ d−1, respectively, and hence only slightly different from the optimum, growth was strongly inhibited at 30 psu (0.04 µ d−1) (). Further increases in salinity were accompanied by a complete inhibition of growth (). Although Klebsormidium sp. did not grow under these hypersaline conditions, it survived 45 psu while at 60 psu many filaments became bleached (data not shown).
Photosynthetic oxygen evolution in Klebsormidium sp. under increasing PFDs led to a PI curve from which characteristic parameters for the description of the light requirements could be derived using the model of Webb et al. (Citation1974) for the data set (). The inset shows the initial slope alpha (photosynthetic efficiency) and the light compensation Ic in the light-limited section (). While the respiration rate amounted for −4.5 µmol O2 h−1 mg−1 Chl. a, maximum photosynthetic rate Pmax in the light-saturated range was 59.9 µmol O2 h−1 mg−1 Chl. a, which resulted in a photosynthesis:respiration (P:R) ratio of 13.3. Even under the highest PFD tested (500 µmol photons m−2 s−1) no photoinhibition could be measured. Klebsormidium sp. exhibited an alpha value of 7.3 µmol O2 h−1 mg−1 Chl. a (µmol photons m−2 s−1)−1, an Ic value of 2.1 µmol photons m−2 s−1 and an Ik (initial light saturation point) value of 8.8 µmol photons m−2 s−1 (), all of which clearly indicate low light requirements for photosynthesis.
Fig. 2. Photosynthetic oxygen evolution as function of increasing photon fluence densities in Klebsormidium sp. (n = 3, mean value ± SD) according to the method of Remias et al. (Citation2010). The dashed line represents a fitted curve of the data measured based on the photosynthetic equation of Webb et al. (Citation1974).
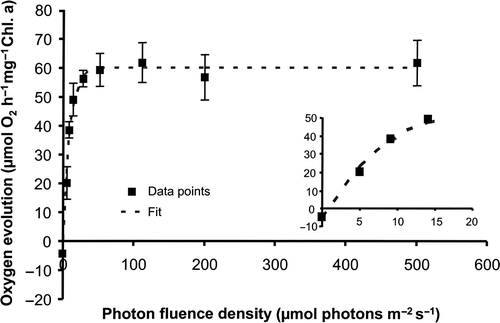
The maximum PSII quantum efficiency F v/F m was salinity dependent in Klebsormidium sp. (P < 0.001; ). This strain showed similar F v/F m values in the salinity range between 5 and 30 psu (0.57). At 1.2 and 45 psu slightly lower F v/F m values of 0.43 and 0.47, respectively, were measured (). Under the highest salinity tested (60 psu), F v/F m strongly decreased to 0.07 indicating photoinhibition.
Fig. 3. Changes in photosystem II efficiency (F v/F m: optimum quantum yield) of Klebsormidium sp. (n = 5, mean value ± SD) after 72 h exposure to a range of salinities. Significance of differences among the treatments was calculated by one-way ANOVA (P < 0.001). Different letters represent significant differences among the salinities as revealed by Tukey's post hoc test. psu, practical salinity units.
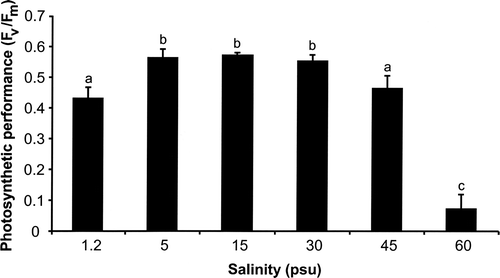
HPLC analysis revealed sucrose as the major peak in extracts of Klebsormidium sp. treated under a range of salinities (). Intracellular sucrose concentrations increased significantly and almost linearly from 29.7 to 171.2 µmol g−1 dry weight between 1.2 and 30 psu (P < 0.001). However, treatment with hypersaline media (45 and 60 psu) led to decreasing sucrose values of 123.5 and 107.2 µmol g−1 dry weight, respectively ().
Fig. 4. Changes in sucrose concentration (µmol g−1 dry weight) of Klebsormidium sp. (n = 4, mean value ± SD) after 72 h exposure to a range of salinities. Significance of differences among the treatments was calculated by one-way ANOVA (P < 0.01). Different letters represent significant differences among the salinities as revealed by Tukey's post hoc test. psu, practical salinity units.
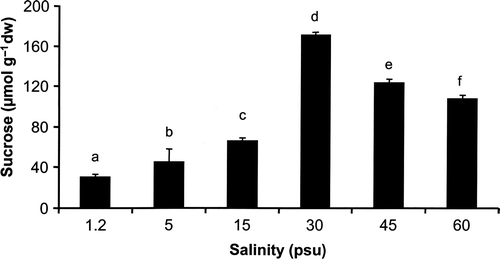
Klebsormidium sp. was air-dried for 2 h under ambient room temperature conditions (about 55% relative air humidity) followed by rehydration and recovery over 48 h. During this desiccation experiment the maximum PSII quantum efficiency F v/F m was recorded at different intervals (). While 1 h air-drying did not affect photosynthetic performance (F v/F m values of about 0.6), after 2 h treatment maximum PSII quantum efficiency was not measurable (F v/F m = 0). After rehydration of the dried samples small initial F v/F m values between 0.03 and 0.09 could be estimated within the first hour followed by further but slow recovery after 2 to 4 h (F v/F m values 0.19–0.22) (). Full recovery to the original maximum PSII quantum efficiency values took at least 48 h ().
Fig. 5. Changes in photosystem II efficiency (F v/F m: optimum quantum yield) of Klebsormidium sp. (n = 4, mean value ± SD) during 2 h desiccation under ambient room temperature conditions (c. 22°C) followed by 48 h recovery after rehydration with stock culture medium. Significance of differences among the treatments was calculated by one-way ANOVA (P < 0.001). Different letters represent significant differences among the time points as revealed by Tukey's post hoc test.
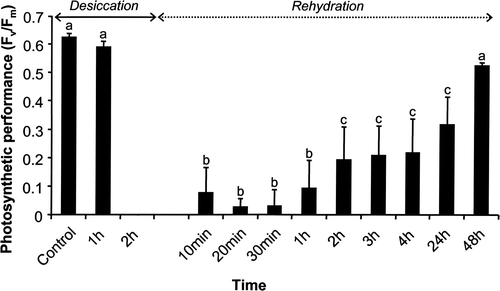
Discussion
Members of the green algal genus Klebsormidium are characterized by an extremely widespread occurrence in freshwater and terrestrial habitats from polar to tropical, and from humid to arid regions (Rindi et al., Citation2008). Of particular ecological importance is their contribution to biological soil crust communities in arid and semi-arid habitats. In these environments Klebsormidium spp. form, along with other photo- and heterotrophic organisms, an intimate association with soil particles and together play significant roles in primary production, nutrient cycling, water retention and stabilization of soils (Evans & Johansen, Citation1999; Lewis, Citation2007). Because of the rather simple morphology with very few diagnostic characters, the systematics of Klebsormidium has long been problematic, and is still not solved (Rindi et al., Citation2008). Based on rbcL gene analysis these authors reported a much higher genetic diversity than expected from morphology. Therefore, it is reasonable to assume that some Klebsormidium entities, as currently circumscribed, will prove to be a complex of morphologically similar species, rather than independent taxa. If this assumption holds true, reassessment will be required, not only of the taxonomic identity of many records, but also for the few reports on ecophysiological performance and biochemical properties.
At terrestrial, urban sites in Europe, species of Klebsormidium preferentially inhabit locations at the bases of old, weathered north-facing walls, exposed to limited amounts of full sunlight (Rindi & Guiry, Citation2004). While most urban environments with high abundances of prasiolalean algae (Prasiola spp., Rosenvingiella spp.), are located in coastal Atlantic regions of Europe typically with high mean rainfall (Rindi et al., Citation2004; Menendez et al., Citation2006), Klebsormidium assemblages preferentially occur in continental Europe, for example, southern Spain and Italy, where the climate is less damp (Ortega-Calvo et al., Citation1991; Rindi & Guiry, Citation2004). These data already indicate differences in desiccation tolerance of these algal groups, and the habitat description correlates well with the ecophysiological data of the present study.
The growth rates of the aeroterrestrial Klebsormidium sp. isolate under increasing PFDs clearly indicate an optimum between 15 and 30 µmol photons m−2 s−1 as well as increasing inhibition above 85 to 110 µmol photons m−2 s−1 (), pointing to a pronounced low-light adaptation. Although light requirements for growth of aeroterrestrial algae are generally little studied, the few investigations available indicate at least similar values. Aeroterrestrial filamentous green algal members of the genus Trentepohlia, such as T. odorata and T. aurea, exhibited maximum growth rates at 122 µmol photons m−2 s−1 (Tan et al., Citation1993) and at 3000 lux (c. 60 µmol photons m−2 s−1) (Abe et al., Citation1998), respectively. Otherwise the low light requirements for growth in Klebsormidium sp. are quite similar to algae from shaded freshwater habitats such as Klebsormidium rivulare, Vaucheria bursata, etc. (Leukart & Hanelt, Citation1995).
Under natural conditions filamentous green algae such as Klebsormidium sp. typically form multi-layered mat-like structures which most probably contribute to a high degree of self-shading of individual filaments inside such a population. More recent data suggest that shading effects due to such three-dimensional spatial arrangements may be more important than appreciated in the past. Gray et al. (Citation2007) reported a very interesting case in terrestrial green algae occurring in biotic crusts of North American deserts. Despite their ability to withstand desiccation, these algae were susceptible to photodamage at a PFD of 130 µmol m−2 s−1, which is quite low if compared with the full sunlight to which these crusts are often exposed. Gray et al. (Citation2007) suggested that in the field these algae may occupy microenvironments within the crust where they are protected from damaging light levels; the differential susceptibility to irradiance expressed by individual taxa suggests that there may be a complex spatial arrangement of green algal species in the crust, structured in response to the attenuation of light with depth through the crust profile. Although self-shading is poorly understood in aeroterrestrial green algal assemblages, it has been well investigated in Ulva macroalgal canopies in southern Spain, where the top layer of the mat-like canopies is generally completely bleached thereby providing photoprotection for subcanopy thalli (Bischof et al., Citation2002).
The photosynthesis–irradiance (PI) curves of Klebsormidium sp. are in full agreement with the low light requirements for growth (). The high alpha and low Ic and Ik values clearly indicate adaptation to very low PFDs, which are common at the natural sites. In most macroalgae low light requirements for photosynthesis are typically coupled to strong photoinhibition under higher photon fluence densities (Hanelt et al., Citation1997 and references therein; Bischof et al., Citation1998). However, although alpha, Ic and Ik in Klebsormidium sp. reflect pronounced low light adaptation, no indication of expected photoinhibition could be noted, which may be interpreted as high photophysiological plasticity. Similar Ik values were reported for the aeroterrestrial Prasiola crispa collected from a rocky cliff underneath a seagull colony on Spitsbergen (Holzinger et al., Citation2006). In addition, two aeroterrestrial Stichococcus strains, ROS 474 and ROS 553, isolated from building facades in northern Germany (Karsten et al., Citation2005), showed also low Ik values between 41 and 49 µmol photons m−2 s−1 (Evelyn Lawrenz, unpublished results). The low light requirements for growth, as already mentioned above, are also reflected in the respective PI curves of macroalgae from light-protected habitats such as streams (Leukart & Hanelt, Citation1995).
Klebsormidium sp. is capable of growing well between 1.2 and 15 psu, while at 30 psu there was a marked reduction in growth rate (). These data point to a rather stenohaline response pattern. Although many freshwater algal species are typically described as being stenohaline with strong growth inhibition under moderate osmotic stress (e.g. Chlorella vulgaris at 6–12 psu: Setter & Greenway, Citation1979), other freshwater as well as aeroterrestrial algal strains can grow even at fully marine salinities (e.g. Stichococcus bacillaris: Brown & Hellebust, Citation1980; Chlorella emersonii (actual designation Scenedesmus vacuolatus): Setter & Greenway, Citation1979; Rosenvingiella radicans: Rindi et al., Citation2004). In a more recent study Gustavs et al. (Citation2009a ) reported on growth in response to changing water availabilities (caused by salinity stress) in five aeroterrestrial green algal species (Trebouxiophyceae) isolated from roof tiles and building facades. Although all investigated strains exhibited maximum growth under brackish conditions, they were capable of growing over a broad salinity range between 0 and 40–65 psu, pointing to rather euryhaline response patterns. Even though most taxa of aeroterrestrial algae are believed to have evolved from freshwater ancestors, the study of Gustavs et al. (Citation2009a ) clearly indicates that all investigated strains can cope well with strong osmotic stress.
Aeroterrestrial green algal species of the Trebouxiophyceae have recently been reported to synthesize and accumulate polyols as organic osmolytes which play a vital role in the process of osmotic acclimation (Gustavs et al., Citation2009a ; Darienko et al., Citation2010), and which may contribute to desiccation tolerance (Oren, Citation2007). Polyols exert multiple functions in metabolism, i.e. besides their roles as organic osmolytes, they can also act as compatible solutes, antioxidants, heat protectants (stabilization of proteins), and rapidly available respiratory substrates (energy supply for a maintenance metabolism under stress and for repair processes) (Yancey, Citation2005, and references therein; Karsten et a l., Citation2007 b). The major physiological goal is to keep homeostasis as long as possible stable, and to minimize or repair any damage of the algal cells as fast as possible. Aeroterrestrial green algal taxa such as Chloroidium, Coccomyxa, Prasiola, Stichococcus and Trentepohlia have been found to synthesize and accumulate high concentrations of a range of sugar alcohols such as glycerol, erythritol, ribitol, arabitol, mannitol, sorbitol and volemitol (Feige & Kremer, Citation1980; Gustavs et al., Citation2009a ; Darienko et al., Citation2010). In contrast, analysis of Klebsormidium sp. extracts clearly indicated the lack of occurrence of any polyol, which fits well with its stenohaline features. Instead of polyols the disaccharide sucrose represented the major organic osmolyte in the investigated Klebsormidium strain (). However, sucrose was only regulated between 1.2 and 30 psu, while higher salinities were accompanied by a decrease in concentration. These data explain the growth inhibition at 45 and 60 psu (). Although sucrose usually occurs as the principal osmolyte in cyanobacteria and green macroalgae (Kirst, Citation1990; Karsten et al., Citation1991b , Citation1996) it is known to interfere at higher concentrations with various enzymes such as isocitrate dehydrogenase (Brown & Simpson, Citation1972), most probably because of the stereochemistry of the ring-form (Borowitzka, Citation1986).
Compared with aquatic environments, aeroterrestrial algae are regularly exposed to harsher environmental conditions such as desiccation, which strongly affects photosynthesis and growth. In many regions of Europe, for example, water availability frequently fluctuates from fluid droplets after rain or snow, to extended periods of dryness during summer or freezing during winter. Water availability is therefore the key ecological prerequisite for long-term survival of aeroterrestrial algae, because only fully hydrated cells will be physiologically functional. Although the degree of water loss from algae in terrestrial habitats is related to external climatic parameters, internal factors also affect the ability of algal cells to retain cellular water. These factors include the algal surface to volume ratio, morphological features such as thick cell walls and mucilage layers or ‘self-protection’ by cell aggregates (e.g. Apatococcus), cell colonies (e.g. Coccomyxa), the formation of biofilms and of biotic crusts (Karsten et al., Citation2007b ).
Air-drying of Klebsormidium sp. for only 2 h resulted in a complete inhibition of the maximum PSII quantum efficiency (F v/F m). Full recovery after rehydration of the dried samples took at least 48 h (), indicating a rather moderate tolerance. In situ photosynthetic performance of an aeroterrestrial green algal biofilm colonizing a building facade and dominated by unicellular taxa was investigated over 6 months, with an emphasis on changing water availability and air humidity (Häubner et al., Citation2006). The measured F v/F m values indicated diurnal activity patterns, i.e. on most sampling dates the photosynthetic performance was particularly high in the morning. This activity profile of the green algal biofilm correlated with the presence of condensation water onto the facade. With increasing solar radiation during the day, the water film evaporated, leading to desiccation of the microalgal organisms and quick inhibition of photosynthesis. However, in contrast to Klebsormidium sp., recovery of photosynthesis in dried algal cells occurred within minutes after artificial moistening (Häubner et al., Citation2006). Similar high tolerance against desiccation has been described for other aeroterrestrial green algae such as Apatococcus lobatus (Bertsch, Citation1966), Klesormidium flaccidum (De Winder et al., Citation1990) and various desert green algae (Gray et al., Citation2007). Klebsormidium flaccidum can survive in dehydrated conditions and can maintain 80–90% of photosynthetic CO2 fixation for some time after almost complete loss of cellular water (De Winder et al., Citation1990). Desert green algae even survived desiccation for at least 4 weeks when dried in darkness and already exhibited high photosynthetic recovery rates only 1 h after rehydration while their aquatic pendants recovered very little (Gray et al., Citation2007). Compared with the high desiccation tolerance and recovery potential of these aeroterrestrial green algae, the studied Klebsormidium sp. appears rather sensitive; this seems to be in agreement with the type of habitat where this strain and other strains with identical rbcL sequence were collected (urban walls and corners, mainly in northern European cities, at sites sheltered from direct sunlight most of the time; Rindi & Guiry, Citation2004; Rindi et al., Citation2008). Although some physiological data on desiccation in algae exist, the underlying mechanisms are not fully understood (Alpert, Citation2006). In contrast, the terrestrial cyanobacterium Nostoc commune has been developed as an excellent prokaryotic model system to understand desiccation and other stress tolerances at the biochemical and molecular level (Potts, Citation1999; Wright et al., Citation2005).
In conclusion, the urban strain of Klebsormidium sp. investigated in the present study exhibits low light requirements for growth and photosynthesis, stenohaline features, a limited capacity for osmotic acclimation and a moderate desiccation tolerance which explains its occurrence at various urban sites in Europe. However, these habitats have to provide protection against direct sunlight and sufficient water availability to guarantee long-term survival of this ecophysiologically less robust species. In addition, further information on inter- and infraspecific differentiation in members of Klebsormidium is urgently needed, to better understand physiological plasticity and adaptive strategies of this cosmopolitan genus.
Acknowledgements
The authors are grateful to Dr Ylenia Chiari (University of Montpellier), who collected the strain used for this study, Dr Daniel Remias (University of Innsbruck) for technical support with the photosynthetic oxygen measurements, Mónica Moniz for assistance with molecular work and Juliane Buss for HPLC analysis. This study was undertaken during Ulf Karsten's sabbatical at the University of Innsbruck in Prof. Cornelius Lütz's laboratory. Financial support by the Deutsche Forschungsgemeinschaft (DFG) (KA899/16-1/2) is gratefully acknowledged. Fabio Rindi is grateful to The Marine Institute of Ireland for financial support under the National Marine Biodiscovery Program at the time of writing of the manuscript.
References
- Abe , K , Mihara , H and Hirano , M . 1998 . Characteristics of growth and carotenoid accumulation of the aerial microalga Trentepohlia aurea in liquid culture . J. Mar. Biotechnol. , 6 : 53 – 58 .
- Alpert , P . 2006 . Constraints of tolerance: why are desiccation-tolerant organisms so small or rare? . J. Exp. Biol. , 209 : 1575 – 1584 .
- Bell , RA . 1993 . Cryptoendolithic algae of hot semiarid land and deserts . J. Phycol. , 29 : 133 – 139 .
- Belnap , J and Lange , OL . 2001 . Biological Soil Crusts: Structure, Function and Management , Berlin, , Germany : Springer .
- Bertsch , A . 1966 . CO2 Gaswechsel der Grünalge Apatococcus lobatus . Planta , 70 : 46 – 72 .
- Bischof , K , Hanelt , D , Tüg , H , Karsten , U , Brouwer , PEM and Wiencke , C . 1998 . Acclimation of brown algal photosynthesis to ultraviolet radiation in Arctic coastal waters (Spitsbergen, Norway) . Polar Biol. , 20 : 388 – 395 .
- Bischof , K , Peralta , G , Kraebs , G , Van de Poll , WH , Perez-Llorens , JL and Breeman , AM . 2002 . Effects of solar UV-B radiation on canopy structure of Ulva communities from southern Spain . J. Exp. Bot. , 379 : 2411 – 2421 .
- Borowitzka , LJ . 1986 . Osmoregulation in blue-green algae . Prog. Phycol. Res. , 4 : 243 – 256 .
- Brown , AD and Simpson , JR . 1972 . Water relations of sugar-tolerant yeasts: the role of intracellular polyols . J. Gen. Microbiol. , 72 : 589 – 591 .
- Brown , LM and Hellebust , JA . 1980 . The contribution of organic solutes to osmotic balance in some green and eustigmatophyte algae . J. Phycol. , 16 : 265 – 270 .
- BÜDEL, B. (2005). Microorganisms of biological crusts on soil surface. In Microorganisms in Soils: Roles in Genesis and Functions (Buscot, F. & Varma, A., editors). Soil Biology 3: 307–323
- Cardon , ZG , Gray , DW and Lewis , LA . 2008 . The green algal underground: evolutionary secrets of desert cells . Bio. Sci. , 58 : 114 – 122 .
- Darienko , T , Gustavs , L , Mudimu , O , Menendez , CR , Schumann , R , Karsten , U , Friedl , T and Pröschold , T . 2010 . Chloroidium, a common terrestrial coccoid green alga previously assigned to Chlorella (Trebouxiophyceae, Chlorophyta) . Eur. J. Phycol. , 45 : 1 – 17 .
- De Winder , B , Matthijs , HCP and Mur , LR . 1990 . The effect of dehydration and ion stress on carbon dioxide fixation in drought-tolerant phototrophic micro-organisms . FEMS Microbiol. Ecol. , 74 : 33 – 38 .
- Eggert , A , Haubner , N , Klausch , S , Karsten , U and Schumann , R . 2006 . Quantification of algal biofilms colonising building materials: chlorophyll a measured by PAM-fluorometry as a biomass parameter . Biofouling , 22 : 79 – 90 .
- Elster , J , Degma , P , Kovacik , L , Valentova , L , Sramkova , K and pereira , AB . 2008 . Freezing and desiccation injury resistance in the filamentous green alga Klebsormidium from the Antarctic, Arctic and Slovakia . Biologia , 63 : 839 – 47 .
- Ettl , H and Gärtner , G . 1995 . Syllabus der Boden-, Luft- und Flechtenalgen , Stuttgart, , Germany : Gustav Fischer Verlag .
- Evans , RD and Johansen , JR . 1999 . Microbiotic crusts and ecosystem processes . Crit. Rev. Plant Sci. , 18 : 183 – 225 .
- Feige , G and Kremer , BP . 1980 . Unusual carbohydrate pattern in Trentepohlia species . Phytochem. , 19 : 1844 – 1845 .
- Flechtner , VR . 2007 . “ North American desert microbiotic soil crust communities: diversity despite challenge ” . In Algae and Cyanobacteria in Extreme Environments , Edited by: Seckbach , J . 537 – 551 . Berlin, , Germany : Springer .
- Friedl , T . 1997 . The evolution of the green algae . Plant Syst. Evol. , 11 : 87 – 101 .
- Gaylarde , CC and Morton , LHG . 1999 . Deteriogenic biofilms on buildings and their control: A review . Biofouling , 14 : 59 – 74 .
- Gray , DW , Lewis , LA and Cardon , ZG . 2007 . Photosynthetic recovery following desiccation of desert green algae (Chlorophyta) and their aquatic relatives . Plant Cell Environ. , 30 : 1240 – 1255 .
- GUSTAVS, L., EGGERT, A., MICHALIK, D. & Karsten, U. (2009a). Physiological and biochemical responses of aeroterrestrial green algae (Trebouxiophyceae) to osmotic and matric stress. Protoplasma, DOI 10.1007/s00709-009-0060-9
- Gustavs , L , Schumann , R , Eggert , A and Karsten , U . 2009b . In vivo growth fluorometry: accuracy and limits of microalgal growth rate measurements in ecophysiological investigations . Aquat. Microb. Ecol. , 55 : 95 – 104 .
- Hanelt , D , Wiencke , C , Karsten , U and Nultsch , W . 1997 . Photoinhibition and recovery after high light stress in different developmental and life-history stages of Laminaria saccharina (Phaeophyta) . J. Phycol. , 33 : 387 – 395 .
- Häubner , N , Schumann , R and Karsten , U . 2006 . Aeroterrestrial algae growing on facades – response to temperature and water stress . Microb. Ecol. , 51 : 285 – 293 .
- Holzinger , A , Karsten , U , Lütz , C and Wiencke , C . 2006 . Ultrastructure and photosynthesis in the supralittoral green macroalga Prasiola crispa (Lightfoot) Kützing from Spitsbergen (Norway) under UV exposure . Phycologia , 45 : 168 – 177 .
- JOHANSEN, J.R. & SHUBERT, L.E. (2001). Algae in soils. In Algae and Extreme Environments (Elster, J., Seckbach, J., Vincent, W.F. & Lhotsky, O. editors). Nova Hedwigia Beiheft, 123: 297–306
- Karsten , U , Barrow , KD , Nixdorf , O and King , RJ . 1996 . The compability of unusual organic osmolytes from mangrove red algae with enzyme activity . Austr. J. Plant Physiol. , 23 : 577 – 582 .
- Karsten , U , Friedl , T , Schumann , R , Hoyer , K and Lembcke , S . 2005 . Mycosporine-like amino acids (MAAs) and phylogenies in green algae: Prasiola and its relatives from the Trebouxiophyceae (Chlorophyta) . J. Phycol. , 41 : 557 – 566 .
- Karsten , U , Lembcke , S and Schumann , R . 2007a . The effects of ultraviolet radiation on photosynthetic performance, growth and sunscreen compounds in aeroterrestrial biofilm algae isolated from building facades . Planta , 225 : 991 – 1000 .
- Karsten , U , Schumann , R and Mostaert , A . 2007b . “ Aeroterrestrial algae growing on man-made surfaces – what are the secrets of their ecological success? ” . In Algae and Cyanobacteria in Extreme Environments , Edited by: Seckbach , J . 583 – 597 . Berlin, , Germany : Springer .
- Karsten , U , Thomas , DN , Weykam , G , Daniel , C and Kirst , GO . 1991a . A simple and rapid method for extraction and separation of low molecular weight carbohydrates from marine macroalgae using high performance liquid chromatography . Plant Physiol. Biochem. , 29 : 373 – 378 .
- Karsten , U , Wiencke , C and Kirst , GO . 1991b . The effect of salinity changes upon physiology of eulittoral green macroalgae from Antarctica and Southern Chile. II. Inorganic ions and organic compounds . J. Exp. Bot. , 42 : 1533 – 1539 .
- Kirst , GO . 1990 . Salinity tolerance of eukaryotic algae . Annu. Rev. Plant Physiol. Plant Mol. Biol. , 41 : 21 – 53 .
- Leukart , P and Hanelt , D . 1995 . Light requirements for photosynthesis and growth in several macroalgae from a small soft-water stream in the Spessart Mountains, Germany . Phycologia , 34 : 528 – 532 .
- Lewis , LA . 2007 . “ Chlorophyta on land: independent lineages of green eukaryotes from arid lands ” . In Algae and Cyanobacteria in Extreme Environments , Edited by: Seckbach , J . 571 – 582 . Berlin, , Germany : Springer .
- Menendez , JL , Rindi , F , Rico , JM and Guiry , MD . 2006 . The use of CHAID classification trees as an effective descriptor of the distribution of Rosenvingiella radicans (Prasiolales, Chlorophyta) in urban environments . Cryptogamie, Algol. , 27 : 153 – 164 .
- Nagao , M , Matsui , K and Uemura , M . 2008 . Klebsormidium flaccidum, a charophycean green alga, exhibits cold acclimation that is closely associated with compatible solute accumulation and ultrastructural changes . Plant Cell Environ. , 31 : 872 – 885 .
- Oren , A . 2007 . “ Diversity of organic osmotic compounds and osmotic adaptation in cyanobacteria and algae ” . In Algae and Cyanobacteria in Extreme Environments , Edited by: Seckbach , J . 641 – 655 . Berlin, , Germany : Springer .
- Ortega-Calvo , JJ , Arino , X , Hernandez-marine , M and Saiz-Jimenez , C . 1995 . Factors affecting the weathering and colonisation of monuments by phototrophic microorganisms . Sci. Tot. Environ. , 167 : 329 – 341 .
- Ortega-Calvo , JJ , Hernandez-Marine , M and Saiz-Jimenez , C . 1991 . Biodeterioration of building materials by cyanobacteria and algae . Int. Biodeter. , 28 : 165 – 185 .
- Porra , RJ , Thompson , WA and Kriedmann , PE . 1989 . Determination of accurate extinction coefficients and simultaneous equations for assaying chlorophylls a and b extracted with four different solvents: verification of the concentration of chlorophyll standards by atomic absorption spectroscopy . Biochim. Biophys. Acta , 975 : 384 – 394 .
- Potts , M . 1999 . Mechanisms of desiccation tolerance in cyanobacteria . Eur. J. Phycol. , 34 : 319 – 328 .
- REMIAS, D., ALBERT, A. & LÜTZ, C. (2010). Effects of realistically, simulated, elevated UV irradiation on photosynthesis and pigment composition of the alpine snow alga Chlamydomonas nivalis and the Arctic soil alga Tetracystis sp. (Chlorophyceae). Photosynthetica, 48: 269–277
- Rindi , F . 2007 . “ Diversity, distribution and ecology of green algae and cyanobacteria in urban habitats ” . In Algae and Cyanobacteria in Extreme Environments , Edited by: Seckbach , J . 621 – 638 . Berlin, , Germany : Springer .
- Rindi , F and Guiry , MD . 2004 . Composition and spatial variability of terrestrial algal assemblages occurring at the bases of urban walls in Europe . Phycologia , 43 : 225 – 235 .
- Rindi , F , Guiry , MD and Lopez-Bautista , JM . 2008 . Distribution, morphology, and phylogeny of Klebsormidium (Klebsormidiales, Charophyceae) in urban environments in Europe . J. Phycol. , 44 : 1529 – 1540 .
- Rindi , F , Mcivor , L and Guiry , MD . 2004 . The Prasiolales (Chlorophyta) of Atlantic Europe: an assessment based on morphological, molecular, and ecological data, including the characterization of Rosenvingiella radicans (Kützing) comb. nov . J. Phycol. , 40 : 977 – 997 .
- Setter , TL and Greenway , H . 1979 . Growth and osmoregulation of Chlorella emersonii in NaCl and neutral osmotica . Aust. J. Plant Physiol. , 6 : 47 – 60 .
- Silva , PC , Mattox , KR and Blackwell , WH . 1972 . The generic name Hormidium as applied to green algae . Taxon , 21 : 639 – 645 .
- Starr , RC and Zeikus , JA . 1993 . UTEX – the culture collection of algae at the University of Texas at Austin 1993 list of cultures . J. Phycol. , 29 : 1 – 106 .
- Tan , CK , Lee , YK and Ho , KK . 1993 . Effect of light intensity and ammonium-N on carotenogenesis of Trentepohlia odorata and Dunaliella bardawil . J. Appl. Phycol. , 5 : 547 – 549 .
- Tompkins , J , Deville , MM , Day , JG and Turner , MF . 1995 . Culture collection of algae and protozoa. Catalogue of strains , Ambleside, , UK : The Culture Collection of Algae and Protozoa, Institute of Freshwater Ecology .
- Webb , WL , Newton , M and Starr , D . 1974 . Carbon dioxide exchange of Alnus rubra: a mathematical model . Oecologia , 17 : 281 – 291 .
- Wright , DJ , Smith , SC , Joardar , V , Scherer , S , Jervis , J , Warren , A , Helm , RF and Potts , M . 2005 . UV irradiation and desiccation modulate the three-dimensional extracellular matrix of Nostoc commune (Cyanobacteria) . J. Biol. Chem. , 280 : 40271 – 40281 .
- Yancey , PH . 2005 . Organic osmolytes as compatible, metabolic and counteracting cytoprotectants in high osmolarity and other stresses . J. Exp. Biol. , 208 : 2819 – 2830 .